Coffee is a tropical woody crop belonging to the Rubiaceae family that grows in approximately 80 tropical countries, and it is native to northern Ethiopia. Arabic coffee (Coffea arabica L.) and robusta coffee (Coffea canephora Pierre ex Froehner) represent one of the most commercially important agricultural products with high genetic value in the world (Caporaso et al. 2018).
It is estimated that around 25 million farming families around the world produce coffee, with a majority of small producers and families whose livelihoods depend deeply on this crop (DaMatta et al. 2019, Semedo et al. 2021). Coffee world production was estimated between 9.6 and 10.3 million Ton year-1 (ICO 2020). However, for 2017-2018 a reduction of 3.6 % in production was reported (ICO 2020).
The genus Coffea is diploid (2n = 22), except for C. arabica, which is the only tetraploid species (2n = 4x = 44) and is autogamous, i.e. self-compatible (Romero et al. 2010), therefore, a seed is a genetic copy of the mother plant. Coffea arabica species are made up of a set of varieties and hybrids that have differentiated agronomic and productive characteristics (ANACAFÉ 2019). The relationships between the main pure Arabic varieties and intervarietal crosses are shown in Figure 1, highlighting that the Typica and Bourbon varieties have originated the other varieties by crosses or mutations. From these different genotypes, varieties Caturra, Mundo Novo, Catuaí, Pacas, Pache and Villa Sarchi have been developed (Várzea et al. 2008, ANACAFÉ 2019).
Genetic improvement in coffee crop has been aimed at increasing productivity (Duicela 2017, 2021) and for greater resistance to the main pests and diseases such as coffee rust, coffee borer, nematodes and anthracnose (Eskes 1989). Coffee breeding programs have considered some interspecific hybrids such as the Timor Hybrid (TH), result of a natural interspecific cross between C. arabica × C. canephora (Figure 1), with resistance to coffee rust (Julca-Otiniano et al. 2018), and/or artificial hybrids such as Icatú and Arabusta, Sarchimor (Villa Sarchi × TH), Catimor (Caturra × TH) and Cavimor (Catuaí × Catimor) (Várzea et al. 2008, ANACAFÉ 2019).
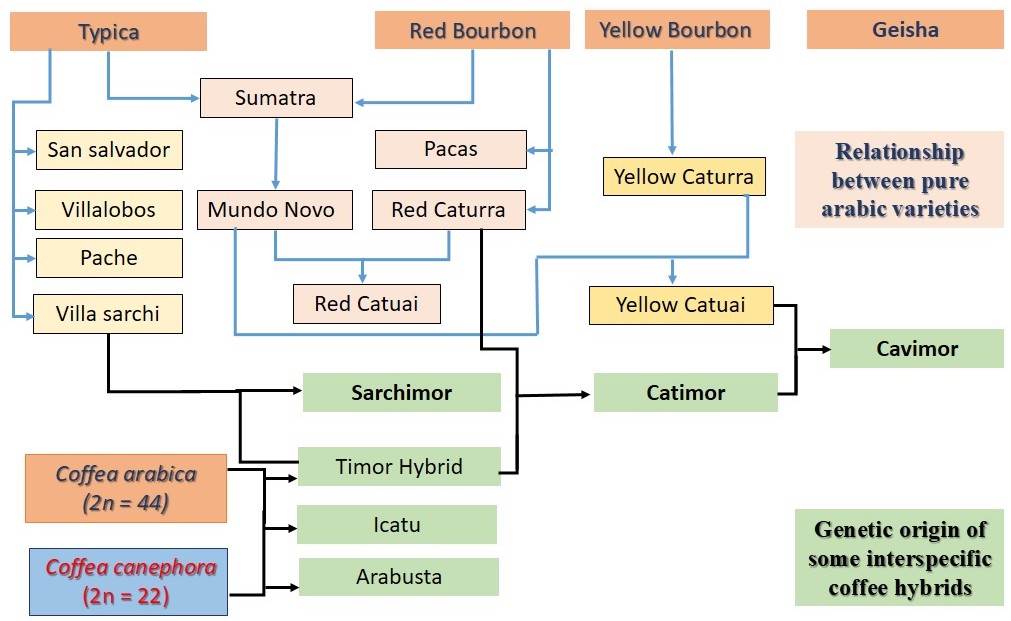
Figure 1 Relationship between pure Arabic varieties (blue lines) and some interspecific hybrids in coffee (black lines).
In Ecuador, a wide range of pure C. arabica varieties and hybrids derived from TH and Icatú are cultivated, highlighting the varieties Sarchimor C-1669, Sarchimor C-4260 and Catimor ECU selected for their high productivity, wide adaptation to different climates and resistance to coffee rust (World Coffee Research 2018). In Nicaragua, the hybrid Mundo Maya H16 (Sarchimor T5296 × ET01), produces an average of 599 g plant-1 while Caturra only produces 370 g plant-1 (Marie et al. 2020), indicating the expression of the production potential of the Sarchimor hybrid.
Ecophysiological information in coffee in Brazil has been reported from various authors (Carelli et al. 2006, Cavatte et al. 2012, DaMatta & Rena 2001, DaMatta 2004a, DaMatta & Ramalho 2006, DaMatta et al. 2016, Semedo et al. 2021, Martins et al. 2016, Rodríguez-López et al. 2014) while only few studies have been done in Ecuador (Tezara et al. 2018, 2020). Averages net photosynthetic rate (A) of 8 μmol m-2 s-1 and stomatal conductance (gs) of 148 μmol m-2 s-1 have been reported for arabic and Conilón coffee in Brazil (DaMatta et al. 2007); while C. arabica genotypes in Ecuador, showed highest values of A (10-15 μmol m-2 s-1) and gs (198-412 μmol m-2 s-1); however, still lack of physiological information (gas exchanges and water status) of Ecuadorian coffee (Tezara et al. 2018, 2020).
A major component of differential adaptation to drought among arabic coffee genotypes may be governed by rates of water use or efficiency of extraction of soil water (DaMatta et al. 2003, Pinheiro 2004). Therefore physiological, and morphological characteristics, such as, gs, root depth and water use efficiency (WUE), should be recommended as potential traits for selecting coffee genotypes with higher performance under drought conditions (DaMatta 2004b).
Although there is information on production and tolerance to diseases in many coffee genotypes, genetic improvement programs in Ecuador lack physiological data (Tezara 2017, Tezara et al. 2018, Tezara Fernández 2020), especially on photosynthetic traits and their response to drought. This information is essential to understand the physiological responses of new coffee genotypes to different environmental variables in the different agroecological regions of Ecuador.
Therefore, in order to gain knowledge on physiological traits and productive performance of 21 C. arabica genotypes in Manabí, Ecuador, we evaluated leaf water status, gas exchange variables during rainy (RS) and drought season (DS). Besides, long-term coffee production (3 years) of adult trees was assessed. These data can then provide insights into whether the high genetic variability among C. arabica genotypes explains photosynthetic and production differences expected, and if the physiological response to DS is different among genotypes. We hypothesized that during DS, 1) differential responses would occur among genotypes due to different physiological variation, and 2) WUE would increase differently among genotypes.
Materials and methods
Study site and climatic conditions. The study was carried out at the “La Esperanza” Experimental Farm located in Solano, Pichincha canton, Manabí province, Ecuador, at 01° 03´ 01” S; 79° 56´ 39” W at 220 m asl. The study area has a mean annual precipitation of 1,300 mm, mean air temperature of 24 °C, relative humidity (RH) of 86 % and a heliophany of 779 sun hours yr-1 were obtained from station of the National Institute of Meteorology and Hydrology (http://186.42.174.236/InamhiEmas/).
Plant material. In this farm, 21 genotypes of C. arabica (Table 1) four years old were grown under unshaded conditions and their production has been evaluated since 20 March 2014. In an area of 756 m2, 12 plants of each of the 21 genotypes of C. arabica were planted at a distance of 1.5 × 2 m, for a total of 252 plants, i.e., a density 3,333 plants per Ha.
Table 1 Name, code, genetic origin and reaction to coffee rust of 21 C. arabica genotypes in the Pichincha canton, Manabí province, Ecuador.
Name | Code | Genetic origin | Reaction to coffee rust |
---|---|---|---|
Catimor 8666 (4-3) | 8666 | CATIE's select line | R |
Catimor 8664 (2-3)* | 8664 | CATIE's select line | R |
Catimor UFV 5607* | U5607 | UFV select line | R |
Catuaí UFV 2144 | U2144 | Mundo novo × Caturra | MS |
Catimor CIFC P1 | CIFCP1 | F5 seed mix Caturra × H. Timor of the progeny CIFC 7960 | R |
Cavimor H 765 | H765 | Hybrid Catuaí × Catimor CIFC | R |
Acawá | Aca | Mundo novo IAC 388-17 × Sarchimor IAC 1668 | MR |
Catucaí 2 SL | 2SL | Hybrid Catuaí × Icatú | MS |
Sarchimor C-4260 | C4260 | Hybrid Villa Sarchi × H. Timor | R |
Catimor CIFC P2 | CIFCP2 | F5 seed mix F5 Caturra × H. Timor of the progeny 7961 | R |
Cavimor H 773 | H773 | Hybrid Catuaí × Catimor CIFC | R |
Cavimor 772 | 772 | Hybrid Catuaí × Catimor CIFC | R |
Catucaí 785-15 | 785-15 | Hybrid Catuaí × Icatú | MR |
Caturra rojo Ecu | CatEcu | Bourbon Mutation Lines T-2308, T-2542 and C-818 | S |
Catimor CIFC P3 | CIFCP3 | F5 seed mix Caturra × H. Timor of the progeny CIFC 7962 | R |
Geisha Ecu | Geis | Pure Arabic from Ethiopia T-2722 | MS |
Catimor UFV 5608 | U5608 | UFV select line | R |
Arará | Ara | Obatá amarillo × Catuaí Crossing (Obatá: Sarchimor 1660-20) | MR |
Caturra amarillo 3386 | 3386 | Bourbon mutation | S |
Mundo novo | Mun | Sumatra × Bourbon rojo | S |
Cavimor H-789 | H789 | Hybrid Catuaí × Catimor CIFC | R |
* Genotypes selected for productivity and resistance to rust.
R: resistant, S: susceptible, M: Moderate
Edaphic and climatic variables of the study site. A chemical analysis of the soil was carried out prior to the establishment of the coffee plantation in the laboratory of soils, water and plant tissues of the Pichilingue Station of the Instituto Nacional de Investigaciones Agropecuarias (INIAP). The soil was moderately acid (pH 5.5), and the qualitative contents of different nutrients were: low for N, Mn, S, and B, high for P2O5, K2O, Ca, Mg, Zn, and Fe, and medium for Cu.
Precipitation data from 2016-2018 were obtained from a pluviometric station (INAMHI http://186.42.174.236/InamhiEmas/) in the Solano precinct, in Pichincha canton, Manabí province, Ecuador.
Agronomic management. Based on the results from the chemical analysis of the soil, an application of CaSO4 was made at sowing, at a rate of 200 g plant-1. The fertilization plan for coffee plantations in production was 2 applications of 66 g plant-1 of urea 46 %, one in January and the other in March; a single application of 60 g of di-ammonium phosphate (DAP) 18-46-00 in January; two applications of 30 g of KCl 60 % in March in 2014. In the second year of establishment of the coffee plantation, 10 % boronat was applied at a rate of 12.5 g plant-1. The management of the coffee plantation included three weeding per year and pruning during the DS.
Leaf nutrient analysis. The leaf nutrient analysis carried out on a sample of coffee trees, two years after establishment, showed the following nutritional status: N 2.2 % (deficient), P 0.17 % (adequate), K 1.18 % (adequate), Ca 1.15 % (adequate), Mg 0.19 % (poor), S 0.18 % (adequate), Zn 10 ppm (poor), Cu 4 ppm (poor), Fe 294 ppm (excessive), Mn147 ppm (suitable) and B 47 ppm (suitable).
Water status. Samples of soil and leaf were collected at 0700 h to determine soil water content (SWC) and leaf relative water content (RWC). The SWC was determined in soil samples taken at 15-cm depth, where fresh mass (FM) was determined, then dried at 70 °C for 48 h, and weighed to obtain the dry mass (DM) (Bilskie 2001). The soil water content was determined as:
Leaf RWC collected at 0700 h and floated on distilled water in the dark at 4 °C for 1 h in order to obtain values of turgid mass (TM). The RWC was determined in leaves (n = 5) i.e., five leaf taken from different plants, followed Turner (1981):
Gas exchange measurements. Net photosynthetic rate (A, μmol m-2 s-1), gs (μmol m-2 s-1), intercellular CO2 concentration (Ci, μmol mol-1), transpiration rate (E, μmol m-2 s-1) were made in intact leaves in five individuals of each genotype (n = 5 per genotype) with a portable infrared gas analyzer (CIRAS-II, PP Systems Inc., Amesbury, MA) connected to a leaf chamber PLC (B). All measurements were made on fully expanded and healthy adult leaves (third leaf from the apex), under the following conditions: CO2 concentration of 400 ± 10 μmol mol-1, leaf chamber temperature of 30.0 ± 1 °C, a leaf-to-air vapor pressure deficit (Δ W ) of 1.3 ± 0.03 (RS) and 1.7 ± 0.1 KPa (DS) and photosynthetic photon flux density (PFD) of 1,200 ± 20 μmol m-2 s-1 (light provided by a LED Based Light Unit from the same manufacturer). To ensure that A was not limited by light and to compare the maximum A at a relatively high PFD, was use supra-saturating PFD. Instantaneous water use efficiency was estimated as WUE = A/E. Measurements were randomly made between 0800 and 1,200 h in all genotypes during three consecutive days from March 31 to April 02 (RS) and June 29 to July 1, 2017 (DS).
Production data recording. The production of the 252 coffee trees of the 21 C. arabica genotypes was evaluated. The harvest of the physiologically mature and healthy fruits was carried out approximately every 15-22 days, when verifying the prevalence of mature fruits, whether red or yellow. The fruits obtained for each harvest, for each coffee tree, were weighed using a technical scale balance. Production of golden coffee (is essentially an ultralight coffee) from 2016 to 2018 was expressed as g plant-1. The term of "gold coffee" is the term that the coffee bean receives once after the different coverings have been separated through the hulling process. To have the annual coffee harvest, partial harvests were added. The potential coffee production was estimated based on the number of plants ha-1 and the use of the conversion coefficient of 5.0 to 1.0 (500 grams of cherry (fresh) coffee allows to obtain 100 grams of golden (dry) coffee, at 10 % of humidity). Production potential was expressed as Kg of golden coffee ha-1.
Statistical analysis. A completely randomized statistical design was used. Results are presented as means ± standard error (SE). Physiological measures were randomly sampled from 5 plants of each genotype of C. arabica (n = 5) in the 21 different genotypes. One- and two-way ANOVAs were performed using the statistical package STATISTICA v. 10 (StatSoft Inc., Tulsa, OK, USA) to evaluate whether the different physiological variables studied differ among genotypes and between seasons. All linear regressions and t tests were tested for significance at P ≤ 0.05. All plots were made using SigmaPlot 11 (Systat Software, San Jose, CA, USA).
Results
Precipitation. The RS in the study site lasts six months, from January to June, and the rest of the year corresponds to DS (Figure 2). It was evidenced that between 2016 and 2018, there was a high variation in the accumulated precipitation per season (2016, 1,533 mm; 2017, 1,838 mm and in 2018, 1,241 mm). However, the daily distribution, expressed in the number of days with and without rain (Figure 2), was key to knowing the true duration of the RS and interpreting its effect on yield. In 2016, in May and June there were only 7 and 4 days with rain; while in 2017, May and June had 20 and 10 rainy days, respectively, and in 2018, May and June had 11 and 2 rainy days, respectively. This means that the RS was c. 5 months/year.

Figure 2 Precipitation cycles during three years from 2016 to 2018 (closed circles), and days with rain (open bars) and without rain (greys bars) during the rainy season in Solano, Pichincha canton, Manabí. The blue arrows represent the days when physiological measurements were done.
Water status. Soil water content (SWC) decreased from 25.8 ± 0.4 in the RS to 11.2 ± 0.7 % during DS, i.e., drought cause a significant reduction of 56.6 % in SWC (P = 0.0000, ANOVA F-test statistic (F) = 255.1 degree of freedom (df) = 1). Significant differences in RWC occurred among coffee genotypes (P = 0.016, F = 1.98, df = 20), between seasons (P = 0.000, F = 265.69, df = 1) and interaction genotypes × seasons (P = 0.0046, F = 2.29, df = 20; (Figure 3). Drought cause a decrease of 15.8 % in RWC average in all coffee genotypes. In all genotypes, the highest RWC were observed in RS, the genotypes that showed the highest RWC were U2144, 2SL, followed by CIFCP2, Mun, H765 and CIFCP1, while the lowest values were observed in genotypes H765, C4260, U5608 during drought (Figure 3).
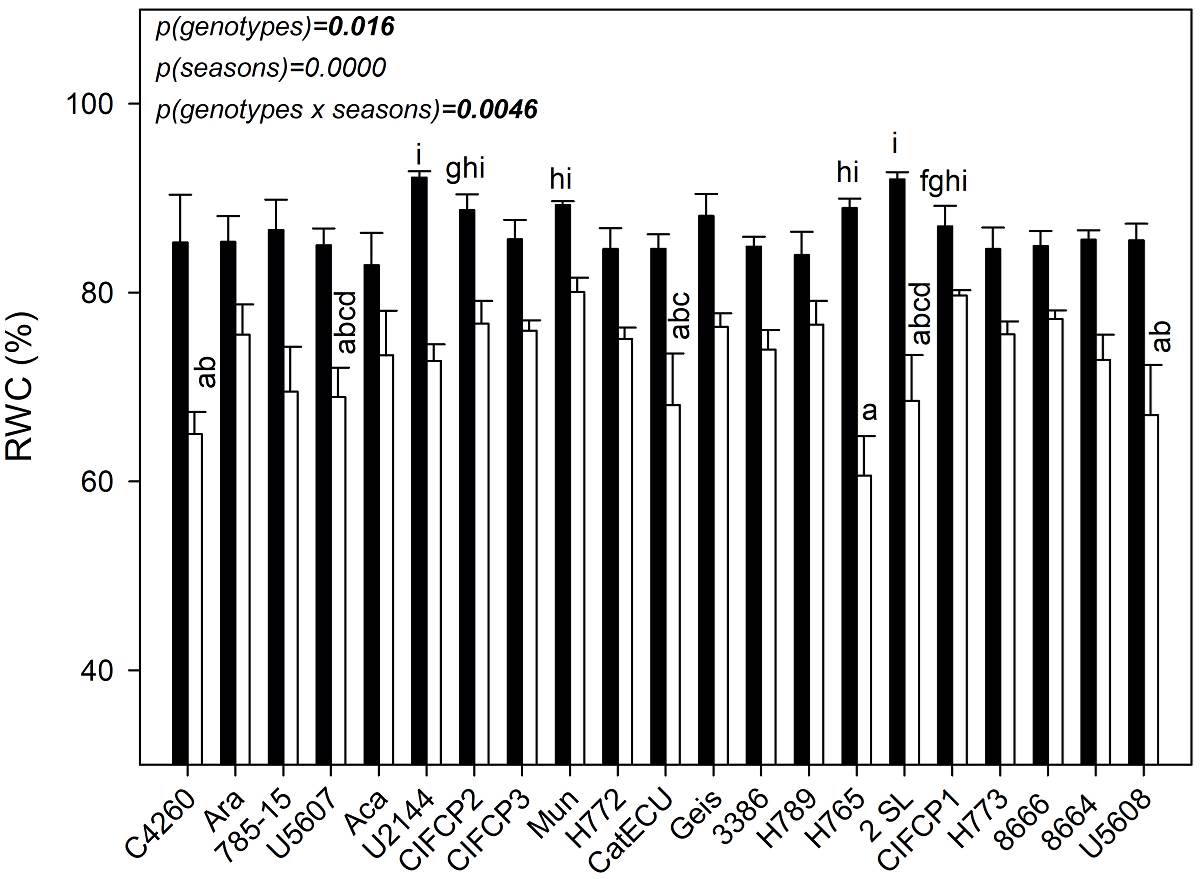
Figure 3 Values of relative water content of 21 C. arabica genotypes (x-axis), in adult trees of the La Esperanza experimental farm, Pichincha canton, Manabí province, Ecuador, in the rainy season (black bars) and at the onset of DS (white bars). Each bar shows the average of 5 different trees ± SE (n = 5). Different letters indicate differences between genotypes and season (P < 0.05), shown only in the maximum and minimum values. Significant differences among genotypes, between seasons and interaction were shown.
Gas exchanges. There was a significant interaction of genotype × season (P < 0.05) in all gas exchange parameters evaluated (Figure 4). During the RS, A varied between 7.6 and 14.7 µmol m-2 s-1 among genotypes (P < 0.0001, F = 4.6, df = 20); the highest A were observed in genotypes 8666, 8664, U5608; whereas A was between 4.2 and 13.1 μmol m-2 s-1 during DS, the lowest values were found in genotypes CIFCP3 and Mun. In most genotypes, drought caused a reduction of 30 % in mean A (P = 0.0001, F = 104.7, df = 1). However, in CIFC-P1, U5607, and 3386, there was no seasonal change in A (Figure 4A). Values of E varied significantly between 2.2 and 5.9 μmol m-2 s-1 in all genotypes (P = 0.0002, F = 2.93, df = 20; Figure 4B). Average E decreased by 33 % with drought (P < 0.0001, F = 199.9, df = 1). Values of gs showed significant differences among genotypes (P = 0.00034, F = 2.33, df = 20; Figure 4C). The average gs was reduced by 44 % from 415 ± 14 to 230 ± 16 μmol m-2 s-1 between seasons. Significant differences were found in WUE among genotypes (P < 0.0001, F= 7.10, df = 20; Figure 4D). Values of WUE varied from 1.5 to 3.3 μmol mol-1 (RS) and from 1.2 to 3.4 μmol mol-1 (DS), without differences between seasons (P = 0.83, F = 0.045, df = 1). Genotype × season interaction on WUE was significant (P = 0.017, F = 1.94, df = 20). The genotypes U5608, 8664, 8666 and H-773, showed the highest WUE, while the lowest were found in C4260, Ara, 785-15 and Mun. Intercellular CO2 concentration (Ci) varied between 286 and 358 µmol mol-1, among genotypes (P < 0.0001, F= 5.06, df = 20), without differences between seasons (P = 0.22, F= 1.49, df = 1). Genotype × season interaction on Ci was significant (P = 0.000027, F = 3.45, df = 20).
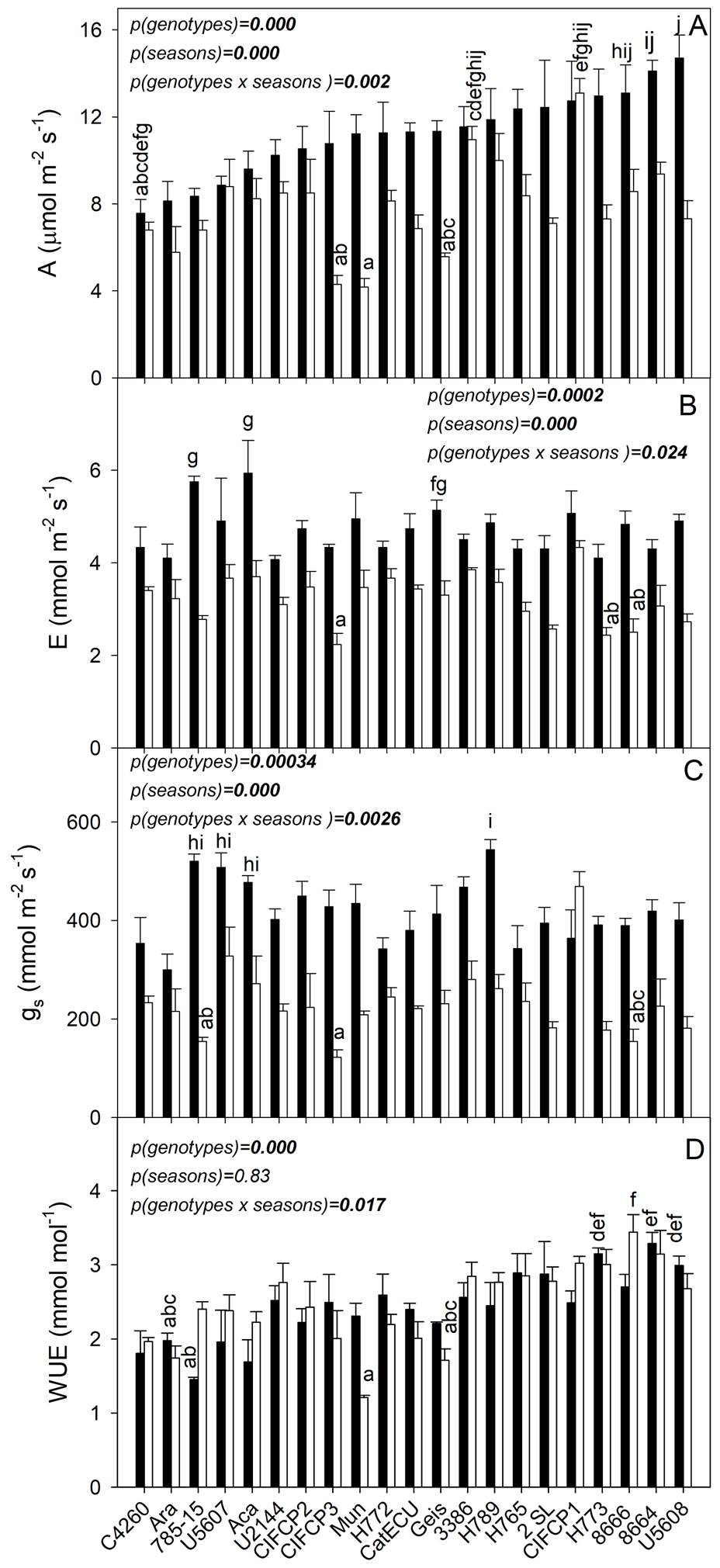
Figure 4 Gas exchange of 21 C. arabica genotypes (x-axis), A. photosynthetic rate, B. transpiration rate, C. stomatal conductance, D. water use efficiency in adult trees of the La Esperanza experimental farm, Pichincha canton, Manabí province, Ecuador, in the rainy season (black bars) and at the onset of DS (white bars). Each bar shows the average of 5 different trees ± SE (n = 5). Different letters indicate differences between genotypes and season (P < 0.05), shown only in the maximum and minimum values. Significant differences among genotypes, between seasons and interaction were shown.
A significant linear relationship was found between A and gs (r 2 = 0.53; P < 0.05; Figure 5). Values of A and gs showed a significant negative linear relationship with Δ W in the 21 genotypes of C. arabica studied, (r 2 = 0.41 and 0.74, respectively; P < 0.05); higher Δ W values caused a reduction in A due to lower gs (Figure 6).

Figure 5 Relationship between photosynthetic rate and stomatal conductance in 21 C. arabica genotypes evaluated in the rainy season (black circles) and the beginning of DS (white circles). Each symbol represents the mean ± standard error (n =5). The linear regression equation and the coefficient of determination are shown, significant at P < 0.05.

Figure 6 A. Relationship between the photosynthetic rate and the leaf-air water vapor gradient and B. Relationship between the stomatal conductance and the leaf-air water vapor gradient of 21 C. arabica coffee genotypes evaluated in the rainy season (black circles) and the onset of DS (white circles). Each symbol represents the mean ± standard error (n = 5). The linear regression equation and the coefficient of determination are shown, significant at P < 0.05.
Coffea potential production. Coffea arabica genotypes were classified in six groups, according to their gold coffee production (g plant-1) and percentage of empty beans (%): I, 500 and 17 %; II, 447-463 and 6-7 %; III, 387- 440 and 19-34 %; IV, 365 and 6 % ; V, 234-355 and 7-26 %; and VI, 134-206 and 12-23 %, respectively (Table 2). Significant differences were found in coffee production among genotypes (P < 0.0075, F = 3.27 df = 20). Catimor 8666, 8664, U5607, and U2144 genotypes showed the highest production and potential production of coffee (440-500 g plant-1; 1320-1501 Kg ha-1); while Ara, 3386, Mun and H789 genotypes had the lowest yields (134-55 g plant-1; 401-465, Kg ha-1) (Table 2).
Table 2 Production of 21 Coffea arabica genotypes was evaluated in twelve adult trees (n = 12) during three consecutive years in the canton of Pichincha, Manabí province. Production of golden coffee from 2016 to 2018 was expressed as grams per plant; shows mean ± standard error of the 3-year average, production potential and percentage of vain beans of all genotypes studied. Different letters indicate significant differences between clones (P < 0.05).
Code | Gold coffee production (g plant-1) | Production potential (kg ha-1) |
Vain grain (%) |
*Group | |||
---|---|---|---|---|---|---|---|
2016 | 2017 | 2018 | Mean ± SE | ||||
8666 | 382 | 636 | 483 | 500 ± 74 f | 1,501 | 17 | I |
8664 | 254 | 636 | 498 | 463 ± 112 ef | 1,388 | 7 | II |
U5607 | 368 | 454 | 518 | 447 ± 43 def | 1,340 | 6 | |
U2144 | 307 | 545 | 468 | 440 ± 70 cdf | 1,320 | 19 | III |
CIFC P1 | 422 | 302 | 585 | 436 ± 82 cdf | 1,309 | 34 | |
H 765 | 523 | 182 | 522 | 409 ±114 bcdef | 1,227 | 21 | |
Aca | 190 | 545 | 470 | 402 ±108 bcdef | 1,205 | 23 | |
2 SL | 553 | 182 | 427 | 387 ± 109 bcdef | 1,162 | 20 | |
C-4260 | 212 | 454 | 430 | 365 ± 77 bcdef | 1,096 | 5 | IV |
CIFC P2 | 497 | 182 | 415 | 365 ± 94 bcdef | 1,094 | 6 | |
H 773 | 162 | 354 | 458 | 325 ± 87 abcdef | 974 | 21 | V |
772 | 229 | 272 | 350 | 284 ± 35 abcde | 851 | 22 | |
785-15 | 277 | 182 | 301 | 253 ± 36 abcd | 760 | 9 | |
CatEcu | 331 | 91 | 325 | 249 ± 79 abcd | 747 | 16 | |
CIFC P3 | 257 | 182 | 294 | 244 ± 33 abcd | 733 | 7 | |
Geis | 167 | 272 | 262 | 234 ± 34 abc | 701 | 26 | |
U5608 | 239 | 91 | 289 | 206 ± 60 a | 619 | 12 | VI |
Ara | 164 | 91 | 210 | 155 ± 35 a | 465 | 23 | |
3386 | 133 | 91 | 199 | 141 ± 31 a | 423 | 14 | |
Mun | 210 | 91 | 115 | 139 ± 36 a | 416 | 13 | |
H-789 | 55 | 182 | 164 | 134 ± 40 a | 401 | 23 |
Discussion
There were significant differences in waters status and gas exchange among C. arabica genotypes and there was an interaction effect of genotype × season, supporting our hypothesis 1. In most of the genotypes, DS caused a significant reduction in RWC, A, E and gs, indicating that coffee was sensitive to drought. Furthermore, significant differences in C. arabica production were found among genotypes. The genotypes Catimor 8664 and Catimor 8666 showed the highest A, WUE, and yield, and a low percentage of vain grains, suggesting that those genotypes could be selected for cultivation in the Pichincha canton, Manabí province, Ecuador. The varied genetic origin of the 21 C. arabica genotypes studied may explain the significant differences in RWC, gas exchange and production found. Also, the interaction effect between genotype and season on A, suggests a differential response of each genotype to DS. Genetic differences in the coffee genotypes studied can be expressed in different leaf phenotypes such as leaf area, specific leaf area; also showed differences in transpiration rate; thus all these traits could affect and explain the differences observed in RWC.
Precipitation data between 2016 and 2018 in the study location showed high variation in the total accumulated precipitation by season, without any relationship with coffee yield. We considered that the daily distribution, expressed in the number of days with and without rain, would be the key parameter to truly know the duration of RS and DS and interpreting the effect on gas exchange and production in C. arabica genotypes, as was recently reported in C. canephora (Duicela 2021).
The lower precipitation during the DS, which halved SWC, caused a reduction of RWC in all coffee genotypes under study, indicated that coffee genotypes were sensitive to drought. Leaves began water and turgor loss when low water availability during DS. However, coffee maintains a relative high RWC under dehydrating conditions, being considered a water saving rather than a dehydrating tolerant species (DaMatta et al. 1993). This may be attributed to an efficient stomatal control on transpiration (Pinheiro 2004), and/or low cell-wall elasticity (DaMatta et al. 1993, 2003, DaMatta 2004b, Pinheiro 2004). Thus, it appears that under drought the maintenance of a high RWC is more important than osmotic adjustment per se in conferring drought tolerance to the coffee plant (DaMatta et al. 1993, DaMatta 2004b).
Catimor 8664 and Catimor 8666 genotypes showed the best physiological performance (high A, and WUE) during RS and both genotypes showed high A and WUE during DS; in eleven coffee genotypes DS caused a marked decrease in gas exchanges parameters. We found differences in gas exchanges parameters among all genotypes in the DS, which suggests differences in tolerance to drought in Arabic coffee genotypes. The results do not support our hypothesis 2, i.e., that WUE would increase differently due to differential physiological variation, since in most of coffee genotypes WUE was not affected during DS; only in three coffee genotypes WUE increased. Most C. arabica genotypes showed optimization of water use, this may be due that photosynthesis was co-limited, i.e., stomatal closure and metabolic factors, changes coordinate way in order to remain constant WUE; supported by the fact that Ci did not showed differences between seasons.
Values of A and gs during RS (12-4 μmol m-2 s-1 and 230-415 μmol m-2 s-1, respectively) found in this study were similar to those reported in ten C. arabica genotypes evaluated in Esmeraldas, Ecuador (Tezara et al. 2018) and higher than those previously reported in C. arabica in Brazil (DaMatta et al. 2007, Semedo et al. 2021). In this study, higher A and gs may be due to microclimate differences between locations and/or an intrinsic higher photosynthetic capacity in the genotypes studied. The study area is a transition zone, between the dry tropical and the subtropical, with climatic variations due to the effect of the confluence of the cold Humboldt and warm El Niño currents that collide in front of Manabí province, causing a special microclimatic variation (Duicela et al. 2003). Besides, the weather conditions at the coast of Ecuador, are characterized by a high cloud density during most of the year, being greater during the DS because of air masses originating in the Pacific (Vuille et al. 2000) and low air evaporative demand, allowing the possibility to grow crops at full sun exposition (Jaimez et al. 2018).
The genotypes of C. arabica evaluated were sensitive to DS, showing a differential response among genotypes in A, gs, and E, without WUE being affected. Contrary, gas exchange was unaffected by water deficit in two C. canephora clones, although the water status and total leaf area were negatively affected by water deficit (DaMatta et al. 2003). Furthermore, a significant genotype × season interaction effect on A, E, gs, and WUE, suggested that the effect of DS on leaf gas exchange was different between the C. arabica genotypes studied. Higher E observed in Catucaí 785-15 and Acawá (6 μmol m-2 s-1), explains the lower WUE of these two genotypes compared to Sarchimor C-4260 and Aará (4 μmol m-2 s-1). Similar range of values of E have been reported in C. arabica (Tezara et al. 2018).
Drought-tolerant coffee genotypes could differ physiologically, presenting mechanisms such as osmotic adjustment, maximization of water uptake by deep root systems and/or minimization of water loss by stomatal closure and maximizing WUE, or by showing morpho-anatomical variations, among others (DaMatta 2004b, Tezara 2017). In our study, decrease of RWC and gs without changes in WUE was a general pattern suggesting water use optimization of coffee genotypes.
Drought may cause a reduction in A due to stomatal closure (decrease in Ci; Cornic 2000) and/or through impaired of metabolic processes (Lawlor & Tezara 2009). In almost all coffee genotypes closed their stomata (decrease gs) and avoided excessive water loss in response to drought, thus negatively affecting A. However, we do not rule out that metabolic factors may have played an important role in the regulation of A (Lawlor & Tezara 2009), since Ci was unaffected by drought (P = 0.22).
In both seasons, a significant linear relationship was found between A and gs for the 21 C. arabica genotypes, suggesting that A was dependent of gs. Similarly, this relationship was previously reported in C. arabica genotypes in Brazil and Ecuador (DaMatta et al. 2007, Tezara et al. 2018), but not in conilon coffee (Damatta et al. 2007) or in robusta coffee, suggesting that A was independent of gs in robusta coffee clones (Tezara Fernández et al. 2020).
A negative linear relationship between A and Δ W suggests a strong stomatal response of C. arabica to Δ W , i.e., the stomata tend to close in a dry atmosphere to avoid excessive loss of water that would translate into a leaf water deficit. A high stomatal sensitivity to increasing Δ W has been reported in C. arabica (Barros et al. 1997, Pinheiro et al. 2005, DaMatta & Ramalho 2006, Tezara et al. 2018). Indeed, Arabic coffee plants stomata respond to the evaporative demand of the atmosphere, which translates into a reduction in gs as the air becomes drier.
Catimor UFV 5608, Cavimor H-789, Mundo novo, Caturra amarillo 3386 and Aará genotypes showed a low potential production (40-465 Kg ha-1), whereas the highest yields (1320-1501 Kg ha-1) were found in the genotypes Catimor 8666, Catimor 8664, Catimor UFV 5607 and Catuaí UFV 2144. These results indicate that not all C. arabica genotypes have a high productive potential in Manabí and according to our results, the best adapted genotypes to the site conditions were Catimor 8666, Catimor 8664, Catimor UFV 5607 and Catuaí UFV 2144. In the best Catimor progenies, production has been reported as high as 526 to 570 g coffee gold plant -1 in Trujillo, Venezuela (Berlingeri et al. 2007) and 599 g plant-1 in hybrids of C. arabica (Marie et al. 2020), very close values to those obtained in Catimor 8666, which reached 500 g plant-1 in the present study. Contrary, Catuaí genotype produced 242 g plant-1 in Venezuela (Berlingeri et al. 2007, Bustamante et al. 2001); lower than Catuaí UFV 2144, which produced 440 g planta-1 in our study.
Our results indicate differences in physiological performance among the 21 genotypes of C. arabica studied. Significant differences in RWC and gas exchange variables occurred among RS and in DS trees, showing a wide range of variation in RWC, A, and gs. Catimor (UFV 5608, 8664, 8666) and Cavimor H-773 genotypes showed the highest WUE. Most of C. arabica genotypes were sensitive to DS but showed different responses, suggesting possible genotypic differences in tolerance. The response of RWC and gas exchange in the DS was differential, i.e., there was a different effect of DS on RWC and gas exchange depending on the genotype. There was no significant effect of DS on A, in Catimor (CIFC-P1, UFV 5607, CIFC-P2).
Long-term production of adult trees was significantly different among the C. arabica genotypes studied in Manabí province. The potential coffee production was low (401-465 kg ha-1) in Catimor UFV 5608, Cavimor H-789, Mundo novo, Caturra amarillo 3386 and Arará genotypes; while it was considerably higher (1,320-1,501 Kg ha-1) in Catimor 8666, Catimor 8664, Catimor UFV 5607 and Catuaí UFV 2144 genotypes.
We conclude that most C. arabica genotypes showed optimization of water use, some genotypes exhibited potential tolerance to DS; however, more physiological and production research is required to select genotypes with mechanisms that might be good alternatives for coffee plant breeding programs in drier environments.