Introduction
Water pollution resulting from anthropogenic activities has made wastewater treatment increasingly difficult and costly, since it requires physical, chemical and biological processes in conventional plants or those that use natural processes to comply with the discharge level established by current environmental regulations. Conventional systems, in most cases, are not accessible to many communities, mainly due to the construction, operating and maintenance costs, so it is necessary to apply affordable and environmentally friendly technologies (Comisión Nacional del Agua [CONAGUA], 2016). In this sense, constructed wetlands (CWs) comply with these characteristics because they have low effluent treatment costs, do not require chemical additives to remove pollutants, do not generate bad odors, have minimal greenhouse gas emissions, are aesthetic, are simple to operate and maintain with minimum electricity consumption, and can be applied in rural and urban areas (Arias & Brix, 2003; Ayaz, 2008; CONAGUA, 2014).
CWs imitate the processes that occur in natural wetlands (Ghosh & Gopal, 2010), through the cultivation of rooted macrophytes (emergent, floating or submerged) on a support medium (gravel, sand or others) and the presence of microorganisms in the root zone (Delgadillo, Camacho, Pérez, & Andrade, 2010). The plants absorb and assimilate pollutants, provide a large surface area for bacterial growth (nitrifiers), favor oxygen transport through the rhizosphere, stimulate organic matter degradation, and retain suspended solids by sedimentation and filtration. Some plant species are more dominant and have greater pollutant removal efficiency (Brix, 1994; Fernández-González, de Miguel-Beascoechea, de Miguel-Muñoz, & Fernández-de la Mora, 2004; Xiao, Peng, Yue, & Wen, 2007).
Romero-Aguilar, Colín-Cruz, Sánchez-Salinas, and Ortiz-Hernández (2009) evaluated the organic load removal from an institution’s domestic wastewater in three horizontal flow CWs with Phragmites australis and Typha dominguensis, installed after a primary treatment. Their results indicate that chemical oxygen demand (COD) was reduced by 90.2 to 95.73 %, total phosphorus (TP) by 3.13 to 40.35 %, and bacteria associated with the system by 1 x 105 CFU·mL-1 in wastewater and 1 x 107 CFU·g-1 in rhizosphere and substrate.
On the other hand, Amabilis-Sosa, Siebe, Moeller-Chávez, and Durán-Domínguez-de Bazúa (2016) determined the accumulation and distribution of mercury in wastewater with Phragmites australis, as a biological barrier, in nine CWs, of which three were inoculated with heavy metal-tolerant bacteria, three without inoculum and three without plants or inoculum. After 304 days of operation, systems with vegetation and metal-tolerant bacteria removed 73 % of the total mercury.
At the Institución Universitaria Colegio Mayor de Antioquia (IUCMA) in Colombia, two subsurface flow CWs (SFCWs) were implemented for the treatment of wastewater with Typha latifolia and Cyperus papyrus, both macrophytes. The results obtained for COD, biochemical oxygen demand (BOD5), total suspended solids (TSS) and TP with T. latifolia were 53.9, 83.2, 95.5 and 97.2 %, while with C. papyrus they were 47.9, 82.9, 40.3 and 95.9 %, respectively (Bedoya-Pérez, Ardila-Arias, & Reyes-Calle, 2014). It is important to note that each region of the world evaluates native macrophyte species to determine their phytoremediation potential in wastewater. Some of the most studied macrophytes are: Typha angustifolia (Park & Polprasert, 2008), Typha angustata (Ghosh & Gopal, 2010), Typha augustifolia (Arivoli & Mohanraj, 2013), Cyperus difformis and Dracaena sanderiana (Saeed, Al-Muyeed, Afrin, Rahman, & Sun, 2014).
In southeastern Mexico, particularly in Tabasco, there is a great diversity of swamp flora that has not been evaluated in wastewater treatment (Bueno, Álvarez, & Santiago, 2005). Among the species little studied in a CW are Sagittaria latifolia and Sagittaria lancifolia (Novelo, 2003); the latter has been evaluated in SFCWs as a tertiary treatment, but not as a primary or secondary one (Zachritz & Fuller, 1993). This species has also been used to remove nutrients from rainwater effluents (Lenhart, Hunt, & Burchell, 2012). Both species have the advantage of being smaller (better management and transport) than those used in the region's wetlands, and they are abundant in the Tabasco wetlands. In urban areas they are regarded as weeds, and it has been reported that certain varieties of these species are efficient in organic matter and nutrient removal (Lenhart et al., 2012; Zachritz & Fuller, 1993); therefore, it is important to generate more information on the ability of these plants to assimilate and remove pollutants.
Considering the above, the aim of this study was to evaluate the efficiency of Sagittaria latifolia and Sagittaria lancifolia in removing basic pollutants such as TSS, BOD5, COD and fecal coliforms (FCs) in nine free flow constructed wetlands (FFCWs).
Materials and methods
Wastewater treatment system (WTS). The system used is located in the División Académica de Ciencias Biológicas (DACBiol) of the Universidad Juárez Autónoma de Tabasco (UJAT), located in Villahermosa, Tabasco, Mexico (17° 59’ 26” and 17° 59’ 17” North latitude; 92° 58’ 16” and 92° 58’ 37” West longitude). It consists of nine independent FFCWs measuring 2.5 m long, 1.2 m wide and 1 m high, with a water operating level of 0.5 m. They are made of 10-gauge carbon steel sheet metal. The exterior is covered with anticorrosive alkyd enamel and the interior is protected with elastomeric sealant, with five layers of textile fiber. All are equipped with hydraulic PVC piping (0.05 m) to distribute the wastewater flow. They have a gravel bed 0.1 m deep and particle size between 2.36 and 19 mm, porosity of n = 49.6 ± 0.7 and permeability > 1 000, which works as a support for the vegetation. They have a distribution tank with a capacity of 200 L, which is fed by pumping from a cistern through a hydraulic PVC pipe. During this study, the FFCWs operated with a flow rate of 0.26 ± 0.1 m3·day-1 and a hydraulic retention time (HRT) of 6.05 ± 2.1 days.
Collection, planting and stabilization of vegetation. Sagittaria latifolia plants were collected in a flooded area in Villahermosa, Tabasco, Mexico (17° 59.219' North latitude and 92° 57.588' West longitude), while Sagittaria lancifolia was extracted from the Pantanos de Centla Biosphere Reserve, Tabasco (18° 18.952' North latitude and 92° 32.376' West longitude). Whole young plants, including flowers and fruits, were collected for subsequent planting and taxonomic identification according to Zepeda-Gómez and Lot (2005).
The collected specimens were placed in 20 L containers with water from the natural environment, to avoid stress. Both types of vegetation were planted in a wastewater canal, in order to adapt to the new environment, where they remained for 10 days. Subsequently, they were transplanted to the FFCWs. Fifteen individuals were planted for each FFCW. Water depth was kept at 0.3 m for 14 days to decrease the growth and propagation time. At 24 days after planting, the water level in the FFCWs was increased to 0.5 m to start the stabilization period, which is not achieved until the vegetation reaches maturity and the bacterial film is formed in the rhizosphere and support medium, a process that can last up to three months (Valles-Aragón, & Alarcón-Herrera, 2014).
The entire process was carried out from August to September 2015, and the evaluation phase began in September 2016. This is because the best efficiency in wetlands is achieved between the first and second year of operation (Amabilis-Sosa et al., 2016; Torres-Bojorges, Hernández-Razo, Fausto-Urquieta, & Zurita-Martínez, 2017).
Wastewater analysis. The wastewater used in the experiments was obtained from the DACBiol-UJAT domestic wastewater concentrator sump (storage tank), which generates a flow of 120 m3·day-1. Thirty-six samplings were carried out over the September-November period of 2016. In each sampling, ten samples were taken at 12:00 hours, one from the distribution tank tributary and nine from the FFCW effluent, giving a total of 360 simple samples. Temperature and pH (field parameters) were measured three times a week with a multiparameter instrument (HI 9828, Hanna InstrumentMR, US). On the other hand, the TSS, BOD5, COD and FCs (laboratory parameters) were determined in accordance with Mexican standards (Table 1), for which the minimum number of samplings was considered, which was one per week, giving 120 simple samples. Pollutant removal efficiency was determined in accordance with Torres-Bojorges et al. (2017).
Table 1 Methods used for the parameters evaluated.
Variable | Method |
---|---|
Temperature | In accordance with ISO 7027 |
pH | EPA 9040B / SM 4500 H+B |
Total suspended solids | NMX-AA-034-SCFI-2001 |
Biochemical oxygen demand | NMX-AA-028-SCFI-2001 |
Chemical oxygen demand | NMX-AA-030-SCFI-2001 |
Fecal coliforms | NMX-AA-042-SCFI-1987 |
Harvest. This activity is part of the routine maintenance and control of the process in the FFCWs. It consists of removing vegetation that is deteriorated or dead, since it no longer assimilates pollutants. If this action is not taken, it has a negative effect on removal efficiency, as there is free decomposition of pollutants previously processed, which increases the BOD5 concentration in the effluent from the treatment units (Lot, Olvera, Flores, & Díaz, 2015; Vera et al., 2010).
At the beginning of the vegetation assessment phase, the stem was manually pruned to 0.1 m above water level (0.5 m). This process was carried out again at the end of the period to account for the biomass generated. The collected vegetation was deposited in 20 L containers for weighing with a spring balance with a capacity of 20 kg. In addition to weight, the height (cm), moisture (%), and leaf width and length (cm) were determined. Also, the bacterial biomass adhering to the support medium (on the rocks) was quantified by gravimetry (volatile matter), by the SVT-adapted method (NMX-AA-034-SCFI-2001); this was done in duplicate for each CW (giving a total of 18 samples).
Experimental design and statistical analysis. In this study, a one-factor design with three treatments was used: FFCW without vegetation (FFCW-Control), CW with Sagittaria lancifolia (FFCW-S-Lan) and CW with Sagittaria latifolia (FFCW-S-Lat), with three replicates each (Figure 1).
To find differences among treatments (FFCW-Control, FFCW-S-Lan and FFCW-S-Lat), the Kruskal Wallis test was performed, followed by the Mann-Whitney test for median comparison for the variables TSS, BOD5, FCs and plant biomass. For COD a one-way analysis of variance was performed and then Tukey’s test (P ≤ 0.05). The data were analyzed in the Statgraphics 7.0MR statistical package.
Results and discussion
Temperature. The average wastewater temperature at the end of the experiment was 26.2 ± 0.9, 27.3 ± 0.5 and 26 ± 0.8 °C, for the FFCWs-S-Lat, FFCWs-S-Lan and FFCWs-Control, respectively (Table 2), values that are below that set out by NOM-001-SEMARNAT-1996 (40 °C) for the protection of aquatic life. This parameter remains constant among treatments, and is an important factor in the growth of mesophilic microorganisms that favor the process of assimilation of pollutants by plants and their decomposition (Vymazal, 2007). It is known that high temperatures affect fauna, aquatic flora and decomposition of organic matter by reducing dissolved oxygen; if it is greater than 50 °C, aerobic digestion and bacterial nitrification are stopped, and if it is less than 5 °C, microbial activity is inhibited (Crites & Tchobanoglous, 2000). Therefore, the recorded temperatures are considered to be optimal for pollutant removal.
Table 2 Average weekly values of the parameters evaluated in the different treatments.
Treatment | Day | TSS1 (mg·L-1) | BOD5 (mg·L-1) | COD (mg·L-1) | Temperature (°C) | pH |
---|---|---|---|---|---|---|
Sump | 09/09/2016 | 329.4 | 254.2 | 635.6 | 25.9 | 7.3 |
16/09/2016 | 324 | 375.5 | 938.8 | 25.5 | 7.4 | |
23/09/2016 | 353.7 | 369.4 | 923.4 | 25.7 | 7.4 | |
30/09/2016 | 383.4 | 437.1 | 979.3 | 25.9 | 7.3 | |
07/10/2016 | 343.6 | 391.7 | 1008 | 26.9 | 8.2 | |
14/10/2016 | 357.7 | 403.2 | 1019.3 | 28.3 | 8 | |
21/10/2016 | 223 | 407.7 | 1092.7 | 28.3 | 8 | |
28/10/2016 | 308.1 | 400.9 | 1040 | 27.83 | 8.07 | |
04/11/2016 | 345 | 400 | 1010 | 23.8 | 8 | |
11/11/2016 | 367 | 387 | 1035 | 24 | 8.4 | |
18/11/2016 | 370 | 365 | 998 | 24.9 | 8.6 | |
25/11/2016 | 310 | 409 | 970 | 24.8 | 8 | |
FFCW-Control | 09/09/2016 | 91 | 150 | 220 | 25.8 | 8 |
16/09/2016 | 94 | 168 | 250 | 25 | 8.1 | |
23/09/2016 | 100 | 170 | 289 | 25.8 | 8 | |
30/09/2016 | 103 | 165 | 198 | 25.8 | 7.5 | |
07/10/2016 | 110 | 178 | 194 | 26.5 | 8 | |
14/10/2016 | 97 | 169 | 238 | 26 | 7.9 | |
21/10/2016 | 89 | 175 | 270 | 27.5 | 7.9 | |
28/10/2016 | 110 | 160 | 195 | 27.5 | 8 | |
04/11/2016 | 98 | 175 | 175 | 25.7 | 7.8 | |
11/11/2016 | 88 | 187 | 188 | 25.8 | 8.2 | |
18/11/2016 | 90 | 172 | 200 | 25.3 | 8.4 | |
25/11/2016 | 120 | 164 | 210 | 25 | 7.9 | |
FFCW-S-Lan | 09/09/2016 | 13.1 | 21.1 | 52.7 | 26.8 | 7.5 |
16/09/2016 | 12.7 | 23.3 | 58.2 | 27 | 7.5 | |
23/09/2016 | 12.9 | 18 | 45.1 | 27 | 7.5 | |
30/09/2016 | 12.9 | 23.5 | 58.7 | 26.9 | 7.5 | |
07/10/2016 | 7 | 24.3 | 60.6 | 27 | 7.5 | |
14/10/2016 | 2.3 | 24.6 | 61.6 | 27 | 7.4 | |
21/10/2016 | 7.3 | 24.6 | 61.6 | 28 | 7.8 | |
28/10/2016 | 5.53 | 24.5 | 61.27 | 27.33 | 7.57 | |
04/11/2016 | 11.9 | 24.8 | 59.9 | 28 | 7.4 | |
11/11/2016 | 12.5 | 23.9 | 62.7 | 27 | 7.7 | |
18/11/2016 | 13.5 | 25 | 65.8 | 28 | 7.5 | |
25/11/2016 | 13.1 | 25.5 | 67.3 | 27 | 7.5 | |
FFCW-S-Lat | 09/09/2016 | 18.5 | 14.9 | 37.3 | 25 | 7.8 |
16/09/2016 | 20.4 | 14.5 | 36.2 | 24.9 | 7.8 | |
23/09/2016 | 15.8 | 14.7 | 36.8 | 25.1 | 7.8 | |
30/09/2016 | 20.6 | 14.7 | 36.8 | 25 | 7.6 | |
07/10/2016 | 21.3 | 7.9 | 19.9 | 26.9 | 7.7 | |
14/10/2016 | 21.6 | 2.6 | 6.5 | 26.4 | 7.7 | |
21/10/2016 | 21.6 | 8.3 | 20.8 | 27.3 | 7.7 | |
28/10/2016 | 21.5 | 6.27 | 15.73 | 26.87 | 7.7 | |
04/11/2016 | 20.8 | 14.4 | 35.7 | 26.87 | 7.7 | |
11/11/2016 | 22.7 | 15.5 | 33.9 | 26.86 | 7.7 | |
18/11/2016 | 21.8 | 14.9 | 37.8 | 26.86 | 7.7 | |
25/11/2016 | 22.5 | 15.2 | 37.2 | 26.86 | 7.7 |
1TSS = total soluble solids; BOD5 = biochemical oxygen demand; COD = chemical oxygen demand; FFCW-Control = free flow constructed wetlands without vegetation; FFCW-S-Lan = free flow constructed wetlands with Sagittaria lancifolia; FFCW-S-Lat = free flow constructed wetlands with Sagittaria latifolia.
Hydrogen potential (pH). The average pH of the wastewater in the FFCWs-S-Lat, FFCWs-S-Lan and FFCWs-Control was 7.7 ± 0.1, 7.5 ± 0.1 and 8.0 ± 0.2, respectively (Table 2). These values comply with the level stipulated in NOM-001-SEMARNAT-1996, since it establishes that the maximum permissible pH limit is 5 to 10. On the other hand, the results obtained agree with the findings reported by Allen, Hook, Biederman, and Stein (2002), who state that the microbial nitrification processes release H+, which causes the pH to decrease. However, the decrease in pH is not greater, mainly due to the interactions between the substrate and the biofilm, the presence of plants and the action of the CaCO3 present in the substrate (Kadlec & Wallace, 2009).
Total suspended solids (TSS). The removal of solids within wetlands occurs due to sedimentation and filtration, as the roots of the macrophytes and the support medium reduce the velocity of the water within the CW. Much of the removal occurs in the first few centimeters of the inlet area, due to the calm conditions and shallowness of the water in the system (Delgadillo et al., 2010; Karathanasis, Potter, & Coyne, 2003). In this study, the sump effluent presented on average 334.58 mg·L-1, and with the treatments the values were 99.2 ± 10, 10.4 ± 3.8 and 20.8 ± 1.9 mg·L-1 with 70, 97 and 94 % removal efficiency for the FFCWs-Control, FFCWs-S-Lan and FFCWs-S-Lat, respectively (Table 2). These results comply with the maximum permissible limits of NOM-001-SEMARNAT-1996 and NOM-003-SEMARNAT-1997, which are 40 mg·L-1 for the protection of aquatic life and 30 mg·L-1 for indirect or occasional public contact.
The FFCWs in this study achieved greater removal efficiencies than those reported by Pérez-Salazar, Alfaro-Chinchilla, Sasa-Marín, and Agüero-Pérez (2012), who treated domestic wastewater from an industrial sector in a tropical area of Costa Rica using FFCWs with Cyperus papyrus, and achieved 73 % TSS removal. Another pilot scale FFCW system, proposed by Valipour, Raman, and Ghole (2009), with emergent vegetation of Phragmites sp., presented 86 % TSS removal.
The Kruskal-Wallis test proved that the treatments have a statistically significant effect (P < 0.0016) on the decrease of TSS, with 95 % reliability. FFCWs-S-Lan had the lowest value (12.6 ± 3.80 mg·L-1), followed by FFCWs-S-Lat (21.4 ± 1.90 mg·L-1). On the other hand, the Mann-Whitney test indicated statistically significant differences (P < 0.05) among the treatment medians, with a 95 % confidence level (Figure 2).
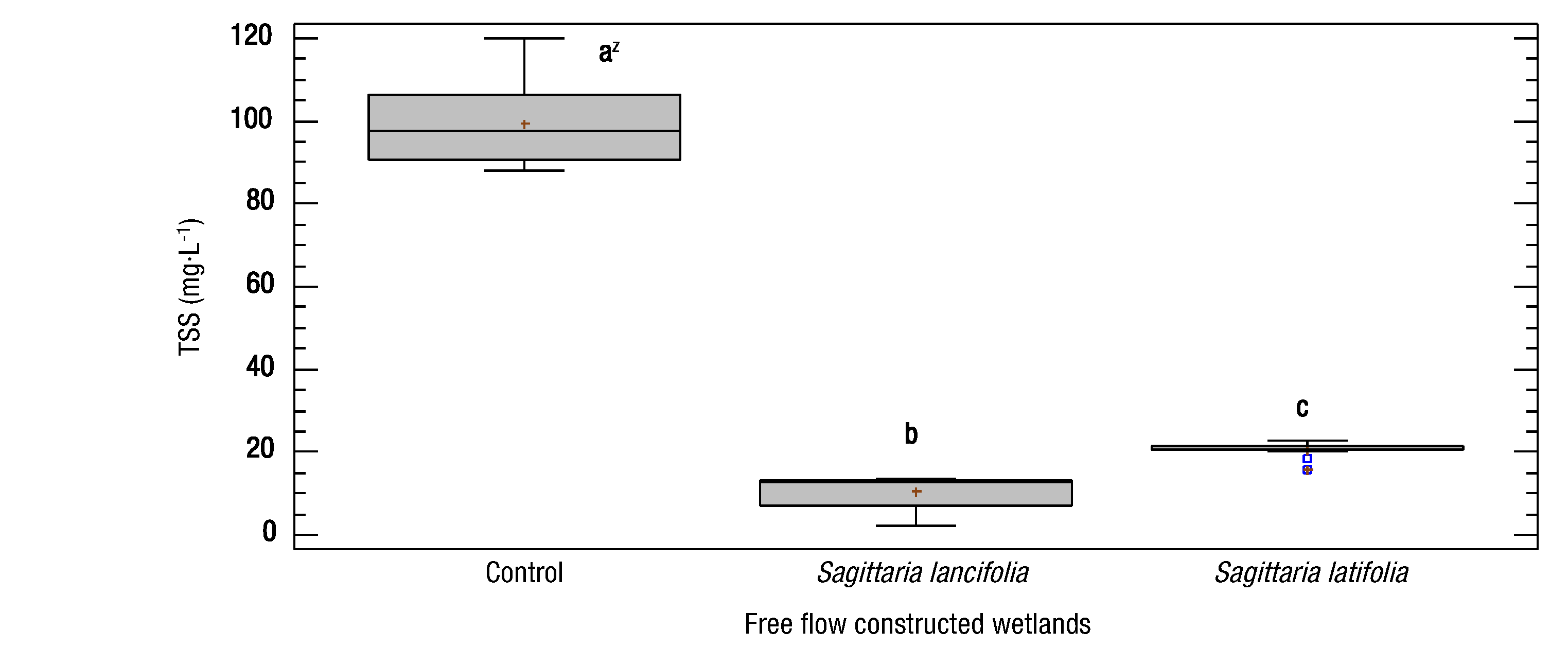
Figure 2 Median values (± standard deviation) of total suspended solids (TSS) obtained from the different constructed wetland treatments evaluated. zMeans with the same letter between treatments do not differ statistically (Mann-Whitney, P ≤ 0.05).
Biochemical oxygen demand (BOD5). The sump wastewater had an average value of 383.39 mg·L-1 in BOD5; after the treatments the values obtained were 23.59 and 11.99 mg·L-1 with FFCWs-S-Lan and FFCWs-S-Lat, respectively. Both treatments comply with NOM-001-SEMARNAT-1996 and NOM-003-SEMARNAT-1997, which establish as maximum limits 30 mg·L-1 BOD5 for the protection of aquatic life and 20 mg·L-1 for service to the public with indirect or occasional contact.
BOD5 found in soluble form is removed by biological degradation (aerobic and anaerobic) carried out by microorganisms adhering to the surface of plants, detritus, the water column (as in the FFCWs) and the support medium; therefore, the level of BOD5 removal in CWs is generally high (Abidi, Kallali, Jedidi, Bouzaiane, & Hassen, 2009).
BOD5 removal efficiency in the FFCWs-S-Lan was 94 % and in the FFCWs-S-Lat it was 97 %. These averages are higher than those reported by Valipour et al. (2009), who establish that CWs with Phragmites sp. vegetation have a BOD5 removal efficiency of 86 %. Solís-Silván, López-Ocaña, Bautista-Margulis, Hernández-Barajas, and Romellón-Cerino (2016) assessed surface and subsurface flow CW at pilot scale with T. dominguensis vegetation for domestic wastewater treatment, and found that FFCWs have higher BOD5 removal efficiency (97 %) than SFCWs, results similar to the efficiencies obtained in this research.
The Kruskal-Wallis test indicated that the treatments have a statistically significant effect (P < 0.0146) with respect to the decrease in BOD5, with 95 % reliability. FFCWs-S-Lat recorded the lowest median value (14.6 ± 4.44 mg·L-1), followed by FFCWs-S-Lan (24.4 ± 2.09 mg·L-1) and FFCWs-Control (169.5 ± 9.38 mg·L-1). The Mann-Whitney test showed statistically significant differences (P < 0.05) among the treatment medians, with a 95 % confidence level (Figure 3).

Figure 3 Median biochemical oxygen demand values (± standard deviation) of the different constructed wetland treatments analyzed. zMeans with the same letter between treatments do not differ statistically (Mann-Whitney, P ≤ 0.05).
Chemical oxygen demand (COD). Verhoeven and Meuleman (1999) point out that high COD removal rates are caused by sedimentation of suspended solids, and by rapid decomposition processes in the water and upper substrate layers. This is evident in this work, since the average COD value of the sump wastewater was 970.8 mg·L-1, and at the conclusion of the treatment the FFCWs-S-Lan had 59.6 mg·L-1 and the FFCWs-S-Lat 29.6 mg·L-1, with a removal efficiency of 93 and 96 % respectively. These percentages are higher than those obtained by Mexico’s Secretaría de Medio Ambiente y Recursos Naturales (SEMARNAT, 2012) in a CW for the treatment of wastewater generated by rural communities, where 60 and 75 % COD removal efficiencies were obtained. For their part, Romero-Aguilar et al. (2009) obtained COD removal efficiencies of 90.2 % with a pilot scale CW with Phragmites australis and Typha dominguensis. Bedoya-Pérez et al. (2014) conducted a study with a SFCW for waters similar to those of this study (institutional) and achieved a COD decrease with an HRT of nine days for T. latifolia and seven days for C. papirus (79.4 and 68.1 % respectively).
The BOD5/COD ratio obtained in this study in the sump wastewater was 0.39, so it can be stated that this effluent is difficult to degrade; that is, most of the organic and inorganic matter present cannot be biochemically oxidized, so other processes such as oxidation, reduction, volatilization, adsorption, absorption, sedimentation and filtration intervene (Bedoya-Pérez et al., 2014; Crites & Tchobanoglous, 2000; Karathanasis et al., 2003).
The one-way analysis of variance showed that the treatments have a statistically significant effect (P < 0.002) in the reduction of COD, with 95 % reliability. This allowed determining that the FFCWs-S-Lat had the lowest average (29.5 ± 10.80 mg·L-1), followed by the FFCWs-S-Lan (59.62 ± 5.87 mg·L-1) and the FFCWs-Control (218.91 ± 35.42 mg·L-1). Tukey's a posteriori multiple comparison test indicated statistically significant differences among the different types of treatments evaluated (P < 0.05), with a 95 % confidence level (Figure 4).
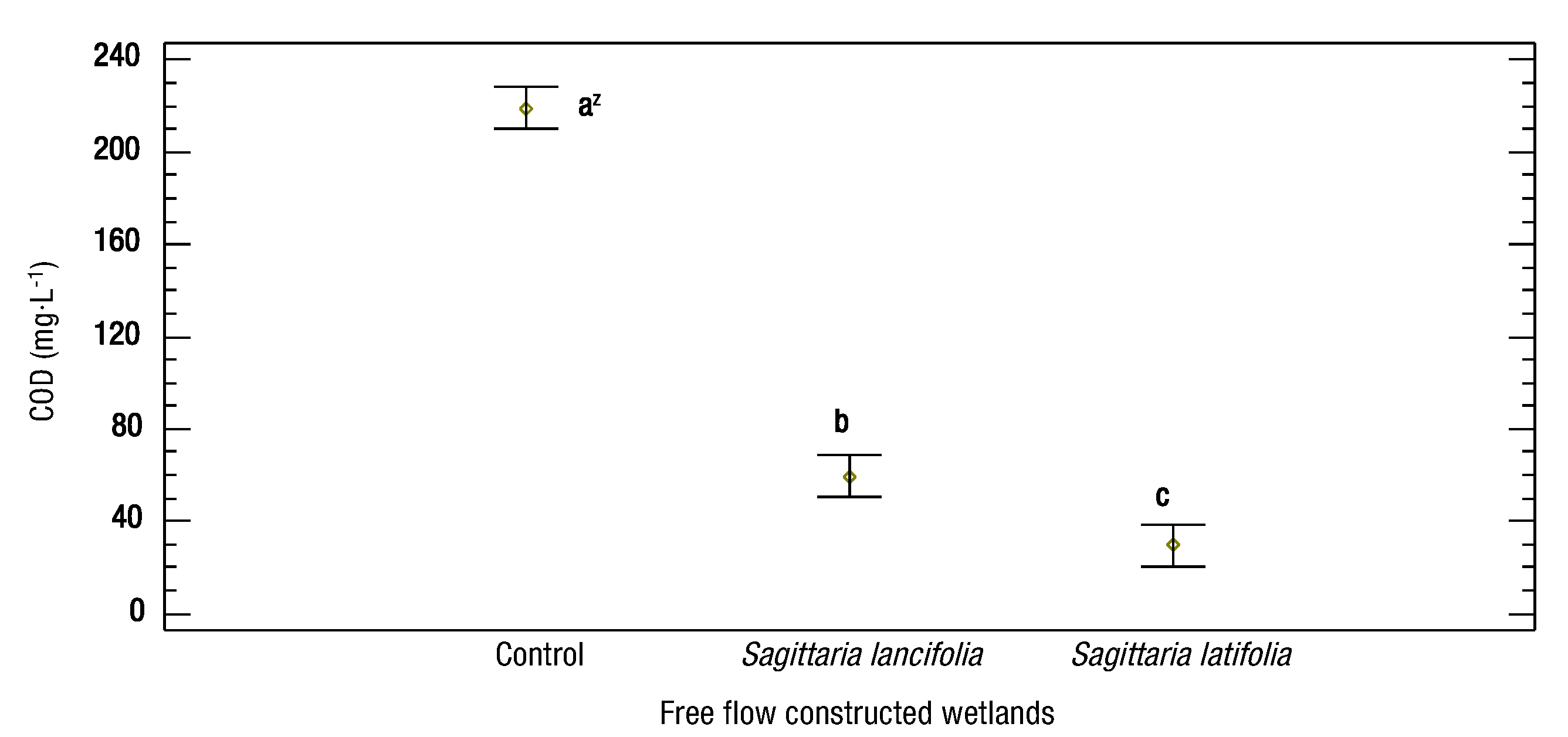
Figure 4 Average chemical oxygen demand values of the different constructed wetland treatments evaluated. zMeans with the same letter between treatments do not differ statistically (Tukey, P ≤ 0.05).
Fecal coliforms (FCs). The sump wastewater presented an average of 100 000 MPN·100 mL-1, and at the end of treatment it was 100 MPN·100 mL-1 in the FFCWs-S-Lan and 1 000 MPN·100 mL-1 in the FFCWs-S-Lat. Based on the results obtained, it was determined that the maximum permissible limit established by NOM-003-SEMARNAT-1997 (1 000 MPP·100 mL-1 for public service with indirect or occasional contact) is met. The FC removal efficiency was 99.9 % in the FFCWs-S-Lan and 99 % in the FFCWs-S-Lat. Keith et al. (2003) state in their study that the main microorganisms in CWs, such as FCs, enterococci, Salmonella, Shigella, Yersinia and coliphages, are removed with efficiencies of 80 to 99 % with Typha latifolia, Effusus and Scirpus validus. In addition to the extensive vegetation, processes that contribute to bacterial removal in CWs are sedimentation, aggregation, oxidation, filtration, solar irradiation, antibiosis, predation and competition (Gersberg, Lyon, Brenner, & Elkins, 1987).
The Kruskal-Wallis test indicated that the treatments have a statistically significant effect (P < 0.02) with respect to the reduction of FCs, with 95 % reliability. The FFCWs-S-Lat had the lowest FC amount in terms of the median value (1 000 MPN·100 mL-1), followed by the FFCWs-S-Lan (100 MPN·100 mL-1) and the CWs-Control (100 000 MPN·100 mL-1). The Mann-Whitney test indicated statistically significant differences (P < 0.05) among treatment medians, with a 95 % confidence level (Figure 5).
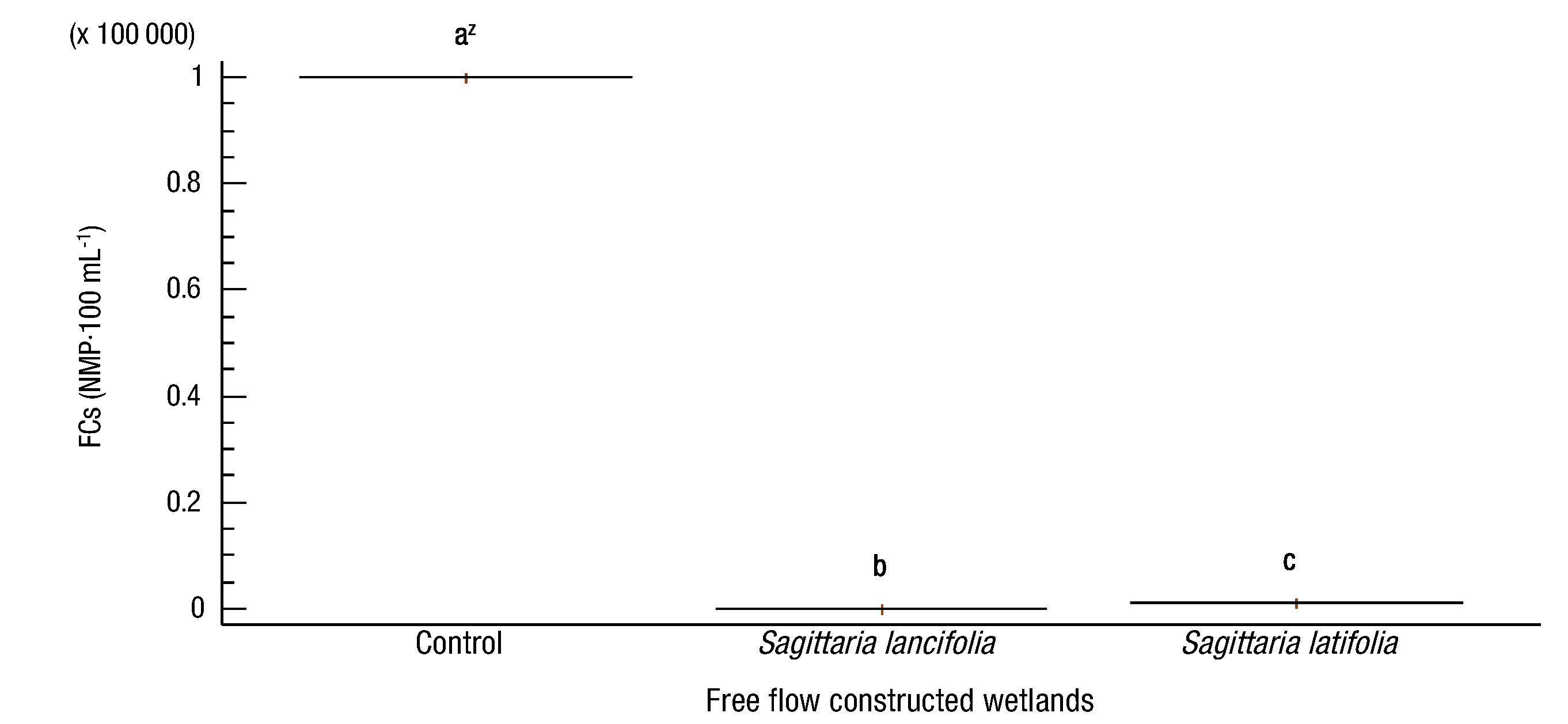
Figure 5 Median fecal coliform values (± standard deviation) of the different constructed wetland treatments evaluated. zMeans with the same letter between treatments do not differ statistically (Mann-Whitney, P ≤ 0.05).
Bacterial biomass. Microorganisms adhering to the support medium are also responsible for the degradation of organic matter; for this reason, the production of microorganisms per unit mass of the support medium was determined. In this sense, the FFCWs-Control had a production of 4 638.0 mg·kg-1, the FFCWs-S-Lan of 29 940.0 mg·kg-1 and the FFCWs-S-Lat of 8 541.83 mg·kg-1.
The Kruskal-Wallis test indicated that the treatments have a statistically significant effect (P < 0.05) on the presence of microorganisms, with 95 % reliability. The FFCWs-Control had fewer microorganisms, with a median of 5 033.0 ± 1 196.36 mg·kg-1, followed by the FFCWs-S-Lat with 7 702.5 ± 2 766.28 mg·kg-1 and the FFCWs-S-Lan with 27 458.5 ± 13 135.6 mg·kg-1. The Mann-Whitney test indicated statistically significant differences (P < 0.05) among treatment medians, with a 95 % confidence level (Figure 6).
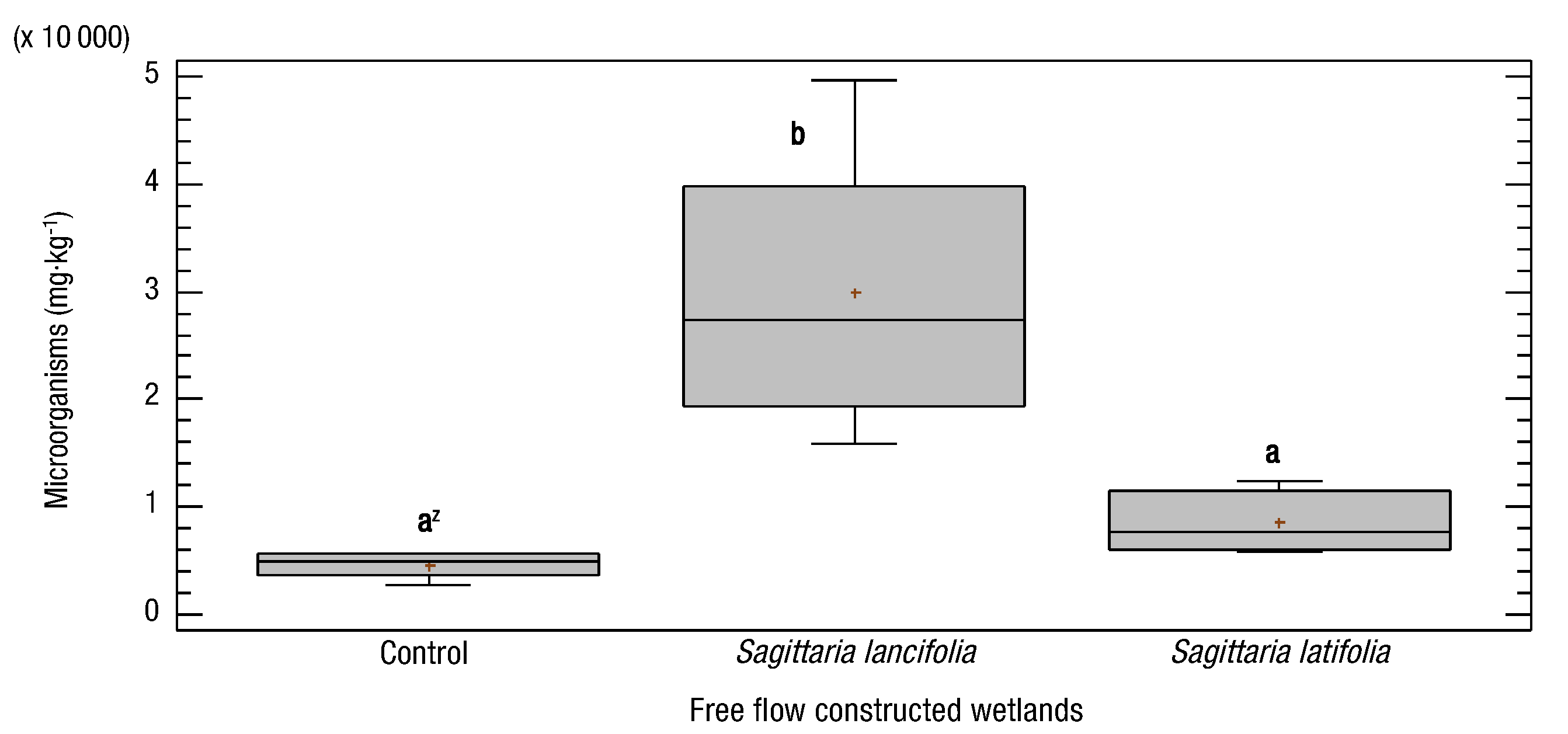
Figure 6 Median bacterial biomass values (± standard deviation) of the different constructed wetland treatments evaluated. zMeans with the same letter between treatments do not differ statistically (Mann-Whitney, P ≤ 0.05).
Plant biomass. The growth of Sagittaria latifolia and Sagittaria lancifolia may vary according to the environmental conditions where they are found. The main parameters influencing plant growth and propagation are temperature, organic matter, available nutrients and water quantity, as excessive water will affect vegetation development (Delgadillo et al., 2010).
For Sagittaria latifolia, the stems reached an average height of 114.5 ± 0.8 cm, and the leaves were 23.10 ± 8.4 cm long and 20.67 ± 2.3 cm wide. At the beginning of the treatment, the biomass was 24.3 ± 1.6 kg and at the end it was 55 ± 1.5 kg, with 61 % moisture. In the case of Sagittaria lancifolia, the stems had a height of 145.7 ± 3.4 cm, and its leaves were 54 ± 5.4 cm long and 17.8 ± 3.4 cm wide. The initial biomass of the planted vegetation was 30.5 ± 0.5 kg and at the end of the experiment it was 78 ± 1 kg. It should be noted that at the end of the experiment the plant had an average of 68 % moisture.
These results show that there is a direct relationship between plant biomass and microorganism establishment, as Sagittaria lancifolia presented more plant biomass and more microorganisms in the support medium. These results are different from those of Romero-Aguilar et al. (2009), who assert that the microorganisms present as biofilm on the support medium and in plant roots do not show a correlation between the number of bacteria and the species of the plant; however, the vegetation that attains the highest biomass achieves greater nutrient uptake and has a vigorous root system that facilitates the growth of the biofilm and the plant itself (Crites, Gunther, Kruzic, Pelz, & Tchobanoglous, 1988).
The Kruskal-Wallis test indicated that the treatments have a statistically significant effect (P < 0.04) on the generation of plant biomass, with 95 % reliability. The results show that the lowest median value was recorded in the FFCWs-S-Lat (18 ± 1.5) and the highest in the FFCWs-S-Lan (26.5 ± 1). The Mann-Whitney test indicated statistically significant differences (P < 0.05) among the treatment medians, with a 95 % confidence level (Figure 7).
Conclusions
The pilot scale FFCWs with Sagittaria latifolia and Sagittaria lancifolia vegetation are efficient in the removal of basic pollutants from domestic wastewater.
The water treated in the FFCWs complied with the maximum permissible limits established by standards NOM-001-SEMARNAT-1996 and NOM-003-SEMARNAT-1997, so the treated wastewater can be reused. It is important to highlight that the removal efficiencies obtained may vary according to the climatic conditions of the area where CWs are developed, the HRT, the type of support used and the characteristics of the raw wastewater.
The potential observed in the FFCWs with Sagittaria latifolia and Sagittaria lancifolia is that the use of these species could be implemented with a sustainable management system in natural wetlands to reduce the organic and nutrient load, which through residual and agricultural inputs reach the lotic or lentic water bodies in urban or rural areas.
Finally, the use of Sagittaria latifolia and Sagittaria lancifolia can be recommended for the establishment of CWs in the tropical areas of Mexico or geographical areas with similar conditions to those of this region.