Highlights:
Sacred fir provenances correspond to an altitudinal transect from 2 850 to 3 540 m.
Terpene diversity and concentration in sacred fir populations vary at different altitudes.
Terpene diversity decreases as elevation increases, but concentration increases.
Three altitude groups were identified according to the chemical profile of populations.
Low altitude provenances showed similarity of chemical profiles.
Introduction
Sacred fir (Abies religiosa [Kunth] Schltdl. & Cham.) forests are distributed in conifer-dominated upland areas, mainly between 2 400 and 3 600 m altitude, between 19° and 20° north latitude, along the Trans-mexican Volcanic Belt (Sáenz-Romero, Rehfeldt, Duval, & Lindig-Cisneros, 2012). These forests are of great ecological importance due to the ecosystem services they provide such as carbon sequestration, water uptake, and protection against erosion (Hernández-Álvarez, Reyes-Ortiz, Villanueva-Díaz, & Sánchez-González, 2021); they are also a wintering site for the monarch butterfly, Danaus plexippus L. (Oberhauser, Nail, & Altizer, 2015).
Sacred fir is an evergreen monoecious tree up to 40 m tall, with thick bark with scaly patches in adult trees and smooth in young trees. Its leaves are alternate, linear, sessile, straight, somewhat leathery, 19 to 70 mm long, with acute or rounded apex and oblong buds protected with resin (Rodríguez Trejo, Aparicio Lechuga, Lara Bueno, Uribe Gómez, & Ramírez Castell, 2021).
One of the main threats to sacred fir forests is climate change, because it will induce a decoupling between their populations and the climate suitable for them (Gómez-Pineda et al., 2020; Sáenz-Romero et al., 2012), which in turn will lead to increasing incidence of bark beetles, as high temperatures provide favorable conditions for the development of these insects (Gómez-Pineda et al., 2022; López-Gómez, Arriola Padilla, & Pérez Miranda, 2015; Rubin-Aguirre et al., 2015; Sosa Díaz et al., 2018).
Populations of the same species with a wide range of distribution are found in a diverse variety of environmental conditions that can be reflected in phenotypic variation (Premoli, Acosta, Mathiasen, & Donoso, 2012). This can be explained because of differential selection of genotypes under the various environmental conditions in an environmental gradient (Ignazi, Mathiasen, & Premoli, 2019). Understanding altitudinal patterns of genetic differentiation among populations is essential for the design of forest management measures that facilitate the adaptation of forest populations to projected climates (Sáenz-Romero et al., 2016). In mountainous regions, the altitudinal gradient is especially important for understanding richness patterns at both community and population levels (Muenchow, Dieker, Kluge, Kessler, & von Wehrden, 2018).
Provenance or common garden trials under open field conditions allow expression of genetic differences by growing plants from different geographical origins (provenances) under the same environmental conditions. In this type of studies, it is expected that the provenance best coupled to the climate of the trial site will have the best performance and the others will show differences, in part, related to the magnitude of the weather differences between the site of origin and the trial site (Cruzado-Vargas et al., 2021; Sáenz‐Romero et al., 2017). A variant of the above are the common garden trials where optimal conditions are provided (as far as possible) to the set of provenances to favor the expression of their growth potential, and with this, quantify whether or not there is genetic differentiation between them. This can be achieved by establishing trials in open field sites located within the optimal climate space of the species, or, as in the case of this study, by providing favorable artificial conditions using shade netting and irrigation during the dry season (Ortiz-Bibian et al., 2017).
Defense and resistance mechanisms in conifers depend on genetic, biotic and abiotic factors and interactions. These mechanisms include physical defense traits and chemical compounds (Zas Arregui & Sampedro Pérez, 2015). Chemical defenses often take the form of sets of substances that defend plants against herbivores (including bark beetles) (Taft, Najar, Godbout, Bousquet, & Erbilgin, 2015), pathogens (Kopaczyk, Warguła, & Jelonek, 2020) and certain types of abiotic stresses such as high temperature (Materić et al., 2015).
Plants synthesize a large number of compounds, including secondary metabolites, many are volatile compounds that vary in composition and concentration between species and from one individual to another within the same species (García-Rodríguez, Bravo-Monzón, Martínez-Díaz, Torres-Gurrola, & Espinosa-García, 2012). Such variation also depends on the plant part being analyzed, the season of the year, geographical origin and other environmental factors (Kopaczyk et al., 2020). Secondary metabolites are synthesized in small quantities and not in a generalized way (some are produced only by one species, genus or family) (Pichersky & Raguso, 2018); they play defense, attraction or competition functions, and their effectiveness depends on the concentration (López-Goldar et al., 2018) and metabolism of the consumer: herbivore or pathogenic (García-Rodríguez et al., 2012).
Terpenes constitute the largest and most diverse group of secondary metabolites in plants (Kopaczyk et al., 2020) comprising more than 40 000 individual compounds (Abbas et al., 2017) and, together with phenolic compounds, are the main chemical defenses in conifers (Lundborg, Fedderwitz, Björklund, Nordlander, & Borg-Karlson, 2016). The most abundant terpenes in the pine family are α-pinene, β-pinene, myrcene, limonene, camphene, and 3-carene (Kopaczyk et al., 2020; Pokorska et al., 2012; Taft et al., 2015).
The objective of this study was to determine whether the phytochemical diversity of A. religiosa varies among 14 populations from an altitudinal gradient (2 850 to the 3 550 m) established in a common garden trial. The hypothesis is that terpene diversity will be higher at lower altitudes, where there is higher herbivory and, therefore, greater selection pressure that would eventually favor terpene diversity as an adaptive response of forest populations (Pellissier et al., 2016).
Materials and Methods
Seed collection and plant production
Mature cones of A. religiosa were collected at 14 sites: 11 trees at each site separated by 50 m altitudinal difference in a transect from 2 850 to 3 550 m (Table 1) on Cerro San Andrés, Municipio Libre de Hidalgo, Michoacán, Mexico. Collection sites were well-preserved stands, dominated mainly by A. religiosa (89 % basal area) in the upper part of the mountain, combined with Pinus hartwegii Lindl. (pino de las alturas) and Cupressus lindleyi Klotzsch ex Endl. (cedar). In the intermediate part of the altitudinal transect, A. religiosa represents 60 % of the basal area of the tree stand in combination mainly with Pinus pseudostrobus Lindl. (pino liso), C. lindleyi and Salix paradoxa Kunth (borreguito). At the lowest part, A. religiosa represents 45 % in combination with P. pseudostrobus, Pinus montezumae Lamb (pino blanco), Quercus obtusata Bonpl. (white oak) and other leafy species such as Arbutus xalapensis Kunth (madroño) and S. paradoxa. The detailed description of the sites can be found in the study by Ortiz-Bibian et al. (2017).
The cones were dried in the sun for 15 days to extract the seeds and, subsequently, germinate them in a peat moss seedbed. The seedlings were transplanted into 380 cm3 containers, where they developed for nine months. In March 2014, a provenance or common garden trial (Figure 1) was set up at the Instituto de Investigaciones sobre los Recursos Naturales de la Universidad Michoacana de San Nicolás de San Nicolás de Hidalgo (INIRENA-UMSNH) located in Morelia, Michoacán. The experiment had a six randomized complete block design with six seedlings per provenance (Ortiz-Bibian et al., 2017).
Table 1 Geographical location of the cone collection sites of 14 provenances of Abies religiosa on Cerro San Andrés, Municipio Libre de Hidalgo, Michoacán, Mexico.
Altitude (m) | Latitude (N) | Longitude (W) |
---|---|---|
3 540 | 19° 48’ 01.0” | 100° 36’ 04.7” |
3 500 | 19° 48’ 03.6” | 100° 36’ 06.8” |
3 450 | 19° 48’ 06.3” | 100° 36’10.3” |
3 400 | 19° 48’ 19.0” | 100° 36’ 13.5” |
3 350 | 19° 48’ 20.2” | 100° 36’ 13.8” |
3 300 | 19° 48’ 23.8” | 100° 36’ 18.6” |
3 250 | 19° 48’ 36.1” | 100° 36’ 16.3” |
3 200 | 19° 48’ 38.0” | 100° 36’ 20.6” |
3 150 | 19° 48’ 40.9” | 100° 36’ 26.4” |
3 100 | 19° 48’ 49.0” | 100° 36’ 31.2” |
3 050 | 19° 48’ 58.7” | 100° 36’ 30.6” |
3 000 | 19° 49’ 10.1” | 100° 36’ 28.4” |
2 950 | 19° 49’ 15.0” | 100° 36’ 05.8” |
2 850 | 19° 49’ 38.6” | 100° 35’ 35.0” |
Source: Ortiz-Bibian et al. (2017).
Sample collection
When seedlings were 28 months old, 2 g samples of mature needles were collected from 10 trees randomly selected from each of the 14 A. religiosa provenances present in the common garden trial to evaluate the chemical profile. Twelve hours before harvesting, the trial was watered to field capacity. Samples were taken between 8 and 10 h in the morning in October 2016 with northern exposure and no signs of damage. Branches were taken from the middle part of the main stem.
From each sample, 1 g of needles was placed in a paper bag, kept in the drying oven at 80 °C for 24 h and weighed. The other gram was placed in an amber glass bottle containing 10 mL of reagent grade n-hexane, which remained at 5 °C in a refrigerator. Subsequently, the sample in n-hexane was ground in a porcelain mortar with 0.5 g of inert sand and 1 mL of tetradecane (C14H30) to a concentration of 1 mg∙mL-1 as an internal standard. The extract was filtered through a funnel with 11 μm pore opening filter paper containing 0.5 g of reagent grade anhydrous sodium sulfate (Na2SO4) and stored in an amber vial at 5 °C until chemical analysis.
Chemical Analysis
Samples were analyzed by injecting 1 µL into a gas chromatograph equipped with a mass detector (Agilent 6890, MSD 5973), flow split (60.3:1) at 250 °C on a HP-5MS nonpolar capillary column (30 m x 20 mm x 20 µm), using helium as carrier gas with a flow rate of 1 mL∙min-1. The oven temperature program was initial ramp of 50-200 °C at 20 °C∙min-1, followed by another ramp of 200-280 °C at 15 °C∙min-1 and a final ramp of 280-300 °C at 20 °C∙min-1. The mass spectrometer was run in electric ionization mode, flow rate of 1 mL∙min-1, ionization voltage at 70 eV, interface temperature at 280 °C and SCAN mode with a mass range of 50 to 550 m/z (García‐Rodríguez, Torres‐Gurrola, Meléndez‐González, & Espinosa‐García, 2016).
The concentration of compounds was calculated by the internal standard method, relating the area of each compound to the area of the tetradecane, whose concentration is known. Subsequently, the concentration was related to the dry weight of the sample (mg∙g-1). These data were used to calculate the chemical diversity of the provenances.
Spectra obtained on MSD ChemStation (Agilent Technologies Inc., 2004) were compared with those of the NIST/EPA/NIH Mass Spectrometer library, version 2.0 (Stein, Mirokhin, Tchekhovskoi, & Mallard, 2012). The identity of the compounds was validated with experimental retention rates by injecting 1 µL of two series of alkanes (C8-C20 y C21-C40, Sigma Aldrich) with the same chromatographic method. The retention times of the alkanes were related to those of the compounds and compared with retention rates reported in the literature (Adams, 2007; Zenkevich, 2010).
Chromatograms were obtained for each sampled individual which, according to statistical analyses, were divided into three groups with an altitudinal interval of 200 m, so a chromatogram was selected from each altitudinal section to exemplify their phytochemical diversity (Figure 2).
Diversity indices
The alpha diversity, expressed through several diversity indices of the 14 provenances of A. religiosa, was estimated with the statistical analysis program PAST 4.03 (Hammer, Harper, & Ryan, 2001). Eleven diversity indices were obtained, of which the most informative (significant) were selected by ANOVA in the graphical interface of the free software R Rcmdr 4.0.2 (R Development Core Team, 2020). These were Fisher's alpha index and Menhinick's index which proved robust since it allows comparison of samples of different sizes.
Total diversity measured with the Menhinick index was based on the relationship between the total number of terpenes and the total number of trees (samples). Fisher's alpha index was used for the most common terpenes in most conifers (α-pinene, camphene, β-pinene, β-myrcene, 3-carene, limonene), which allows comparison of the level of richness between provenances, which vary in the level of abundance and depend on the number of individuals sampled. In both indices, each terpene was considered as a 'species' and the provenances as 'communities'.
Statistical Analysis
ANOVA and multivariate analyses were carried out using the SAS 9.4 TS 1 M3 statistical package (SAS Institute Inc., 2015). PROC GLM was used for the ANOVA and PROC STEPDISC for the stepwise analysis to select statistically significant (P < 0.05) and informative variables. A correlation analysis was also performed with PROC CORR to remove highly correlated variables and avoid collinearity problems, with the exception except for terpenes commonly found in conifers such as α-pinene, camphene, β-pinene, β-myrcene, 3-carene, limonene (Kopaczyk et al., 2020; Pokorska et al., 2012; Taft et al., 2015). Subsequently, a matrix of averages was obtained for multivariate principal component, correspondence and cluster analyses performed with PRINCOMP, CORRESP and CLUSTER procedures, respectively.
Results
Table 2 shows the 32 compounds and their retention indices (RI) found in the needles of the 14 provenances of A. religiosa: 17 monoterpenes (RI 932-1 132), 10 sesquiterpenes (RI 1 345, 1 375-1 700), one phenyl propanoid (RI 1 196), one alkatetraene (RI 1 237) and three esters (RI 1 223, 1 287, 1 379).
Table 2 Secondary metabolites identified in 14 provenances of Abies religiosa from Cerro San Andrés, Municipio Libre de Hidalgo, Michoacán, Mexico, ordered by retention time and retention index.
RT | RI | RIexp | SM | RT | RI | RIexp | SM |
---|---|---|---|---|---|---|---|
3.45 | 932 | 942 | α-Pinene* | 5.79 | 1 237 | 1 254 | Pulegone |
3.57 | 946 | 959 | Camphene* | 6.1 | 1 287 | 1 298 | Bornyl acetate |
3.74 | 969 | 981 | Sabinene | 6.64 | 1 345 | 1 376 | α-Cubebene |
3.78 | 974 | 987 | β-Pinene* | 6.7 | 1 379 | 1 381 | Geranyl acetate |
3.82 | 988 | 992 | β-Myrcene* | 6.78 | 1 375 | 1 397 | Copaene |
4.02 | 1 002 | 1 019 | α-Phellandrene | 7.1 | 1 417 | 1 446 | Caryophyllene |
4.09 | 1 008 | 1 027 | 3-Carene* | 7.33 | 1 452 | 1 482 | α-Humulene |
4.13 | 1 020 | 1 032 | o-Cymene | 7.49 | 1 458 | 1 506 | Alloaromadendrene |
4.16 | 1 024 | 1 036 | Limonene* | 7.56 | 1 484 | 1 518 | Germacrene D |
4.24 | 1 025 | 1 047 | β-Phellandrene | 7.57 | 1 500 | 1 519 | α-Muurolene |
4.26 | 1 044 | 1 050 | β-Z-Ocimene | 7.69 | 1 522 | 1 539 | δ-Cadinol |
4.39 | 1 054 | 1 096 | γ-Terpinene | 7.77 | 1 574 | 1 552 | Germacrene D-4-ol |
4.62 | 1 086 | 1 096 | Terpinolene | 8.18 | 1 582 | 1 619 | Caryophyllene oxide |
4.93 | 1 132 | 1 137 | (E,E)-Cosmene | 8.41 | 1 645 | 1 656 | Cubenol |
5.46 | 1 196 | 1 209 | Estragole | 8.48 | 1 652 | 1 667 | α-Cadinol |
5.63 | 1 223 | 1 232 | Fenchyl acetate | 8.65 | 1 700 | 1 695 | Eudesm-7(11)-en-4-ol |
RT: retention time, RI: retention index, RIexp: retention index experimental, SM: secondary metabolite. *SM most commonly found in conifers.
Diversity indices
Figure 3 shows that provenances were significantly different from each other (P ≤ 0.0174, α = 0.05), according to the specific richness calculated with the Menhinick index. Simple linear regression reports a negative association between terpene diversity and height above see level (P = 0.0016, R2 = 0.5788); that is, the number of terpenoids decreased as altitude increased. Tukey's mean comparison test showed three groups according to the number of terpenes in each provenance: 1) the 2 850 m provenance, different from the rest and where the highest terpene diversity is found; 2) the provenances between 2 950 and 3 300 m, including the highest altitude (3 540 m), which together present intermediate diversity; and 3) the provenances from 3 350 to 3 500 m, where the lowest terpene diversity is found regarding the distribution range studied.
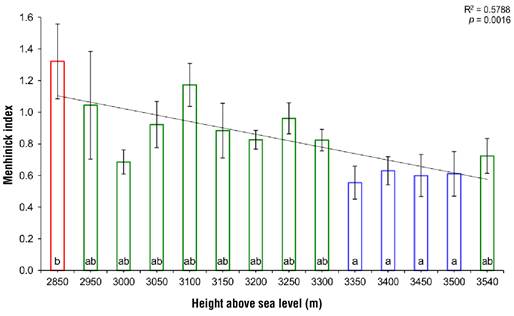
Figure 3 Variation of terpene diversity calculated with Menhinick's index for 14 provenances of Abies religiosa from Cerro San Andrés, Municipio Libre de Hidalgo, Michoacán, Mexico. The same color indicates belonging to a Tukey's mean clustering (P = 0.05).
On the other hand, according to Figure 4, the provenances also showed significant differences among themselves (P ≤ 0.0083), according to Fisher's alpha index. Similar to Menhinick's index, it was shown by simple linear regression that terpenoid diversity decreases as elevation increases (P = 0.0013, R2 = 0.5919). Moreover, Tukey's comparison of means established three sets according to the diversity of terpenoids analyzed: 1) the provenance at 2 850 m having the highest diversity, 2) the provenances between 2 950 to 3 350 m, including the highest altitude provenance (3 540 m) with intermediate diversity, and 3) the provenances between 3 400 to 3 500 m with lower terpenoid diversity.
Multivariate analysis
Significant differences between provenances were found by analysis of variance for sabinene, o-cymene, terpinolene and α-cubebene (P < 0.05) and highly significant differences for (E,E)-cosmene and eudesm-7(11)-en-4-ol (P < 0.0001). Stepwise analysis identified germacrene D, α-cubebene, germacrene D-4-ol and δ-cadinol (P < 0.05) and terpinolene (P < 0.0001) as more informative variables.
In addition to the terpenes selected with ANOVA and stepwise analyses, a correlation analysis was performed, removing terpenes highly correlated with each other, except for those commonly found in conifers. Thus, out of the 32 compounds identified, 22 were selected and principal component, correspondence and cluster analyses were performed. According to the principal component and correspondence analyses, three discrete groups were detected corresponding to altitudinal ranges of 200 m: 2 850 to 3 050 m, 3 100 to 3 300 m and 3 350 a 3 540 m.
Principal component analysis
The first five principal components had eigenvalues greater than 1.0 and explain 90 % of the total variance. The first component had an eigenvalue of 7.4 explaining 33.7 % of the total variance. Terpenes such as α-pinene, β-pinene, δ-cadinol and cubenol contributed strongly to the variance of this component, with eigenvectors greater than 0.3. The second component had an eigenvalue of 4.13 explaining 18.8 % of the total variation. The terpenes that contributed significantly to the variation were o-cymene, (E,E)-cosmene, pulegone and eudesm-7(11)-en-4-ol. The third component had an eigenvalue of 3.6 contributing 16.3 % of the total variance; the terpenes that had eigenvectors greater than 0.3 were limonene, β-phellandrene and eudesm-7(11)-en-4-ol. The fourth component had an eigenvalue of 2.85 which contributed 12.9 % of the variance; terpenes that had eigenvectors greater than 0.3 were camphene, sabinene and terpinolene. Finally, the fifth component had an eigenvalue of 1.88 contributing 8.5 % of the total variance; the terpenes α-phellandrene, germacrene D and cubenol contributed to this variation. Finally, the fifth component had an eigenvalue of 1.88 that contributed 8.5 % of the total variance; the terpenes α-phellandrene, germacrene D and cubenol contributed to this variation.
Figure 5 shows the distribution of the 14 provenances of A. religiosa according to the first three principal components (introduced with the prefix CP), which account for 68.6 % of the total variance explained. Populations below 3 300 m (green squares and red triangles) are more closely related to each other than to populations above that altitude (blue circles), according to the terpenes that define the first three components.
Correspondence analysis
The correspondence analysis showed a significant P-value (<0.0001) by means of the Ӽ2 statistic, where the sum of the first two dimensions is 61.4 % of the total variance explained. Figure 6 indicates the distribution of the colored provenances according to the altitudinal group they were assigned to against the first two dimensions yielded by the analysis. The graph shows that low and intermediate altitude sources are in the first quadrant (upper right quarter) and only two altitudes in the second (lower right quarter), which are associated with four of the compounds frequently found in conifers: β-pinene, limonene, camphene and 3-carene; terpenes that serve mostly for protection against pathogens. Higher altitude provenances are distributed in the second, third (lower left) and fourth quadrants (upper left) of the graph.
Cluster analysis
Figure 7 shows the dendrogram identified with cluster analysis according to Ward's clustering method, which statistically marks a cutoff at 0.1592 of semipartial R2 based on the pseudo t2 statistic. The dendrogram reflects an altitudinal clinal pattern; that is, the diversity and concentration of terpenes decrease as altitude increases. There are three groups formed: i) high altitude provenances (3 500, 3 450 and 3 350 m), ii) higher altitude provenance (3 540 m) and iii) low to intermediate altitude provenances (2 850 to 3 300 and 3 400 m).
Discussion
The needles of the 14 provenances of A. religiosa had 32 compounds, mostly terpenes, specifically monoterpenes. In conifer-dominated sites, monoterpenes are the major compounds in the emission of biogenic volatile organics (Iñíguez et al., 2014; Trowbridge et al., 2021). These metabolites play an important role in tree-insect interactions, because they are a central element of constitutive and inducible defenses of conifers and are an essential component of defensive resins as they are toxic to many herbivores (Pezet et al., 2013; Whitehill et al., 2019); oleoresin secretion is the main form of chemical and mechanical defense (Heredia-Bobadilla et al., 2014).
Several studies have shown that altitudinal variation and chemical diversity of chemical compounds are closely related. Mullin et al. (2021) determined that concentrations of monoterpenes (limonene and 3-carene) and diterpenes increase as elevation increases in populations of Pinus contorta Douglas ex Loudon (Lodgepole pine) (western Alberta, Canada). In contrast, Večeřová et al. (2021) found that, in Picea abies (L.) Karsten (spruce), the content of β-pinene, α-pinene, sabinene, and α-terpineol decreased substantially at higher altitude, and that the only positively associated monoterpene was 3-carene.
The indices in the present study indicate greater terpene diversity in the provenance at lower altitudes (2 850 m) and that as elevation increases, diversity decreases. This is probably because in the lower part of the species distribution, plants are more susceptible to pathogen attack (Rasmann, Pellissier, Defossez, Jactel, & Kunstler, 2014; Rodríguez‐Castañeda et al., 2010); Moreira et al. (2014) indicate that altitude is a biotic driver of plant defense evolution. In this regard, several studies have shown that the incidence of herbivore damage, particularly bark beetles in conifers, is higher at lower altitudes when comparing both intraspecific and interspecific variability. For example, in intraspecific variability there is the case of the bark beetle Ips confusus Wood & Bright. that attacks Pinus edulis Engelm. (pinyon pine) (Santos & Whitham, 2010); in the interspecific, P. contorta is found on lower slopes and Pinus albicaulis Engelm (whitebark pine) on higher slopes, both continuously exposed to the complex formed by bark beetles and fungi, although in the past P. albicaulis was intermittently exposed. In this ecosystem, chemical defense syndromes associated with pine monoterpenes are related to exposure history and thus elevation (Raffa et al., 2017).
Consistent with the diversity indices, principal component and correspondence analyses showed that terpene diversity is higher in provenances below 3 350 m and that they are mostly associated with limonene or 3-carene, which act as repellents for herbivore attack (Phillips & Croteau, 1999). Terpinolene, identified as part of a genotype of Picea sitchensis (Bong.) Carr. (Sitka spruce), confers resistance to white weevil (Pissodes strobi Peck) attack (Robert et al., 2010) and β-pinene found in Pinus ponderosa Douglas ex Lawson is synthesized in response to herbivory activity (Litvak & Monson, 1998).
On the other hand, the cluster analysis suggests association among provenances, except for group two, formed by the highest altitude provenance (3 550 m), which could have greater similarity with the populations of the lower part. This apparently atypical behavior of the provenance near the peak is possible because it receives pollen from the Michoacán slope on the west side of Cerro San Andrés and from the east side (Estado de México). This is consistent with patterns observed for species of the genus Betula, where terpenes have a dominant maternal heritability component, but with influence of pollen (Isidorov Stocki, & Vetchinikova, 2019). Furthermore, this atypical behavior of higher altitudinal provenances has been observed in Pinus hartwegii Lindl. at the peak of Pico de Tancítaro, for the composition of isozyme allele frequencies (Viveros-Viveros et al., 2010). The altitudinal clinal pattern for the diversity and concentration of the terpenes found is similar to that of Pinus patula Schiede ex Schltdl. & Cham. and its monoterpenes α-pinene, Δ-3-carene, β-phellandrene and limonene (Lockhart, 1990). Furthermore, the concentration of sesquiterpenes such as geranyl acetate and δ-cadinol decreased as altitude increased in Pinus yunnanensis Franch. (Yunnan pine, China) (Hengxiao et al., 1999).
It is important to consider that the results of the present study come from a common garden trial under optimal conditions for plant growth. Therefore, it is possible that terpene abundance could vary if the trial had been conducted under field conditions in the distribution area of A. religiosa, as a result of an interaction between genotype and environment; however, because all provenances grew under the same conditions, it is reasonable to assume that terpene diversity varied among populations as a consequence of genetic differences. Clinal patterns have been detected for height, diameter, and survival of this species (Castellanos-Acuña, Lindig-Cisneros, Silva-Farias, & Sáenz-Romero, 2014; Ortiz-Bibian et al., 2017; Ortiz-Bibian et al., 2019), meaning that altitudinal variation should be considered in the management and conservation of forest species populations.
Conclusions
Diversity and concentration of terpenes in Abies religiosa varied according to the altitude of populations; above 3 350 m, diversity was lower, but with higher concentration. The higher terpene diversity at lower altitudes, according to several studies, is a consequence of evolutionary pressures caused by herbivory. Both the diversity indices and the cluster analysis demonstrate the existence of three defined groups; that is, three differentiated chemical profiles that should be considered in the establishment of germplasm collection programs for reforestation and restoration, especially to adapt to the expected effects of climate change.