Highlights:
Nitrogen reservoirs were quantified in the Jocotitlán and Tláloc mountains, Estado de México.
Soil ammonium and nitrate concentrations varied temporally, but total inorganic N was constant.
Nitrogen resorption in high mountain forests is higher than 50 %.
Soil ammonium concentrations varied in pulses according to soil moisture.
Introduction
Nitrogen (N) deficiency limits the productivity of forest ecosystems (Johnson & Turner, 2014). Tree species ensure nutrient availability by strategies such as atmospheric N fixation using bacteria, symbiotic associations with mycorrhizae, uptake of organic forms of N, and high rates of N resorption (Cronan, 2018; Zhang, Yuan, Liu, & Yin, 2019). The two main forms of inorganic (absorbable) N in soil solution are ammonium (NH4 +) and nitrate (NO3 -), and their availability depends on the mineralization of soil organic compounds. Ammonium and nitrate concentrations change seasonally due to factors such as plant uptake, immobilization by microorganisms, and leaching; however, these variations are related to the potential and rate of mineralization of the element in the soil (Chen et al., 2019). There is a general correlation between gross soil mineralization and total N concentration, which means that its status in vegetation is largely defined by the magnitude of soil N reservoirs (Booth, Stark, & Rastetter, 2005). It has been proposed that, near the maximum elevation limit where trees can be found, forest species tend to increase N concentrations in leaf tissue, either to maintain the rate of photosynthesis (even if net growth is restricted by temperature) or to ensure the supply of the nutrient and compensate for the disadvantage of short growing seasons (Liu et al., 2020).
In high mountain forests (4 750 m), N acquisition varies with season, and, in fact, there may be seasonal preference in the uptake of ammonium, nitrate, and organic forms (Hong, Qin, Ma, Xu, & Wang, 2019; Zhang et al., 2020). On the other hand, as atmospheric CO2 concentrations increase, there may be a stimulus in tree growth that will be sustained as long as N supply does not restrict the productivity of forest species (Norby & Zak, 2011). Therefore, the study of the available forms of N in the soil and its resorption by vegetation is relevant information for the study of the functioning of high mountain forests in relation to changes in chemical composition of the atmosphere (Silva, Gómez‐Guerrero, Doane, & Horwath, 2015).
The influence of N on ecosystem productivity is physiologically explained by its close relationship with the enzyme rubisco, responsible for carbon fixation (Schlesinger & Bernhardt, 2020). Seasonal changes of N in foliage and stoichiometry with respect to other nutrients determine the rate of decomposition of organic residues and, together with microorganisms and climate, influence the availability and circulation of inorganic soil N forms (Reed, Townsend, Davidson, & Cleveland, 2012).
The objective of this study was to quantify N reservoirs in forest litter, topsoil (0-10 cm) and needle litterfall, and to measure temporal soil nitrate (NO3 -) and ammonium (NH4 +) concentrations and N resorption (ReabsN) in the Jocotitlán (JO) and Tláloc (TLA) mountains of central Mexico. The hypotheses were that (1) the forest with higher total N reservoirs in forest litter and soil has higher soil concentrations of nitrate and ammonium, (2) ReabsN levels are similar in the two mountains, and (3) nitrate and ammonium concentrations follow seasonal pulses. The above, considering that, in high mountain forests, leaf N concentrations are determined by the availability of soil N forms, climate and capacity of trees to reuse nutrients.
Materials and methods
Study area
The study area included two mountainous elevations in Estado de México: Jocotitlán (JO), located between the municipalities of Atlacomulco and Jocotitlán, and Tláloc (TL) in the municipality of Texcoco. These mountains are part of the Transmexican Volcanic Belt, geologically composed of an andesite-dacite complex and pyroclastic deposits (Arce et al., 2019). The climate is type C(w2)(w), semi-humid semi-cold with summer rainfall and less than 5 % winter rainfall with precipitation/temperature ratio higher than 55 (Garcia, 2004). Mean annual precipitation ranges from 900 to 1 200 mm and mean annual temperature ranges between 5 and 10 °C. Both mountains have forests of Pinus hartwegii Lindl., on volcanic soils (Andisols). Nutrient dynamics, radial growth, water use efficiency and wood density in both mountains can be found in the literature (Correa‐Díaz et al., 2019, 2020; Correa-Díaz, Gómez-Guerrero, Vargas-Hernández, Rozenberg, & Horwath, 2020; Morgado-González et al., 2019; Núñez-García, Gómez-Guerrero, Terrazas-Salgado, Vargas-Hernández, & Villanueva-Díaz, 2021; Torres-Duque, Gómez-Guerrero, Trejo-Téllez, Reyes-Hernández, & Correa-Díaz, 2022).
Observation sites
Four observation sites located in pure stands of P. hartwegii were established on each mountain to collect needle litterfall, forest litter, and mineral soil. To capture variation in the mountains, observation sites covered elevations from 3 500 to 3 900 m and northwest and southwest exposures (Table 1). These observation sites were also used for a more detailed study on stoichiometry of needle litterfall by Torres-Duque et al. (2022).
Table 1 Sites used in the periodic collection of Pinus hartwegii needle litterfall in the mountains of Jocotitlán y Tláloc, Estado de México.
Mountain | Site | Elevation (m) | Aspect | Latitude (°) | Longitude (°) |
---|---|---|---|---|---|
Jocotitlán | NO-3800 | 3 800 | Northwest | 19.7423 | -99.7645 |
SO-3800 | 3 800 | Southwest | 19.7412 | -99.7662 | |
NO-3700 | 3 700 | Northwest | 19.7427 | -99.7610 | |
SO-3700 | 3 700 | Southwest | 19.7397 | -99.7680 | |
Tláloc | NO-3900 | 3 900 | Northwest | 19.4240 | -98.7245 |
NO-3500 | 3 500 | Northwest | 19.4161 | -98.7258 | |
SO-3900 | 3 900 | Southwest | 19.7255 | -98.7255 | |
SO-3500 | 3 500 | Southwest | 19.4045 | -98.7419 |
Needle samples
In June 2017, 12 green foliage samples of adult trees were taken on each mountain to estimate mean total N content. To quantify N mass in needle litterfall, a 20 m transect was established on the contour line, with three equidistant traps (10 m apart) of 30 cm diameter, located at ground level and delimited with 127 mm thick polyduct, as explained by Torres-Duque et al. (2022). A total of 12 traps were established on each mountain (three replicates for each of the four collection sites)
Needle litterfall samples were collected in 9 (JO) and 10 (TL) visits for one year (June 2017- June 2018). The needles were dried in forced circulation air oven (Sheldon Fx14-S, USA) at 70 °C for 72 h and weighed using an Ohaus digital scale (Scout Pro, USA) with 0.01 g accuracy. The values of needle mass were expressed in kg∙ha-1.
Gravimetric soil water content and N resorption
Gravimetric soil water content was determined using a forced circulation stove at 105 °C for 12 h until constant weight (Rowell, 2014).
ReabsN was estimated by considering the mean value of total N concentration in one- and two-year-old green needles, still active in the tree, and of recently needle litterfall that, due to their senescent stage, had already fulfilled their function in the canopy. The equation ReabsN (%) = [(Nv - Nscn ) / Nv]*100 (Kobe, Lepczyk, & Iyer, 2005); where, Nv and Nscn are the N concentrations in green and senescent needles, respectively. The mean value of N in green needles was 1.7 %, which is similar to that reported for P. hartwegii by Ramírez-Contreras y Rodríguez-Trejo (2009).
Nitrogen in forest litter, soil and needles
At the beginning of the experiment, three forest litter samples were taken at each site (12 per mountain) and analyzed for N concentration. In this study, the term forest litter or forest floor refers to all the organic material above the mineral soil and with different degree of decomposition; that is, the O horizon. The N concentration of the mineral topsoil (0-10 cm), which corresponded to the A horizon, was determined in 36 samples at JO and 40 at TL during the year of study. Once dry, samples of forest litter, green foliage and needle litterfall were ground and analyzed for N concentration using the semi-micro-Kjeldahl method (Bremmer, 1965).
Inorganic nitrogen
A total of ten g of soil were weighed and the inorganic N was extracted with 100 mL of a 2 M KCl solution. The sample in solution was filtered using funnels and 150 mm diameter Whatman No. 1 filter paper. The filtrate was stored at -5 °C until analysis. Inorganic N was determined using the micro-Kjeldahl method, by steam distillation as indicated in method AS-08 established in NOM-021-SEMARNAT-2000 (Secretaría de Medio Ambiente y Recursos Naturales, 2000).
Statistical analysis
Mass (kg∙ha-1) and N flux (kg∙ha-1∙yr-1), ReabsN (%) and inorganic forms NO3 - and NH4 + (mg∙kg-1) were the response variables for comparison between mountains. Results between the two mountains were compared using the t-test. Data was checked for compliance with the assumption of normality and when this was not the case the nonparametric Wilcoxon test was used (Zar, 2010).
Time trends were analyzed with linear mixed models to perform a longitudinal variance analysis considering time, mountain and time*mountain interaction as fixed factors (Fitzmaurice, Laird, & Ware, 2004). This procedure finds that the measurements are correlated over time and the statistical analysis focuses on the temporal trends of each group studied. We tested whether temporal trends followed a quadratic or cubic pattern (P < 0.05). Analyses were carried out with the statistical packages, SAS (Statistical Analysis System, 2011) and R (3.6.3) (R Core Team, 2020).
Results and Discussion
Mass and N inputs in topsoil
The mass of N in JO forest litter was about 2.5 times higher than that of TL (360 vs. 136 kg∙ha-1), but including the first 10 cm of mineral soil, N mass was similar in both mountains (3 278 vs. 3 026 kg∙ha-1) (Table 2). This result denotes that the organic horizon and the first 10 cm of soil are an important reservoir of N in both mountains, although the residence time of N in the organic horizon (O horizon) was higher in JO compared to TL. This was corroborated using the reservoir and N flux data in forest litter, assuming equilibrium conditions, which was congruent with a longer residence time in JO (Table 2).
N content in mineral soil, in some cases, is related to differences in soil texture, since as the fine fraction (silt and clay) increases, so does the capacity to retain organic matter and, therefore, nitrogen. However, in this study, soil texture in both mountains is similar (Marín, Escolero-Fuentes, & Trinidad-Santos, 2002), so it is more likely that the N components in Table 2 reflect the chemical composition and decomposition rate of the forest floor organic matter (Thomas & Prescott, 2000), because N input via needles is higher in JO. Also, the efficiency of N use in needles is consistent with this explanation; 1 kg of N corresponded to a productivity of 115 and 205 kg of needles for JO and TL, respectively.
Table 2 Components of N dynamics in Pinus hartwegii forests in the mountains under study.
Mountain | Forest litter (kg N∙ha-1) | Mineral soil (0-10 cm) (kg N∙ha-1) | Forest floor + topsoil (kg N∙ha-1) | Needle litterfall (kg N∙ha-1∙año-1) | Use efficiency (kg needle∙kg-1 N) | Residence of N in forest floor (year) |
---|---|---|---|---|---|---|
Jocotitlán | 360.6 | 3 278 | 3 642.6 | 119.5 | 115 | 3 |
Tláloc | 136.7 | 3 026 | 3 166.7 | 67.4 | 205 | 2 |
Soil moisture, reservoirs and N resorption
Mean values of gravimetric water content and percentages of total N, ammonium and nitrate of topsoil were statistically similar (P > 0.05) in both mountains. However, when considering total inorganic N (ammonium + nitrate), the mean was higher in JO (Table 3). This result is explained by the larger N reservoirs in topsoil mass; however, it is important to mention that even with a larger N reservoir in JO, N resorption was higher in TL; that is, in TL, trees used leaf N more efficiently.
Although soil inorganic N may be an indirect indicator of soil N mineralization potential (Booth et al., 2005; Thomas & Prescott, 2000), N uptake by trees depends on N immobilization by soil microorganisms and leaching (Chen et al., 2019). Although the above processes were not measured in this study, N reservoirs are large in both mountains, since, in many forest ecosystems, forest litter reservoirs summed with soil profile vary between 2 and 6 Mg (Johnson & Turner, 2014). In this study, including forest litter and the first 10 cm of mineral soil, 3 Mg N was exceeded (Table 2). With such adequate sample amounts, it is very likely that annual N fluxes are higher than the demand by soil microorganisms and plant uptake (Booth et al., 2005).
N resorption (ReabsN) values ranged from 55 to 66 % (Table 3) and are high compared to estimates between 30 and 39 % for Pinus montezumae Lamb. (Lopez-Escobar et al., 2018); however, values are low compared to 88 % in oak species (Chávez-Vergara, González-Rodríguez, Etchevers, Oyama, & García-Oliva, 2015). In Pinus pinaster Ait, ReabsN ranged between 50 and 65 % with lower values in regions with lower extreme temperatures (González-Zurdo, Escudero, & Mediavilla, 2015). ReabsN in this study was similar to that found for Larix kaempferi (Lamb.) Carrière and Cryptomeria japonica D. Don with values of 63 and 57 %, respectively (Enta et al., 2019). Worldwide, forest plantations show ReabsN values of 59 % (Jiang et al., 2019), but the variation ranges from 30 to 70 % for natural coniferous forests (He et al., 2020).
Differences in ReabsN may be related to soil fertility; in poorer soils, ReabsN tends to be higher (Kobe et al., 2005). However, the results of this study are not explained by differences in soil fertility, since Torres-Duque et al. (2022) found a higher sum of exchangeable bases (Ca+2, K+ y Mg+2) in TL topsoil than in JO. Probably, no trend is shown (Tables 2 and 3) due to the high concentrations of total N and net soil N mass in both mountains. The difference in ReabsN could rather be related to the level of productivity of each site. The fact that TL is more productive (Correa-Díaz et al., 2020) is possibly related to higher N resorption by plant life.
Table 3 Mean soil moisture values and nitrogen compartments in topsoil (0-10 cm) in two Pinus hartwegii forests.
Mountain | Gravimetric water content (%) | Total nitrogen (%) | Nitrate (mg∙kg-1) | Ammonium (mg∙kg-1) | Nitrate + ammonium (mg∙kg-1) | N resorption (%) |
---|---|---|---|---|---|---|
Jocotitlán | ||||||
Mean | 63.1 | 0.44 | 30.1 | 16 | 46.1 | 55.8 |
Standard deviation | 32 | 0.18 | 4.3 | 8 | 6.7 | 8.2 |
CV (%) | 50.7 | 42.1 | 14.2 | 50 | 14.5 | 14.7 |
n | 36 | 36 | 36 | 36 | 36 | 36 |
Tláloc | ||||||
Mean | 53.5 | 0.4 | 27.7 | 14.1 | 41.7 | 60 |
Standard deviation | 23.3 | 0.13 | 8.4 | 6.1 | 11.3 | 5.9 |
CV (%) | 43.6 | 32.8 | 30.3 | 43.7 | 27.2 | 9.8 |
n | 40 | 40 | 40 | 40 | 40 | 40 |
P ≤ 0.05 | 0.3187 | 0.4953 | 0.1526 | 0.4082 | 0.0441 | 0.0397 |
Wilcoxon test for gravimetric water content and ammonium. T-test for the rest of the variables.
Soil inorganic nitrogen
Mean values of inorganic N were higher (Table 3) than those reported in the topsoil of some montane forests (1 500 to 2 500 m elevation) with concentrations of 11.3 and 27.6 mg∙kg-1 of ammonium and nitrate, respectively (Zhang et al., 2020). Inorganic N concentrations depend on factors such as temperature, soil moisture, and organic substrate quality. In pine plantations with low precipitation (450 mm) and sandy soils, inorganic N concentrations can be as low as 3 mg∙kg-1 (Zhan-Yuan, Fu-Sheng, De-Hui, Qiong, & Guang-Sheng, 2008). The study area with precipitation between 900 and 1 200 mm and medium to loamy textured soils reported the expected results.
Higher amounts of nitrate relative to ammonium are common in ecosystems with large N reservoirs and highly dynamic nutrient cycling (Rothstein, 2009), which is also supported by low canopy residence times (Table 1); moreover, with short periods of soil moisture saturation, during summer rains, ammonium accumulation is less common.
The N mass of forest floor needles plus N mass in topsoil (0-10 cm) (Table 1) is higher than that reported by Fonseca and Figueiredo (2018) in coniferous forests, where reservoirs of 1 371 to 2 693 kg N∙ha-1 were found. This difference could be because the high mountain forest studied (3 500 - 3 900 m elevation) is more productive in needles, compared to the ecosystems of those authors. Thomas and Prescott (2000) found amounts close to 1 300 kg N∙ha-1 in coniferous forests, including forest litter and the first 12 cm of mineral soil, which is also a lower amount than that found in this study.
Temporal trends of inorganic N
Environmental conditions and chemical composition of needle litterfall are similar between mountains (Torres-Duque et al., 2022), so similar temporal trends of inorganic N would also have been expected. Only ammonium showed a pattern with a cubic trend (Figure 1). Ammonium accumulation seems to have two maxima: one in autumn (October) and one in summer (June), but with a more marked pulse in JO. Soil moisture partially explains this trend; in October, the topsoil still has gravimetric water content close to 75 %, reaching a minimum in March and recovering moisture with the beginning of the rainy season in June (Figure 2); however, it should also be considered that temperatures are lower in autumn than in summer and for that reason the second maximum is higher, especially in JO. The temporal variation of inorganic N is also regulated by tree uptake (Dittman, Driscoll, Groffman, & Fahey, 2007), so it is likely that trees preferentially absorb nitrate during the second maximum, which corresponds to the growing season (Hong et al., 2019; Zhang et al., 2020).
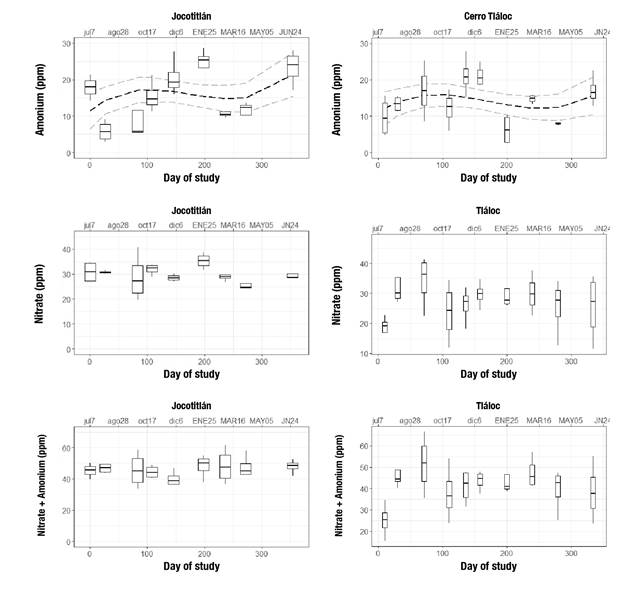
Figure 1 Temporal trend (June 2017-June 2018) of inorganic nitrogen forms in Pinus hartwegii forests. Only the temporal trend for ammonium is plotted, the only case in which the variation was statistically significant according to a cubic trend (P < 0.05).
Temporal trend of leaf N resorption (ReabsN)
The temporal trend of ReabsN showed its minimum with the end of the growing season (October) as autumn arrived, and the highest uptake was recorded near the beginning of the growing season (April) in spring (Figure 3). This result denotes a parallel pulse with the growth rate of the tree stand, reusing more leaf N as the growth rate is established; it is also correlated with moisture and temperature. By June, in both mountains, the rainy period is already established, and temperatures are approximately 2 °C lower than March-April (Correa-Díaz et al., 2020), favoring new foliage expansion and N reuse (Jiang et al., 2019). In general, variations of inorganic N ranged from 40 to 60 mg∙kg-1 that are common in forest ecosystems (Verchot, Holmes, Mulon, Groffman, & Lovett, 2001).

Figure 3 Temporal trend (June 2017-June 2018) of leaf nitrogen resorption in Pinus hartwegii forests. Variation was significant according to a cubic trend (P < 0.05).
Based on the results of this study, the hypotheses proposed are supported in different ways. With respect to the first hypothesis, it is confirmed that inorganic N concentrations are higher in soils with larger N reservoirs. Regarding the second hypothesis about similar N resorption levels in the two mountains, the hypothesis is not supported; the TL forest with less N reservoirs showed higher resorption, possibly as a result of higher nutrient limitation. With respect to seasonal pulses of inorganic forms of N, the hypothesis is partially supported, since only ammonium showed them.
Conclusions
This study confirms that it is possible to identify differences in functioning between forests based on N dynamics. The Jocotitlán mountain, with higher N reservoirs, also reflected higher total inorganic N (ammonium + nitrate). Leaf N resorption was higher in Tláloc mountain, where the mass of total N in topsoil was lower. N resorption in needles and inorganic forms of soil N were useful to understand N dynamics in high mountain forests. N components in forests provide critical information on their functioning and are relevant to characterize the response of these ecosystems to climate change.