Introduction
Forest plantations play an important role in meeting the world's needs for fiber and wood (Schimleck, Antony, Dahlen, & Moore, 2018). Therefore, breeding programs focus on priority species to increase productivity (McKeand, Mullin, Byram, & White, 2003) using intensive silviculture practices. Productivity results from the balance between physiological growth potential (photosynthetic activity and water and nutrient uptake capacity) and space-related growth constraints (competition with other individuals and limited resource availability) (Álvarez-Taboada, Barrio-Anta, Gorgoso-Varela, & Álvarez-González, 2003).
The initial spacing of trees influences the scheduling of silvicultural treatments (Hébert et al., 2016). The ideal combination of growth space with rotation age, site quality and silvicultural treatments, particularly pruning and thinning, favors wood properties (Guerra-Bugueño, Célis-Mosqueira, & Moreno-García, 2014; Hébert et al., 2016). Spacing influences total volume, stem shape, growth rings, juvenile wood, and branch length and diameter (Macdonald & Hubert, 2002).
Climate is a determining factor in growth ring characteristics (Haghshenas et al., 2016). Several studies relate radial growth rate and ring characteristics to environmental variables such as temperature, rainfall, and light intensity (García-Suárez, Butler, & Baillie, 2009), as well as changes in ring characteristics to altitudinal gradients (Hernández & Castellanos, 2006). For example, rainfall during winter and early spring greatly influences radial growth of Pinus halepensis Mill., especially earlywood formation (Olivar, Bogino, Spiecker, & Bravo, 2012; Olivar, Rathgeber, Ordóñez & Bravo, 2013). In Pinus patula Schltdl. & Cham. it has been shown that populations located at a higher elevation are less productive than those at a lower elevation; however, the opposite may also be true if lower elevation populations are at the lowest limit of the species' natural distribution range (Salazar et al., 1999). Because of this, the width of growth rings and their components (early and latewood) are important and reliable variables for assessing the effect of the environment and growing space on productivity and wood quality (Zhu, Scott, Scallon, & Myers, 2007). Knowledge of this relationship facilitates the study of wood properties and allows separating the effect of age associated with the physiological processes of trees, with respect to the effect of the site’s environmental factors.
The overall objective of this study was to evaluate the radial growth of P. patula in two plantations with different ages and thinning intensity, as well as its relationship with interannual variation in temperature and rainfall. The specific objectives were a) to evaluate the effect of growing space on tree radial growth; b) to evaluate the effect of plantation age on tree response to growing space; and c) to estimate the correlation between radial tree growth and temperature and rainfall conditions during the year of ring formation.
Materials and methods
Study area
The study was conducted in two P. patula plantations in the community of Ixtlán de Juárez, Oaxaca. The first one was established in 1995, at an elevation of 2 538 m (17° 22' 38.3" N and 96° 28' 43.2" W), with 1.5 x 1.5 m initial spacing (4 444 trees·ha-1). The second one was established in 2005, at an elevation of 2 877 m (17˚ 40' 17.6" N and 96˚ 46' 05.3" W), with 2.5 x 2.5 m spacing (1 600 trees·ha-1).
At each plantation, 10 trees were evaluated from each of three 400 m2 (20 x 20 m) plots, which included two thinned and one unthinned plot. The 1995 plantation was thinned in 2004, 2008 and 2017, in accordance with the management plan established by the forestry technical service provider in the community. In the third thinning (2017), 20 % (plot 1) and 28 % (plot 2) of trees per hectare were removed. Thinning in the 2005 plantation was done in 2016, removing 16 % (plot 1) and 26 % (plot 2) of trees, according to the established management plan. The estimated density of these plots, before thinning, was 1 400 trees·ha-1 (plot 1) and 950 trees·ha-1 (plot 2). In 2018, diameter at breast height and total height measurements were made, and basal area and total volume of the plots in each plantation were estimated (Table 1).
Table 1 Mensuration characteristics of Pinus patula trees in 13- and 23-year-old plantations.
Plantation | Variable | Plot 1 (slight thinning) | Plot 2 (moderate thinning) | Plot 3 (no thinning) |
---|---|---|---|---|
1995 (23 years) | NT (trees·ha-1) | 950 | 825 | 2 475 |
mDBH (cm) | 24.20 | 27.20 | 19.73 | |
BA (m2·ha-1) | 46.11 | 58.51 | 30.98 | |
VOL (m3·ha-1) | 107.81 | 131.88 | 68.30 | |
2005 (13 years) | NT (trees·ha-1) | 1 175 | 700 | 1 550 |
mDBH (cm) | 14.95 | 16.85 | 13.65 | |
BA (m2·ha-1) | 18.41 | 23.44 | 15.34 | |
VOL (m3·ha-1) | 42.35 | 44.50 | 39.65 |
NT = number of trees, mDBH = mean diameter at breast height, BA = basal area; VOL = total volume.
Tree distribution and sampling
A distribution plan of trees in each plot was drawn up. Each plot was divided into 10 x 10 m quadrants; subsequently, angles and distances were recorded for each individual, taking as a reference one dominant tree per quadrant. The data were recorded in an Excel 2013 sheet, and imported by the Quantum GIS 2.18 software (QGIS Development Team, 2002) to create the distribution plan.
Based on the spatial distribution of trees per plot, 10 trees representative of the average level of competition within the plots were selected. In order to obtain a greater number of growth rings, the wood sample (chip) from each tree was extracted at 80 cm from the ground. The sample was taken perpendicular to the slope, with a 5 mm diameter Pressler® auger, in the spring of 2018 (the last ring of the sample was formed in 2017). The samples were transported in plastic straws as a means of protection, stored in a cool environment, mounted on wooden bases, and polished with coarse grit sandpaper (60 and 100) and extra-fine grit sandpaper (1200) to see the growth rings in detail.
Measurement of neighboring trees
On the same field sampling date, the four neighboring trees closest to each selected tree (10 trees per plot) were measured to estimate the individual and per-plot competition index. Quadrants were established for each selected tree following the cardinal orientation (N, E, S and W). In each quadrant the nearest tree was identified; diameter at breast height, total height and distance to the selected tree were measured. With these data the competition index (CIi) for each sampled tree was calculated with the following formula (Daniels, Burkhart, & Clason, 1986):
where,
|
diameter at breast height of the j-th neighboring tree |
|
distance from j-th neighboring tree to the i-th sampled tree. |
The
Sample processing
Wood samples (cores) from each tree were scanned and the images were processed with the winDENDROTM software (Regent Instruments Inc., 2008). In each identified image, the growth rings were counted, taking as a reference the ring formed in 2017. Once the year of formation of each ring was identified, total ring width (RW, mm) was measured, as well as earlywood width (EWW) and latewood width (LWW). Latewood proportion (LWP) was estimated with the equation:
The annual basal area increment (BAI) was determined with RW. For this, the accumulated growth in diameter and basal area was calculated, and the BAI was obtained by subtracting the value of the previous year.
Statistical analysis
An analysis of variance was carried out with the SAS 9.3 GLM procedure (SAS Institute, 2004), using relative values of the ring characteristics. Relative values were obtained by adjusting the original values, based on the average value of the characteristic in each tree and plot, to reduce the effect of growth rate or size among trees within each plot. The equation used to obtain relative values was:
where,
Xijr |
relative value in the i-th ring of the j-th tree. |
Xij |
absolute value in the i-th ring of the j-th tree. |
| |
|
average value of plot. |
The radial growth of the plantations and its relationship with climatic conditions (temperature and rainfall) in the year of ring formation was compared using the following procedure: first the effect of cambial age on RW and EWW was eliminated using a negative exponential regression model; then, the average value of the variable in the plantation was added to the residuals of this model to return the values to the original scale; and then, with the adjusted values of RW and EWW, the LWW and LWP in each ring were estimated by difference. Finally, with the adjusted values, an analysis of variance was made to evaluate the differences between plantations in the characteristics of rings formed from 2008 to 2017, using the MIXED procedure in SAS (SAS Institute, 2004).
The relationship between growth ring characteristics and temperature and rainfall, during the year of wood formation, was compared using a correlation analysis between the RW, EWW and LWW variables and the average annual mean, maximum and minimum temperature, and annual rainfall estimated for each plantation site. Climate data were estimated with the "Climate NA v5.00" software model (Wang, Hamman, Spittlehouse, & Carroll, 2016). The 1999-2016 time series was used in the analysis for the 1995 plantation, and the 2009-2016 time series was used for the 2005 plantation. These series include all the growth rings available in the samples from the two plantations.
Results and discussion
Effect of growing space on ring characteristics
The analysis of variance with relative values allowed detecting more variables with significant differences (P ≤ 0.10) between the P. patula plots. In the 1995 plantation, differences were found in BAI in 2011, in LWW in 2006 and 2008, and in LWP in 2008 and 2017. In contrast, the 2005 plantation did have differences in the five characteristics evaluated and in a greater number of years, as shown in Table 2.
Table 2 Analysis of variance (P values) of growth ring characteristics in Pinus patula trees in three plots (with light, moderate and no thinning) of 13- and 23-year-old plantations.
Year of formation | 1995 Plantation (23 years) | 2005 Plantation (13 years) | |||||||||
---|---|---|---|---|---|---|---|---|---|---|---|
RW | BAI | EWW | LWW | LWP | RW | BAI | EWW | LWW | LWP | ||
1999 | 0.710 | 0.704 | 0.555 | 0.887 | 0.871 | ||||||
2000 | 0.894 | 0.819 | 0.986 | 0.702 | 0.746 | ||||||
2001 | 0.916 | 0.711 | 0.498 | 0.289 | 0.235 | ||||||
2002 | 0.222 | 0.952 | 0.436 | 0.411 | 0.883 | ||||||
2003 | 0.395 | 0.996 | 0.336 | 0.498 | 0.284 | ||||||
2004 | 0.677 | 0.885 | 0.637 | 0.848 | 0.881 | ||||||
2005 | 0.596 | 0.938 | 0.737 | 0.445 | 0.713 | ||||||
2006 | 0.900 | 0.920 | 0.629 | 0.096* | 0.303 | ||||||
2007 | 0.813 | 0.855 | 0.460 | 0.656 | 0.623 | ||||||
2008 | 0.856 | 0.748 | 0.355 | 0.031* | 0.041* | ||||||
2009 | 0.897 | 0.731 | 0.971 | 0.975 | 0.871 | 0.252 | 0.283 | 0.306 | 0.285 | 0.004* | |
2010 | 0.606 | 0.528 | 0.492 | 0.178 | 0.200 | 0.060* | 0.157 | 0.082* | 0.545 | 0.955 | |
2011 | 0.208 | 0.091* | 0.200 | 0.910 | 0.592 | 0.014* | 0.030* | 0.015* | 0.141 | 0.194 | |
2012 | 0.670 | 0.681 | 0.750 | 0.413 | 0.141 | 0.009* | 0.092* | 0.383 | 0.009* | 0.126 | |
2013 | 0.710 | 0.503 | 0.696 | 0.131 | 0.194 | 0.001* | 0.001* | 0.003* | 0.715 | 0.751 | |
2014 | 0.759 | 0.814 | 0.735 | 0.514 | 0.351 | 0.149 | 0.018* | 0.342 | 0.069* | 0.074* | |
2015 | 0.864 | 0.766 | 0.907 | 0.267 | 0.549 | 0.005* | 0.001* | 0.008* | 0.290 | 0.750 | |
2016 | 0.383 | 0.316 | 0.380 | 0.404 | 0.733 | 0.479 | 0.010* | 0.665 | 0.002* | 0.003* | |
2017 | 0.197 | 0.192 | 0.185 | 0.198 | 0.046* | 0.109 | 0.001* | 0.353 | 0.029* | 0.118 |
RW = ring width, BAI = basal area increment, EWW = earlywood width, LWW = latewood width, LWP = latewood proportion. * P values ≤ 0.10 indicate significant differences among plots.
Figure 1 shows that tree ring characteristics had a similar pattern in the plots. In both plantations a juvenile effect was observed with a slight increase in ring width (1a and 1f) in the first years, especially in the young plantation. In the 1995 plantation, all three plots followed the same trend. BAI increased rapidly in the first years; it reached its highest point at eight, and then gradually declined (Figure 1b). The RW and EWW characteristics showed high values, but after three or four years they decreased and in the last years they stabilized at very low values (Figures 1a and 1c). On the other hand, LWW showed wide fluctuations in the first 10 years and then a gradual reduction in latewood formation (Figure 1d). Finally, LWP increased with tree age (Figure 1e) and was the only one with a significant response to the 2017 thinning (Table 2); the highest value was obtained in the plot with the largest growing space (28 %).

Figure 1 Behavior of average values (± standard error) of growth ring characteristics in trees in thinned and unthinned plots in two Pinus patula plantations.
In the 2005 plantation, RW and EWW had a similar trend. These characteristics increased in the first four or five years and then gradually decreased (Figure 1f and 1h). BAI increased rapidly in the first seven years and then decreased slightly (Figure 1g). LWW did not show a clear trend (Figure 1i), while LWP showed a gradual increase with age (Figure 1j). Compared to the 1995 plantation, the differences among the plots in the 2005 plantation were larger, in favor of the thinned plots, especially in plot 2 which had the largest growing space (26 %).
According to the literature, initial spacing and pre-thinning are the most effective tools to control tree growth and wood quality in the plantation’s early years, as they affect competition among trees and canopy closure (Guerra-Bugueño et al., 2014; Tong & Zhang, 2005). The 1995 plantation was established using narrow spacing (1.5 x 1.5 m), which generated strong competition from the early years and rapid crown recession; this situation cancelled out the expected effect of thinning. Furthermore, although a different number of trees were removed during thinning, the average CI was similar between the plots thinned, due to differences in tree dimensions when thinning was applied (0.30 ≤ CI1995 ≤ 0.39 and 0.20 ≤ CI2005 ≤ 0.28). The combined effect of the narrow initial spacing with crown recession and the small variation in growing space between plots largely explains the limited response of tree radial growth to thinnings made for management purposes.
Latham and Tappeiner (2002) and Auty et al. (2018) indicate that reducing competition for space induces an increase in tree radial growth. In turn, accelerated growth results in a higher number of earlywood cells and less latewood formation (Koga, Zhang, & Bégin, 2002). Hébert et al. (2016) state that fast growing species usually produce a higher percentage of earlywood. For that reason, a noteworthy increase in tree diameter growth was expected in the thinned plots; however, in the 1995 plantation, these plots showed very narrow growth rings in the last years, a higher proportion of latewood, and no significant differences (P > 0.10) with trees in the unthinned plot, except in the last year. Apparently, although trees in the two thinned plots had larger average spacing, they also had larger diameter and basal area per hectare, and were therefore actually exposed to similar competition (Arenas-Castro, Fernández-Haeger, & Jordano-Barbudo, 2015).
In the 2005 plantation, trees in the thinned plots showed greater growth than in the control plot, starting in the first rings. In particular, trees with more growing space showed higher growth. According to the mensuration data, immediately after the 2016 thinning, plot 2 was left with a density of 700 trees·ha-1 with a mean diameter at breast height (mDBH) of 16.85 cm (Table 1), while plots 1 and 3 showed higher density and lower mDBH. Due to the higher mDBH, basal area in plot 2 was 5.03 and 8.1 m2·ha-1 greater than in plots 1 and 3, respectively (Table 1).
Trees acquire the necessary resources to generate photosynthates and use them in various tissues and functions (Binkley, 2004). Akers, Kane, Zhao, Teskey, and Daniels (2013) indicate that light limitations influence tree growth more than soil resource limitations. In this sense, although the three plots were established with the same planting density (1600 trees·ha-1), plot 2 had higher initial mortality. This resulted in greater spacing among the trees that survived in that plot, thereby favoring radial growth.
Differences in tree ring characteristics between plantations
Table 3 shows that the characteristics of the P. patula growth rings showed differences (P ≤ 0.10) between the two plantations during the common period evaluated (2009-2017). The effects were more noticeable in RW and EWW during the years 2009 to 2015, excluding only 2011. In the case of LWW, there were differences in 2010 and 2015, while LWP was different in several years, which included the beginning and end of the common time series.
Table 3 Analysis of variance (*significant values P ≤ 0.10) of the characteristics of growth rings adjusted by cambial age, between the two Pinus patula plantations.
Year of formation | Ring width | Earlywood width | Latewood width | Latewood proportion |
---|---|---|---|---|
2009 | <0.001* | <0.001* | 0.590 | <0.001* |
2010 | 0.013* | 0.034* | 0.018* | 0.107 |
2011 | 0.608 | 0.638 | 0.911 | 0.239 |
2012 | 0.008* | 0.062* | 0.107 | 0.279 |
2013 | 0.001* | <0.001* | 0.500 | 0.009* |
2014 | 0.088* | 0.012* | 0.228 | 0.002* |
2015 | 0.011* | 0.038* | 0.034* | 0.183 |
2016 | 0.501 | 0.191 | 0.348 | 0.091* |
2017 | 0.703 | 0.364 | 0.264 | 0.073* |
The behavior of ring characteristics in the two plantations was different during the common period (2009-2017). According to Figure 2, in the first years (2009 and 2010), the trees from the 1995 plantation developed wider growth rings and had more earlywood than those from the 2005 plantation, whereas in the 2012-2015 period the opposite was true. Such behavior is related to the gradual increase in ring width in the young plantation, while in the other one the width was relatively stable during 2009-2017. It appears that crown recession in the older plantation, due to excessive competition among trees, reduced the response capacity of rings. Although trees with average competition were selected, the level of competition was similar among plots within the same plantation, but not between the two plantations. Competition was more intense in the 1995 plantation, due to differences in initial tree spacing. At the beginning of the comparison period (2009), the 1995 plantation had an average density of 2 358 trees·ha-1 and an average basal area of 47.09 m2·ha-1, while the 2005 plantation had 1 300 trees·ha-1 and a basal area of 19.06 m2·ha-1.
The young plantation showed greater growth from 2012 onwards, which is attributed to spacing between trees and the size and position of live crown (Domínguez-Domínguez, Bravo, & del Río, 2006). Crown length is largely determined by space among trees (Castelán-Lorenzo & Arteaga-Martínez, 2009; Gartner, North, Johnson, & Singleton, 2002). On the other hand, it seems that the variation in radial growth is not due to differences in site productivity, since both plantations have a similar site index (SI1995 = 33 m and SI2005 = 29 m), which was estimated from the equation adjusted by Santiago-García et al. (2017) in P. patula stands for the same study area. Although ring width varies with site conditions, pine species maintain a stable proportion of 30 % latewood in cold climates (Düthorn, Schneider, Günther, Gläser, & Esper, 2016); therefore, the value found for both plantations (approximately 20 %) indicates a combined effect of the species' productive potential and favorable environmental conditions. Pompa-García and Camarero-Martínez (2015) reported a similar proportion of latewood (18 %) in Pinus cooperi Blanco in productive sites.
The variation observed between growth ring characteristics in both plantations is attributed to the environmental conditions in which trees grow (Schimleck et al., 2018). According to Baldwin et al. (2000), as initial spacing increases, crown diameter and length also increase in trees. This favors the formation of wider rings and more earlywood. Rodríguez-Ortíz (2010) recommends an initial spacing of 2.40 to 2.75 m for P. patula because it is a species sensitive to competition in its early years.
Relationship between growth ring characteristics and climatic conditions
According to Table 4, ring characteristics (RW, EWW, and LWW) in the 1995 plantation showed a significant (P < 0.10) and positive correlation only with average minimum temperature (TAMI). On the other hand, in the 2005 plantation only the LWW showed a significant correlation, which was positive with average annual temperature and negative with annual rainfall.
Table 4 Pearson's correlation coefficients between growth ring characteristics and climatic conditions in two Pinus patula plantations.
Variables | 1995 Plantation | 2005 Plantation | ||||||
---|---|---|---|---|---|---|---|---|
TAA | TAMA | TAMI | RA | TAA | TAMA | TAMI | RA | |
RW | 0.054 | 0.239 | 0.506* | -0.295 | 0.156 | 0.266 | 0.120 | -0.084 |
EWW | 0.037 | 0.343 | 0.446* | -0.248 | 0.054 | 0.190 | 0.042 | 0.009 |
LWW | 0.063 | -0.094 | 0.398* | -0.257 | 0.698* | 0.581 | 0.534 | -0.619* |
*Significant correlation coefficients P ≤ 0.10 (n1995 = 18; n2005 = 8). TAA = average annual temperature; TAMA = average maximum temperature; TAMI = average minimum temperature; RA = annual rainfall; RW = ring width; EWW = earlywood width; LWW = latewood width.
The positive correlations of RW, EWW and LWW with TAMI in the 1995 plantation (Figures 3a, 3b and 3c) indicate that the increase in minimum temperature induces an increase in radial growth. In the 2005 plantation, this positive relationship between temperature and radial growth was only observed for LWW (Figure 3d); in contrast, the correlation between LWW and annual rainfall was negative, which indicates that in the wettest years there was less latewood formation (Figure 3e).
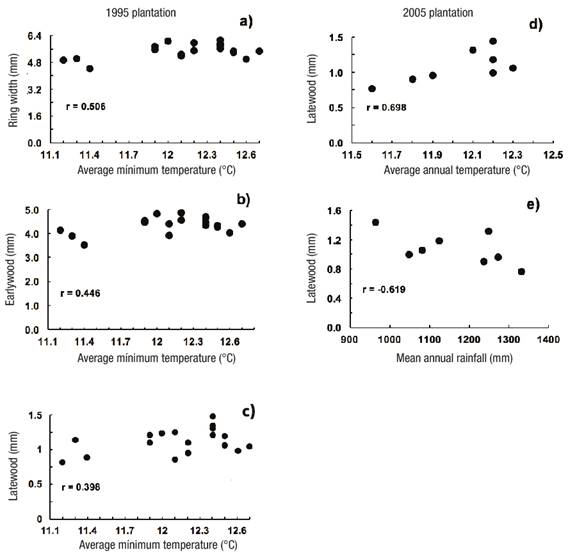
Figure 3 Pearson's correlation of Pinus patula growth ring characteristics with environmental conditions. a, b and c: 1995 plantation (n = 18); d and e: 2005 plantation (n = 8).
Temperature and rainfall are important elements in the dynamics of radial growth (Chacón-de la Cruz & Pompa-García, 2015). Consequently, a positive correlation between environmental conditions and radial growth was expected for both plantations. Growth ring characteristics in the 1995 plantation were positively associated with average minimum temperature. These results coincide with the study on wood formation carried out in Juniperus przewalskii Kom. (Zhang et al., 2018), as well as in early and latewood width in P. cooperi (Pompa-García & Camarero-Martínez, 2015). On the other hand, the negative relationship between latewood width and annual rainfall in the 2005 plantation was unexpected, as studies carried out on other species have generally indicated a positive relationship. For example, Olivar et al. (2013) indicate a positive relationship between radial growth in P. halepensis and rainfall, and attribute the development of latewood to the winter rainfall effect. Lebourgeois (2000) also found a positive relationship (r = 0.46) between latewood width and mean monthly rainfall in Pinus nigra Arn. However, due to the dynamics of cambial activity during wood formation, the relationship of growth and climatic conditions is very complex and varied (Żywiec et al., 2017).
Temperature and rainfall affect the speed and duration of cambial activity, as well as the transition from early to latewood in woody species (Rossi, Morin, & Deslauriers, 2012). For example, greater rainfall in the middle of the growth period may promote increased foliage and cambium activity, which prolongs the formation of earlywood and delays the formation of latewood. It has been demonstrated that the transition from early to latewood is associated with the cessation of apical growth (Larson, 1969) and that earlywood formation promotes high hydraulic conductivity (Cruickshank & Filipescu, 2017). In contrast, lower rainfall in the middle of the growth period may result in a faster transition to latewood formation, as part of the response to water stress and to reduce hydraulic vulnerability (Abe, Nakai, Utsumi, & Kagawa, 2003; Domec & Gartner, 2002; Wilkinson, Ogée, Domec, Rayment, & Wingate, 2015).
Conclusions
The response of Pinus patula to growing space was different in the two plantations. In the older plantation (23 years), thinnings had a lesser impact on stem radial growth and growth ring characteristics because trees, due to their larger size in the thinned plots, had competition levels similar to the control plot. In the younger plantation (13 years), the wider growing space in the thinned plots stimulated radial growth, resulting in wider rings and a greater amount of earlywood. Therefore, it is important to take into account tree age and size, as well as the level of competition between them, when defining the appropriate thinning intensity. On the other hand, temperature and rainfall during the period of wood formation influenced annual ring characteristics. Temperature had a positive effect on ring width and its components (early and latewood), which is an important issue in the context of climate change.