Introduction
Metal pollution in soils, sediments and natural water reservoirs is one of the biggest environmental problems worldwide (Srivastava & Thakur, 2006). Inorganic micropollutants are released by industries dedicated to electroplating, metal finishing, mining, tannery and battery manufacturing, among others (Ezzouhri, Castro, Moya, Espinola, & Lairini, 2009). Many metals are cytotoxic, carcinogenic and mutagenic, even at very low concentrations, as in the case of arsenic, cadmium, chromium, copper, lead, mercury, nickel, selenium, silver and zinc (Nordberg, Fowler, & Nordberg, 2015).
Metals are commonly removed by chemical precipitation, ion exchange, solvent extraction and oxide-reduction reactions; however, these methods are relatively expensive and inefficient, especially when the concentration of contaminants is in the range of 1 to 100 mg·L-1 (Krauter, Martinelli, Williams, & Martins, 1996). An alternative to these conventional methods is bioremediation, which is based on the ability of certain living organisms, such as plants, bacteria and fungi, to grow in the presence of toxic compounds (Rhodes, 2014). In particular, filamentous fungi are a group of microorganisms which has received special attention for their high surface/volume ratio (Dixit et al., 2015) and their development capacity under extreme conditions of pH, temperature and nutrient availability, as well as high metal concentrations (Anand, Isar, Saran, & Saxena, 2006).
The introduction of metals into ecosystems induces morphological and physiological changes in microbial communities (Verma et al., 2001). This implies that the contaminated sites become a source of microorganisms that are tolerant or resistant to these micropollutants (Mohammadian, Babai, Arzanbu, Oustan, & Khazaei, 2017). The mechanisms used by filamentous fungi to tolerate or resist metals include biosorption, intracellular uptake and chemical transformation, but may vary depending on the species (Danesh, Tajbakhsh, Goltapeh, & Varma, 2013).
According to the Mexican Geological Survey (2017), Sonora is ranked first among Mexican states in mineral production; in 2016, the state contributed 33.3 % of the national total. In this sense, mining has been and is synonymous with growth and development for the state and the country; however, the intense mining activity has contaminated soils and waterbodies due to the release of metals. Hence there is a need to explore new strategies to eliminate or reduce the presence of such compounds in the environment. The objective of this work was to identify a species of fungus tolerant to metals and determine if it has potential for their bioremediation.
Materials and methods
Fungus isolation and identification
Soil samples from an active mine located in the state of Sonora, Mexico, which were collected in sterilized containers, were analyzed. The samples (1 g) were deposited in 100 mL of sterilized distilled water; the mixture was stirred for 20 min at room temperature and serial dilutions were made (10-1 to 10-4). Aliquots of 100 μL of each dilution were then taken, placed in Petri dishes with PDA (potato dextrose agar) culture medium and incubated at 28 °C for five days. The developed colonies were selected and the sowing procedure was repeated in Petri dishes with PDA, until the isolation and purification of the fungal strain was achieved. This was identified based on the main morphological features of the colonies, considering macroscopic characteristics such as color, shape and type of colony, as well as the most important microscopic structures such as spores, conidial head and conidiophore.
Spore suspension preparation
The isolated fungus was inoculated in 250-mL Erlenmeyer flasks containing 50 mL of PDA, and then incubated for seven days at 28 °C. Subsequently, 100 mL of distilled water at room temperature were added and carefully stirred for 5 min with a magnetic stirrer. The spore count was performed with a Neubauer chamber. The spore suspension was used in the inoculation of the culture medium supplemented with the salts of the toxic metals to be evaluated.
Tolerance index
The salts AgNO3, CdCl2, K2Cr2O7, CuSO4, HgCl2, (CH3COO)2Pbx3H2O and ZnSO4x7H2O were used as a source of the metals Ag, Cd, Cr, Cu, Hg, Pb and Zn, respectively. The effect of the metals on the growth of the fungus, also known as the tolerance or resistance index, was determined following the methodology described by Ezzouhri et al. (2009), under which the index is defined as the ratio of the extension radius of the colonies of the fungus treated with the metal to that of the colonies of the fungus without metal (control), that is to say:
The isolated fungus was seeded in PDA supplemented with the metal salts, individually, at a concentration of 1 mM. Previously, a hole was made in the center of the PDA solidified in the Petri dishes in order to obtain circular-shaped colonies and thus enable measurements of the radius. A suspension of 108 spores·mL-1 was deposited in said hole; the dishes were incubated at 28 °C. The growth of the fungus was determined seven days after the inoculation by measuring the radius of the colonies; all tests were performed in triplicate. The spores used were collected from a culture of the fungus with seven days of previous growth in PDA.
Minimum inhibitory concentration
The minimum inhibitory concentration (MIC) is defined as the lowest concentration of metal that inhibits the growth of a microorganism (Ezzouhri et al., 2009). In this study, to determine the MIC, the fungus was inoculated in the PDA culture medium containing, individually, the metal salts AgNO3 (silver nitrate), CdCl2 (cadmium chloride), K2Cr2O7 (potassium dichromate), CuSO4 (copper sulfate), HgCl2 (mercury chloride), (CH3COO)2Pbx3H2O (lead acetate trihydrate), and ZnSO4x7H2O (zinc sulfate heptahydrate) as a source of the metals Ag, Cd, Cr, Cu, Hg, Pb and Zn, respectively, at concentrations of 5, 10, 15 and 20 mM. Inocula of 108 spores·mL-1 with seven days of growth were used. The inoculated plates were incubated at 28 °C for seven days. The growth of the extension radius (cm) of the colonies was measured every 24 h, to determine the tolerance index at each concentration. All tests were performed in triplicate.
Results and discussion
Identification of the isolated fungus
According to the macroscopic characteristics, the colonies of the fungus in PDA, after seven days of growth, were white to yellowish (between the first 24 to 48 h of culture), turning black and granular, with yellow to green on the opposite side (Figure 1). Among the microscopic features, globose conidial heads of black to brown tone stand out, along with smooth, light brown conidiophores and conidia or globose spores. The characteristics mentioned match those of the fungus Aspergillus niger Tiegh. 1867 (Abarca, 2000; Sáez, Flórez, & Cadavid, 2002).
Tolerance index of the fungus
Prolonged exposure of soil fungi to metals can generate a physiological adaptation with important modifications that reduce the number of populations. The changes may be associated with the increase in biosorption, bioaccumulation and metal transformation capacity (Ashraf & Ali, 2007). Iram, Zaman, Iqbal, and Shabbir (2013) found that some fungi of the genus Aspergillus usually predominate in soil contaminated with metals.
In this study, the tolerance index of A. niger, exposed to a 1 mM concentration of each metal salt, was greater than 0.80 after seven days of growth. Table 1 shows the tolerance index of the fungus to each of the evaluated salts. A filamentous fungus can be considered highly tolerant when exposed to a concentration of at least 1 mM of metal and the tolerance index is equal to or greater than 0.80 (Ezzouhri et al., 2009). Based on the above, the isolated filamentous fungus was highly tolerant to all metals evaluated.
Table 1 Tolerance index of Aspergillus niger exposed to 1 mM of AgNO3, CdCl2, K2Cr2O7, CuSO4, HgCl2, (CH3COO)2Pbx3H2O and ZnSO4x7H2O as a source of the metals Ag, Cd, Cr, Cu, Hg, Pb and Zn, respectively, after seven days of incubation at 28 °C.
Metal | Tolerance index of the fungus |
---|---|
Cd | 0.89 ± 0.12 |
Hg | 1.03 ± 0.14 |
Pb | 1.05 ± 0.18 |
Ag | 0.94 ± 0.11 |
Cu | 0.88 ± 0.04 |
Zn | 0.87 ± 0.07 |
Cr | 1.27 ± 0.07 |
± Standard deviation of the mean
When A. niger was exposed to a 1 mM concentration of cadmium chloride, a tolerance index of 0.89 ( 0.12 was obtained. This result is slightly higher than that described by Ezzouhri et al. (2009) for Penicillium sp. (S4S) and Penicillium sp. (S5S) with indices of 0.55 and 0.62, respectively, using cadmium sulfate as the source of said element. These authors also determined that the index of Alternaria alternata (Fr) Keissl. 1992 is only 0.13 and that several species of Fusarium sp. did not grow in the presence of 1 mM of cadmium. Roane and Pepper (2000) mention that tolerance levels among fungal species may be different due to the variation in resistance mechanisms.
In the case of mercury (1 mM of mercury chloride), A. niger had a tolerance index of 1.03 ( 0.14, so it was highly tolerant to metal. Kurniati, Arfarita, and Imai (2014) reported that Aspergillus flavus Link 1809 had a tolerance index of 0.80 with 25 ppm of mercury chloride.
Lead is the metal that is found mostly as a contaminant in soil, water and air and is also highly toxic to plants, humans, animals and microorganisms (Low, Lee, & Liew, 2000), hence the importance of the search for alternatives to treat sites contaminated with this metal. In this sense, it was found that A. niger is highly tolerant to 1 mM of lead acetate, since its index was 1.05 ± 0.18.
In this work, it was observed that A. niger was highly tolerant to silver, since the index was 0.94 ± 0.11 (using silver nitrate as the source of the element). At present there are not many studies that demonstrate the tolerance or resistance of filamentous fungi to this metal; however, Singh (2006) found that Fusarium oxysporum Link 1809 reduces silver ions in solution, resulting in a stable suspension.
With respect to copper (1 mM of copper sulphate), A. niger showed tolerance rates of 0.88 ± 0.04; that is, the fungus is highly tolerant. Ezzouhri et al. (2009) indicated similar values of tolerance to 1 mM of copper: 0.79, 0.98, 0.91 and 1.0 for the fungi Penicillium sp. (S2W), Penicillium sp. (S2S), Fusarium sp. (S1S) and Aspergillus niger (S5W), respectively.
The tolerance index of A. niger to 1 mM of zinc sulfate was 0.81 ± 0.07; therefore, the fungus was highly tolerant. Sintuprapa, Thiravetyan, and Tanticharoen (2000) suggested that certain species of filamentous fungi tolerate zinc thanks to the mechanism of uptake through intra- and extracellular exchange in the form of polyphosphate precipitation.
Finally, in the case of chromium (1 mM of potassium dichromate), A. niger had a high tolerance index of 1.27 ± 0.06. Ezzouhri et al. (2009) reported similar indices in other fungi; these authors found that A. alternata and Penicillium sp. were highly tolerant to chromium (potassium dichromate as a source) with indices of 1.02 and 1.23, respectively. They also indicated that Geotrichum candidum Link 1809 is not very tolerant to metal, since they determined an index of 0.19.
Minimum inhibitory concentration
Table 2 indicates the minimum concentration of metals (in the form of a metal salt) that inhibited the growth of A. niger. Mercury, copper and silver were the most toxic for A. niger, since the MIC was found between 5 and 10 mM of each metal salt, while, for cadmium chloride, the MIC was between 15 and 20 mM. In the presence of lead, zinc and chromium salts, the fungus grew even with the highest concentration of these metals; therefore, the MIC was greater than 20 mM. In this sense, other studies with higher concentrations of lead, zinc and chromium are necessary to find those that inhibit the growth of A. niger.
Table 2 Minimum inhibitory concentration (MIC) of Aspergillus niger exposed to AgNO3, CdCl2, K2Cr2O7, CuSO4, HgCl2, (CH3COO)2Pbx3H2O and ZnSO4x7H2O as a source of the metals Ag, Cd, Cr, Cu, Hg, Pb and Zn, respectively.
Metal | MIC (mM) / CMI (mM) |
---|---|
Cd | 15 < MIC < 20 / 15 < CMI < 20 |
Hg | 5 < MIC <10 / 5 < CMI <10 |
Pb | MIC > 20 / CMI > |
Ag | 5 < MIC < 10 7 5 < CMI <10 |
Cu | 5 < MIC <10 / 5 < CMI < 10 |
Zn | MIC >20 / CMI > 20 |
Cr | MIC > 20/v |
At the lowest concentrations of the metal salts (1 and 5 mM), the fungus was very tolerant and showed strong growth, exceeding the control (without metal) in the case of lead, zinc and chromium. The growth pattern suggests the development of tolerance or the adaptation of the fungi to the presence of metals.
Figure 2 shows the tolerance indices of A. niger exposed to 5 mM of each metal salt. From said concentration, the lag phase was prolonged in comparison with the control, especially with Cd, Hg, Cu and Ag; the growth of the fungus could be observed and measured up to three days after the inoculation.
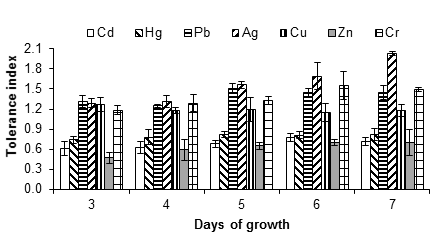
Figure 2 Tolerance index of Aspergillus niger in the presence of 5 mM of AgNO3, CdCl2, CuSO4, HgCl2, (CH3COO)2Pbx3H2O, ZnSO4x7H2O and K2Cr2O7, as a source of the metals Ag, Cd, Cu, Hg, Pb, Zn and Cr, respectively. The standard deviation of the mean is shown on the bars.
It was observed that A. niger was tolerant up to 5 mM of silver nitrate, since the fungus had no growth capacity at 10 mM. Silver is a metal widely used in the photographic, electrical, electronic and chemical industries; the soluble form, as in other heavy metals, is very dangerous because it is easily transported and becomes available for plants and animals. For humans, poisoning with this type of metal can cause severe dysfunction of the kidneys, reproductive system, liver, brain and central nervous system (Dixit et al., 2015), hence the importance of finding possible strategies to treat sites contaminated with the metal.
In the presence of copper (copper sulfate), the MIC of A. niger was between 5 and 10 mM. The fungus had growth capacity up to 5 mM with a tolerance index of 1.18 ± 0.38, after seven days of incubation (Figure 2). Ezzouhri et al. (2009) found similar MIC values for Penicillium sp. (S4W) and Penicillium sp. (S5S), being 7.5 mM < MIC < 10 mM for both fungi, also using copper sulfate as the metal source. According to Kermasha, Pellerin, Rovel, Goetghebeur, and Metche (1993), the tolerance or resistance of fungi to copper, such as A. niger, is due to an active process that involves the synthesis of a metallothionein (a copper-dependent protein).
Mercury appears to be toxic to Aspergillus; despite this, certain species are still able to grow at certain concentrations of the metal. In this work, A. niger was highly tolerant to mercury (tolerance index of 1.03 ± 0.14 at 1 mM of mercury chloride). When increasing the concentration of the mercurial salt to 5 mM, the tolerance index decreased to 0.82 ± 0.03. The fungus showed significant growth capacity in the presence of up to 5 mM of mercury chloride, but the inhibition was complete at 10 mM. Another study found that A. flavus tolerates up to 100 ppm of mercury (Kurniati et al., 2014). It has also been indicated that Phanerochaete chrysosporium Burds 1974 is tolerant and has the ability to accumulate this metal (Dhawale, Lane, & Dhawale, 1996).
Figure 3 shows the tolerance index of A. niger at 10 mM of cadmium, lead, zinc and chromium salts. This concentration reduced growth and increased the lag phase compared to the control (without metal). However, in some cases, the fungus grew relatively quickly, even after a long lag phase, for example with lead and chromium salts at 15 and 20 mM. The reduced growth rate is a typical response of fungi in the presence of toxins (Gadd, 1993), while the lengthening of the lag phase is not always present (Ezzouhri et al., 2009).
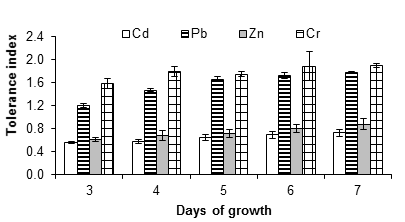
Figure 3 Tolerance index of Aspergillus niger in the presence of 10 mM of CdCl2, (CH3COO)2Pbx3H2O and ZnSO4x7H2O, K2Cr2O7 as a source of the metals Cd, Pb, Zn and Cr, respectively. The standard deviation of the mean is shown on the bars.
Figure 4 shows that A. niger had growth capacity up to 15 mM of cadmium chloride with a tolerance index of 0.61 ± 0.09, after seven days of incubation at 28 °C; however, at 20 mM there was no growth. This could be due to an inhibition effect resulting from the poisoning of the fungus, since cadmium is considered one of the most dangerous metals due to its high stability and toxicity. Joo and Hussein (2012) observed a strong inhibition of the growth of A. niger exposed to 1 mM of cadmium. Jabbari, Faezi, Khosravan, Farahmad, and Shakibaie (2010) observed that cadmium exerts severe inhibitory effects on the physiological processes of organisms, in some cases, even at concentrations less than 2 ppm. By contrast, Massaccesi, Romero, Cazau, and Bucsinszky (2002) indicated that several species of filamentous fungi, isolated from contaminated industrial sediments, were able to remove 63 to 70 % of the cadmium during 13 days of growth. In addition, Mohammadian et al. (2015) indicated that Aspergillus versicolor Vuillemin 1903 and Aspergillus fumigatus Fresenius 1863 have MIC of 2 000 mg·L-1 and 1 000 mg·L-1, respectively. This shows the adaptability of microorganisms, as well as their potential for use in bioremediation.

Figure 4 Tolerance index of Aspergillus niger in the presence of 15 mM of CdCl2, (CH3COO)2Pbx3H2O, ZnSO4x7H2O and K2Cr2O7 as a source of the metals Cd, Pb, Zn and Cr, respectively. The standard deviation of the mean is shown on the bars.
Figure 5 shows that A. niger had greater resistance to lead, zinc and chromium, since it tolerated a concentration of 20 mM of the salts used as a source of these metals. In the case of lead, the fungus had a tolerance index of 0.71 ± 0.12 after seven days. An increase in the growth of the fungus was observed at all evaluated concentrations; Ezzouhri et al. (2009) observed similar behavior in A. alternata, which increased its growth by 4 % in the presence of 1 mM of lead carbonate. These authors also found that the MIC (mM) of lead for Penicillium sp. (S3S) Penicillium sp. (S4W), Penicillium sp. (S5S), Fusarium sp. (S5S), A. niger (S5W) and A. niger (S5S) was 12.5 < MIC < 15, 20 < MIC < 25, 7.5 < MIC < 10, 12.5 < MIC < 15, 20 < MIC < 25 and 25 < MIC < 30, respectively; all strains were isolated from soil with mining activity. On the other hand, Sanyal, Rautaray, Bansal, Ahmad, and Sastry (2005) reported that lead is not toxic to the fungus F. oxysporum, which grows easily after exposure to this metal.

Figure 5 Tolerance index of Aspergillus niger in the presence of 20 mM of (CH3COO)2Pbx3H2O, ZnSO4x7H2O and K2Cr2O7 as a source of Pb, Zn and Cr, respectively. The standard deviation of the mean is shown on the bars.
The fungus A. niger was moderately tolerant to zinc, since indices of 0.87 ± 0.07, 0.55 ± 0.01, 0.88 ± 0.10, 0.66 ± 0.02 and 0.55 ± 0.01 were obtained at 1, 5, 10, 15 and 20 mM of zinc sulfate, respectively, after seven days of growth. Given that even at 20 mM significant growth of the fungus mycelium was observed, the MIC is greater than this concentration. Vadkertiova and Slavikova (2006) found that Pichia anomala Hansen 1904, Candida krusei Berkhot 1923 and Cryptococcus laurentii (Kuff.) C. E. Skinner 1950 tolerate zinc at concentrations higher than 20 mM, a result similar to that found in this work with A. niger. Castro-Silva et al. (2003) also observed such similarity in yeast strains isolated from coal mines. On the other hand, Levinskaite (2002) showed that the growth rate of Penicillium atramentosum Pitt 1980 25SL slowly decreased from 40 mM of zinc, using zinc sulfate as the metal source. Moreover, Sintuprapa et al. (2000) suggested that the mechanism of zinc uptake, of certain species of filamentous fungi, is intra- and extracellular exchange in the form of polyphosphate precipitation. Zinc is essential for all organisms, although at high concentrations it can become toxic (Balsalobre et al., 2003).
The chromium source promoted the growth of the fungus at all concentrations evaluated. Tolerance indices of 1.27 ± 0.06, 1.49 ± 0.06, 1.90 ± 0.03, 1.35 ± 0.01 and 1.30 ± 0.05 were found for 1, 5, 10, 15 and 20 mM of potassium dichromate, respectively; that is, A. niger is highly resistant and the MIC is greater than 20 mM. In this sense more studies are required to find the chromium concentration that inhibits the growth of A. niger. In this regard, Ezzouhri et al. (2009) used potassium dichromate, as a source of chromium, and observed similar MIC values (20 mM < MIC <25 mM) for fungal species such as Penicillium sp. (S3S) and Fusarium sp. (S5S), while for Penicillium sp. (S4W), Penicillium sp. (S5S), A. niger (S5W) and A. niger (S5S) the MIC were lower: 12.5 < MIC < 15, 15 < MIC < 20, 12.5 < MIC < 15 and 10 < MIc < 12.5, respectively. The detoxification of chromium by A. niger can be mediated by an antioxidant enzyme system such as peroxidase, catalase and ascorbate peroxide (Srivastava & Thakur, 2006).
In general, the growth pattern of the fungus suggests the development of tolerance and adaptation to the presence of the metals evaluated. With 1 mM of each metal salt, the growth of the fungus began 24 h after incubation, while, starting at 5 mM, the growth of the fungus began at 72 h, which implies an extension of the lag phase. Figure 6 illustrates the tolerance indices of A. niger at the different concentrations evaluated for each metal, during seven days of culture. The most toxic metals for the fungus were silver, mercury and copper, since from 10 mM of the metal salts there was no growth, followed by Cd (15 mM). At 20 mM, the growth of the fungus was only observed with the lead, zinc and chromium salts.

Figure 6 Tolerance index of Aspergillus niger at 1, 5, 10, 15 and 20 mM of AgNO3, CdCl2, CuSO4, HgCl2, (CH3COO)2Pbx3H2O, ZnSO4x7H2O and K2Cr2O7, as a source of the metals Ag, Cd, Cu, Hg, Pb, Zn and Cr, respectively, after seven days of culture at 28 °C. The standard deviation of the mean is show on the bars.
This and other studies have found that filamentous fungi tolerate the presence of toxic metals. Therefore, these microorganisms are considered excellent candidates to accumulate metals in their mycelia and spores and even to reduce toxicity (Bennet, Wunch, & Faison, 2002). Biosorption, intracellular uptake and chemical transfer are some of the mechanisms that fungi use to tolerate, accumulate and transform toxic metals, although they can vary depending on the species (Chatterjee, Mukherjee, Sarkar, & Roy, 2012; Dixit et al., 2015). Biosorption is based on the interactions between metals and living or non-living cells (biomass) of microorganisms (Izkandar, Zainudin, & Tan, 2011) and includes ion exchange, chelation, adsorption, crystallization, and precipitation, followed by diffusion through the cell wall (Danesh et al., 2013). In filamentous fungi, the wall is formed by chitin, chitosan, glucans, proteins, lipids and other polysaccharides. This composition confers on the cell wall a large number and variety of functional groups or sites, such as an ion exchange resin. On the other hand, the mechanisms of transfer or chemical transformation are reduction, methylation and dealkylation (Danesh et al., 2013; Gadd, 1993). With this last type of mechanism, it is important to consider the possibility that the product resulting from the transformation, by the action of the microorganisms, is more toxic than the initial form of the metal; this can happen with lead or mercury. This research did not review the production of methylated lead and mercury compounds, but it has been considered to do so in subsequent studies.
Conclusions
The results of this research indicate that the filamentous fungus A. niger, isolated from a site with industrial activity such as mining, has the capacity to tolerate high metal concentrations. The tolerance level depended on the metal, with cadmium, mercury, silver and copper being the most toxic, while lead, zinc and chromium did not affect the growth of the fungus at the highest evaluated metal salt concentration (20 mM). Therefore, it is suggested that A. niger is a promising candidate for the removal of metals in contaminated environments. It is recommended to determine the amount of metals that this fungus can bioaccumulate and transform, as well as to evaluate different culture conditions (temperatures, pH, contact times and metal mix).