Introduction
Rotifers are part of the microzooplankton and due to their unique characteristics, these organisms have been widely employed as an essential source of feed for fish larvae (marine and fresh water) in aquaculture (Odo et al., 2015). Two thousand rotifer species have been described, most of them belong to fresh water bodies and a lesser number to marine environments (Lubzens & Zmora, 2003). From the two most important marine rotifer species in mariculture, Brachionus plicatilis is the most frequently used to feed several fish and crustacean species (Rehberg-Haas et al., 2015), the nutrients provided by the rotifer vary according to its diet. In addition, B. plicatilis is not difficult to digest and easily transfers nutrients (essential amino acids and long-chain fatty acids) to the organism that feeds on it, therefore, this species is largely employed in larviculture (Zhou et al., 2009).
The mass production of rotifers in commercial laboratories demands high quantities of live feed (microalgae) with high nutrimental value and physiological characteristics that enable its consumption by B. plicatilis. However, one of the main disadvantages of microalgae mass culture is the high production costs of using traditional microalgae (Isochrysis galbana, Nannochloropsis oculata, Tetraselmis suecica, Dunaliella salina, Pavlova lutheri, Chaetoceros muelleri, and Thalassiosira pseudonana) (Wikfors & Ohno, 2001). These species must be cultured under very specific controlled parameters such as light (120-170 µmol photons m-2 s-1), salinity (32-36 UPS), temperature (19-23°C), among others. Therefore, in order to reduce production costs, alternatives are pursued through the use of new native microalgae species that are adapted to the environment of the working area. Specially microalgae from tropical and subtropical environments, given that these strains are exposed to raised levels of solar radiation and have evidenced high synthesis of antioxidants and an increased ability to transform solar light into lipids (Lim et al., 2012). For a new microalgae strain to be considered live feed for rotifers, it must comprise the following characteristics: appropriate size, digestibility, planktonic habits and must be easy to culture. In addition, the biochemical composition must contain high concentration of proteins (essential amino acids), carbohydrates, and lipids (omega-3 fatty acids). It is important to note that microalgae are the source of two of the most difficult nutrients to synthetize, eicosapentaenoic acid EPA; 20:5n − 3) and docosahexaenoic acid (DHA; 22:6n − 3) (Tacon & Metian, 2013).
Several species of microalgae have been used to feed B. plicatilis, however, this rotifer feeds on different items including several species of microalgae, bacteria, and bacteria flocculates, depending on the natural or culture environment, and feeding is limited to the size of crown and mouth respectively, thus, there are no specific microalgae species to feed B. plicatilis (Hino et al., 1997).
Derived from the increased aquaculture activities and the need for alternative sources of lipids and proteins to feed larvae and other organisms, it is essential to suggest new microalgae species that substitute the costly traditional microalgae, mainly Chaetoceros spp. and Isochrysis galbana. This would require the isolation, characterization and assessment of microalgae strains to be used as live feed. In this study, we considered an important geographic area for aquaculture to collect microalgae. Four tropical strains were obtained and partially characterized, the nutritional input for the rotifer Brachionus plicatilis was evaluated in order to substitute traditional microalgae and minimize production costs.
Materials and methods
Microalgae isolation and purification
Seawater samples were collected at Playa Las Salinas (26° 4’13.58”N, 109°23’58.67”W), located in the northwestern portion of Ahome municipality in the state of Sinaloa, and at Bahia de La Paz (24°16’7.66”N, 110°19’29.53”W) in the state of Baja California Sur, Mexico. Sampling was performed to obtain plankton species at 1 m depths in the water column. Samples containing microalgae were transported to the Experimental Aquaculture Laboratory in Pichilingue unit of Universidad Autonoma de Baja California Sur (UABCS) located at kilometer 17 in the highway to Pichilingue, La Paz, B.C.S.
Samples were filtered on a WHATMAN® (1 µm) filter and rinsed with sterile seawater to reduce the presence of bacteria. Microalgae were sown into test tubes with sterile seawater and F/2 medium (Guillard, 1973) as nutrient source. Strains were isolated by different methods, such as serial dilutions and sowing in marine agar plates (DB-2216). Once mono-algal strains were obtained, 24 h baths were performed with antibiotic (gentamicin 240 mg 3mL-1) at a concentration of 10 µL in 300 µL of culture. Microalgae were sown by streaking in marine agar medium plates to verify the absence of bacteria.
The isolated microalgae were measured and photographed with a microscope Nikon (Optiphot-2) and camera (Sight Ds-L1). These organisms were identified at the genus level according to the keys described by Tomas (1997) and O’sullivan & Reynolds (2004). The microalgae strains were cultured under controlled conditions of constant light (33.7 a µmol photons m-2 s-1), continuous aeriation, and average temperature of 22 ± 2°C. Taxa were morphologically identified as: Chaetoceros sp. (key number LPU-1), Chaetoceros sp. (key number LPU-3), Synechococcus sp. (key number LPU-10) and Dicrateria sp. (key number LPU-8).
Microalgae were grown in F/2 medium (Guillard, 1973) up to 17 L each. Growth curves were estimated at these volumes by daily counts using a Neubauer counting chamber. The cultures were semicontinuous with a daily renewal rate of 25 % of the culture volume. The harvested volume was used in the experimental treatments to feed B. plicatilis on a daily basis.
Evaluation of diet in Brachionus plicatilis
The assessment of live feed for B. plicatilis was performed in 19 L propylene containers. The experiment started at a concentration of 6.3 ± 0.4 rot mL-1. The wasted feed and rotifer concentration before feeding were recorded daily using a hemocytometer and Sedgewick-Rafter counting-slide, respectively. The concentration of feed for B. plicatilis was constant (80 000-100 000 cell mL-1) at all treatments. Isochrysis galbana (T-ISO) was used as control since it is one of the most commonly employed microalgae to feed B. plicatilis and was available in the strain collection of the Microalgae Laboratory of UABCS. Cultures were maintained in triplicate for seven days. After this period, the total of each microalga and rotifer culture was harvested by centrifugation at 4000 rpm for 30 min (IEC, model GP8R) and using a 40 µm mesh sieve, respectively. All harvests were rinsed with ammonium formate (5 %) to reduce salts. Samples were frozen at -40 °C and lyophilized for 48 h (Virtis Co, mod 12525) for bromatological analysis.
The bromatological composition of microalgae and rotifers was determined in triplicate employing spectrophotometric techniques (Beckman model DU® 640). Protein content was established according to the method suggested by Lowry et al. (1952), Lactalbumin (99 % pure) was employed to build the calibration curve. For total lipids, the method suggested by Bligh & Dyer (1959) was used, tripalmin (99 % pure) was employed to build the calibration curve. Ashes were determined by calcination at 480ºC for 24 h in a Thermolyne muffle. Weight comparisons were performed to establish ashes content.
Statistical analyses
Percentage data of the bromatological components (proteins, carbohydrates, and lipids) of microalgae and rotifers fed different diets were transformed (arcsin) for normalization. A one-way (ANOVA) was conducted to determine statistical differences among treatments. A Tukey’s test was employed for statistical comparisons among treatments. All statistical analyses were performed in STATISTICA 7 software.
Results and Discussion
In this research we isolated local microalgae from Bahia de La Paz and Sinaloa, the weather in this geographic area varies considerably, water temperature ranges 21-31°C throughout the year (Castro et al., 2000). This environmental variation requires microalgae to adapt and/or produce biochemical compounds that guarantee their survival (Duong et al., 2015). The population growth of the different microalgae isolated in our study evidences that these species adapt to culture under laboratory conditions for mass production (Figure 1) Despite the fact that in this research microalgae were not cultured outdoors, according to Pacheco-Vega et al. (2015), tropical endemic microalgae present a wide plasticity that enables them to respond to variations caused by external factors during culture, favoring the mass production of microalgae.
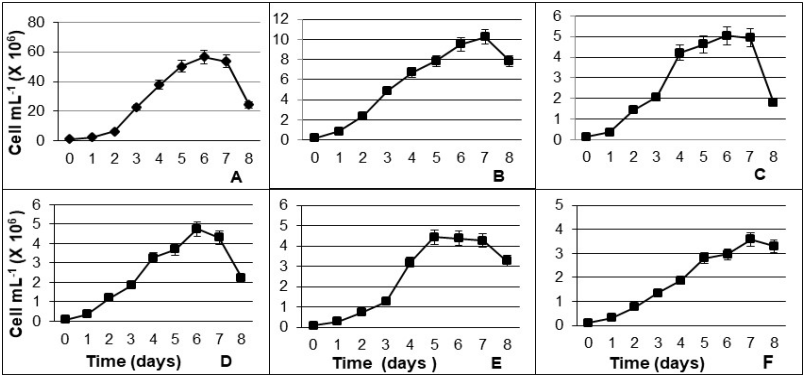
Figure 1 Growth of microalgae under controlled laboratory conditions. A) Synechococcus sp. (LPU-10), B) Dicrateria sp. (LPU-8), C) Chaetoceros sp. (LPU-2), D) Chaetoceros sp. (LPU-3), E) Chaetoceros sp. (LPU-4), and F) Isochrysis galbana. Data are mean± SDs.
Each microalga species follows a specific pattern of standard growth (Huesemann et al., 2016), thus, our results indicate that the differences found are due to the presence of different taxa. The population growth among species exhibited the different phases and periods for each microalga; in addition, the maximum densities for each group were observed, Synechococcus sp. and Dicrateria sp. presented the highest cell densities with 50 240 000 cell mL-1 and 10 231 250 cell mL-1, respectively.
Most microalgae strains can successfully grow under laboratory conditions; however, optimal growth may vary according to each strain. Therefore, performing a single culture method might not ensure good growth in all of the strains due to the varied requirements of light, temperature, nutrients, and other water conditions among species (Huesemann et al., 2016). In Figure 1-A it is shown that the cyanobacteria Synechococcus sp. reached a higher cell density than microalgae, this is because the growth rate in cyanobacteria is higher than in microalgae (Sánchez-Alejandro and Sánchez-Saavedra 2015). Rosales-Loaiza et al. (2008) reported densities up to 406.13 X 106 ± 21.74 at 27 °C, while in our study, the maximum density was 56.54 x 106, this divergence is explained by differences in the culture conditions employed in both researches, mainly temperature (26.5 °C and 23°C, respectively). In addition, microalgal growth is influenced by variations of organic nutrients and this is reflected in the bromatological composition of microalgae (Prieto et al., 2005). Synechococcus sp. and Dicrateria sp. are small sized-cells, hence these microorganisms reproduce faster than larger cells, such as Chaetoceros sp. in this study, which reached exponential stage at day two with a density of 5 x106 cell mL-1. Similar values were revealed by Rosales-Loaiza et al. (2012) in a semicontinuos culture of Chaetoceros sp., with a maximum density of 6.1 x106 cell mL-1, growth stages were also coincident with our research.
With regard to population growth of the rotifer B. plicatilis in this study, the best diets (p>0.05) were Chaetoceros sp. (LPU-2) and control (Figure 2), followed by Chaetoceros sp. (LPU-3). The least favorable diets were Chaetoceros sp. (LPU-4), Synechococcus sp. (LPU-10) and Dicrateria sp. (LPU-8) (Figure 2).
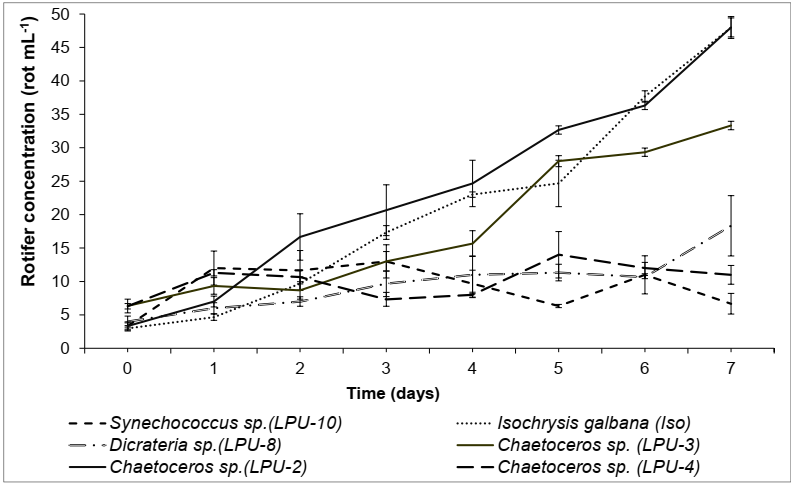
Figure 2 Population growth of the rotifer B. plicatilis fed different diets of microalgae. Data are mean± SDs.
Before choosing a diet for a given organism, the nutritional requirements of that particular species must be considered, in this case, B. plicatilis presents general nutritional requirements of proteins, lipids, and carbohydrates in a specific microalgal size. Abou-Shanab et al. (2016) noted that species of the genus Brachionus are filter feeders that select the particles to be ingested. Hotos (2002) evidenced the selective capacity of B. plicatilis for feeding, the author compared several microalgae as feed and found that the rotifer preferred the microalga Asteromonas gracilis ranging 20-25 µm. In this study, LPU-2, with which B. plicatilis presented the best population growth, was smaller (5.1-5.7 µm) than the size reported by Hotos (2002). In addition to good particle size, in this research, similar bromatological compounds were detected between Chaetoceros sp. (LPU-2) and I. galbana, which confirms the relevance of Chaetoceros sp. (LPU-2) as feed for B. plicatilis.
Bromatological composition of microalgae and rotifers
In order to be considered as good quality feeds, the microalgae employed in aquaculture must present favorable chemical composition, size, acceptability, and digestibility, as well as being manageable under culture conditions. The bromatological composition of the microalgae evaluated in this study varied (p>0.05) according to each genus (Figure 3). Among the bromatological constituents, proteins were found in a larger proportion. Chaetoceros sp. (LPU-2) was statistically similar to I. galbana with an average protein content of 31.7 % and 31.8 %, respectively. These percentages are relatively consistent with what was detected by Jamali et al. (2015) for Chaetoceros muelleri (39.8 %) and I. galbana (30.3 %), the slight differences are explained by particularities in age and conditions of each culture. The concentrations of these constituents enhanced the population growth of B. plicatilis.

Figure 3 Bromatological composition of different microalgae: Isochyrisis galbana (Iso), Synechococcus sp. (LPU-10), Dicrateria sp. (LPU-8), Chaetoceros sp. (LPU-2), Chaetoceros sp. (LPU-4), Chaetoceros sp. (LPU-3). Different letters in the same row indicate significant differences (Tukey’s test). Data are mean± SDs.
The carbohydrate concentration was similar between the control (I. galbana) and Chaetoceros sp. (LPU-2), although the highest values were obtained with the cyanobacteria Synechococcus sp. (LPU-10), averaging 35 %, which is concordant with Brown et al. (1997), who noted that chlorophytes are carbohydrate-rich microalgae. As mentioned earlier, the composition of microalgae is important for rotifer feeding, especially the protein: carbohydrate proportion, one example is the rotifer Brachionus manjavacas that was fed microalgae with high content of protein and low content of carbohydrates, the life period and reproduction of the rotifer increased (Snell, 2014). In the results of our study, the highest protein: carbohydrate proportions were detected in diets Chaetoceros sp. (LPU-2) and I. galbana (control), therefore, the highest population growth for B. plicatilis were found with these diets.
The ash content varied among species. Chaetoceros spp. (LPU-2, LPU-3, and LPU-4) are diatoms, and given their frustules made of silica, these microalgae presented the greatest quantity of ashes. In concordance with Martínez-Córdova et al. (2012), who found ash concentrations ranging 34-46 % for C. muelleri, our results ranged 34-42.3 %.
Lipids act directly on rotifer development, hence, lipid deficiencies during culture may cause problems in reproductive processes, loss of vision, anemia and mortality, mainly derived from a decrease in the performance of phospholipids within the central nervous system and brain (Glencross, 2009). Therefore, in addition to high protein content, an increased lipid concentration is required for feeds and rotifers cultured in the laboratory. The content of lipids, proteins and carbohydrates in B. plicatilis is directly influenced by ingested feed (Cabrera et al., 2005). This statement is consistent with the results obtained in this study, the maximum lipid value recorded for B. plicatilis was 26% when fed Chaetoceros sp. (LPU-3), which was one of the diatoms with greatest lipid content (Figure 4). In addition, our result is concordant with the value reported by Campaña-Torres et al. (2012) for B. rotundiformis (21 % lipid content) fed Nannochloropsis oculata. Moreover, Melo Costa et al. (2009) used C. calcitrans to feed B. plicatilis and the population growth of the rotifer was equivalent to the values obtained at day 7 in the present study. This is attributed to the fact that the protein and lipid content is similar between Chaetoceros sp. (LPU-2) and C. calcitrans, these characteristics are essential components of structural functions within cells, nutrient transport, among others; otherwise a low content of lipids would hinder the population density due to a decrease in the hatching rate.
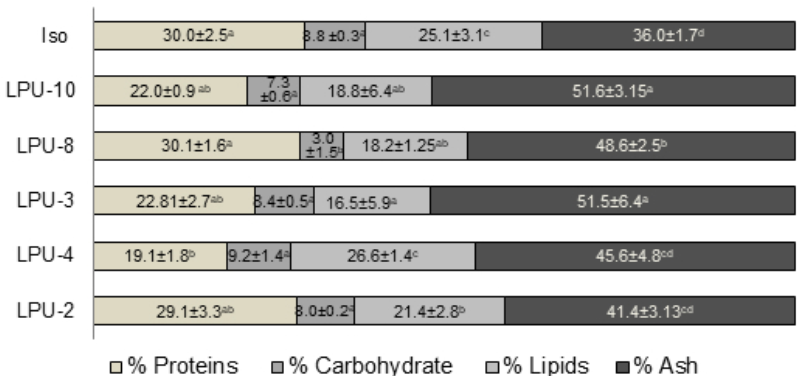
Figure 4 Bromatological composition in the rotifer B. plicatilis fed during 8 days with different microalgae: Isochyrisis galbana (Iso), Synechococcus sp. (LPU-10), Dicrateria sp. (LPU-8), Chaetoceros sp. (LPU-2), Chaetoceros sp. (LPU-4), Chaetoceros sp. (LPU-3). Different letters in the same row indicate significant differences (Tukey’s test). Data are mean± SDs.
Conclusions
The microalgae and cyanobacteria isolated from Ahome, Sinaloa and Bahia de La Paz, B.C.S., and identified as Synechococcus sp. (LPU-10), Dicrateria sp. (LPU-8), Chaetoceros sp. (LPU-2), Chaetoceros sp. (LPU-4) and Chaetoceros sp. (LPU-3) are feasible for management and culture under laboratory conditions. In addition, the composition of B. plicatilis varies according to each microalga. Chaetoceros sp. (LPU-2) presents the highest protein content, and similar to the control diet (Isochrysis galbana), the population growth of B. plicatilis is 48 rotifers mL-1 at day 7 when fed this microalga. Hence, Chaetoceros sp. (LPU-2) is suggested for culture to feed Brachionus plicatilis.