Introduction
The use of microalgae as food for shrimp larvae during the first life stages is a common practice in shrimp farming. As live food, microalgae are a source of highquality nutrients given the varied content of essential aminoacids and fatty acids that are fundamental for shrimp growth and development (Pedroza-Islas, 2002). In shrimp-culture systems of tropical and subtropical areas, different strategies are implemented: microalgae can be supplied as single (monoalgal) or as several mixed species. Unfortunately, these feeding strategies are limited due to the reduced number of species that can be incorporated into shrimp culture, which are maintained at temperatures ranging 26-31 °C, while microalgae need controlled temperatures that range 22-26 °C, causing problems related to thermal shock. In addition, the unknown origin of these species in many cases results in ecological impacts and increases production costs due to the putative presence of nonnative species (Gozlan, 2010).
Aquaculture faces a number of challenges to develop as profitable and sustainable activity. One of the most important challenges is to achieve aquaculture production without exploiting natural resources such as soil or water (Crab et al., 2012). In this sense, some microalgae can promote bacterial growth in discontinuous cultures, enhancing metabolite products that are beneficial for the development of shrimp larvae. Over the last few years, this process has generated interest towards the use of probiotic bacteria in shrimp-culture systems (Leal et al., 2010).
When microalgae and bacteria are cultured together, they become more digestible by larvae and other small marine organisms, stimulating growth and survival. Bacteria are actively engaged in the process of digestion of microalgae due to the production of enzymes that are external to the digestive system of larvae (Riquelme & Avendano-Herrera 2003). According to Natrah et al. (2013), microalgae act as capsules that incorporate beneficial bacteria into the digestive system of shrimp larvae. In addition, microalgae contribute to larval feeding with nutritional elements and compounds that inhibit the growth of pathogenic microorganisms, either by competitive exclusion mechanisms or by producing bactericide substances. Moreover, mixed cultures of bacteria and microalgae can promote microalgae growth in the culture systems (De-Bashan & Bashan, 2010). Although it is worth noting that the presence of microalgae and bacteria can affect the digestive physiology of shrimp.
The enzymatic activity, mobilization and storage of reserve energy have been evaluated for qualitative and quantitative determination of proteins, lipids and carbohydrates (Arcos-Ortega et al., 2015). Nevertheless, the aquaculture industry must promote research for novel species of marine microalgae and bacteria that are able to maintain an efficient culture performance and present characteristics that enhance nutritional quality, especially digestive processes and water quality improvements (Crab et al., 2012; Simões et al., 2002). This type of research can positively impact shrimp culture, particularly improving the growth stage. In this regard, it has been detected that some bacteria-microalgae associations such as BFT (biofloc) in shrimp culture present dynamic processes due to the environmental variables that impact the systems, triggering the generation of metabolites that benefit shrimp welfare (Jagadeesan et al., 2014; Natrah et al., 2013). For instance, bacteria from the genus Bacillus can improve nutrient digestibility in shrimp larvae (Aguirre-Guzmán et al. 2012; Ziaei-Nejad et al., 2006). Furthermore, the temperature range of the environment in which bacteria and microalgae live is a variable that may influence microalgae-bacteria-shrimp interactions. Although it is not possible to generalize the effect of temperature over mutualist species, it is possible to predict whether bacteria and algae strains isolated from similar environments will show a positive interaction, and thus allow to generate balanced and controlled culture systems, mainly for water quality control (Zhou et al., 2009).
The number of microalgae used in aquaculture is limited. If the variety of species used in this activity is diversified, areas of live feed production may be reinforced, the nutritional quality improved and the biological diseases within culture systems decreased (Crab et al., 2012). By including new microalgae species in aquaculture, an optimization of intensive systems is expected, as well as the promotion of sustainable practices for shrimp development and water quality, which will allow to reduce production costs.
In this study, the effect of including four novel strains of probiotic microalgae and bacteria in diets of shrimp cultured in a subtropical environment (Bahia de La Paz, Mexico) was assessed. Several shrimp diets were evaluated with the following addition methods: monoalgae, mixed microalgae, and microalgae mixed with probiotic bacteria from laboratory strains (obtained from the digestive tract of an adult individual of Penaeus vannamei) containing Lactobacillus sp. (Keys: TD23, TD219, and R42C). In order to determine the capacity of shrimp larvae to digest the nutritional compounds contained in microalgae, we evaluated changes in the proximal composition of P. vannamei with different feeding strategies using microalgae and probiotic bacteria.
Material and Methods
Culture of microalgae and probiotic bacteria
The microalgae evaluated in this study were collected in Bahia de La Paz, Baja California Sur, Mexico. These were isolated and preserved in the strain repository at Universidad Autonoma de Baja California Sur (UABCS). Culture mediums were enriched in F/2 culture medium (Guillard,1975). Strain culture was maintained in test tubes and flasks under controlled conditions and inoculating in progressive volumes. Fernbach flasks (≥ 1 L), carboys, and cylindrical translucent polycarbonate tanks (300 L) were maintained outdoors without artificial light or temperature control. The 300 L tanks were maintained at semi-continuous cultures (i. e. 30 % volume was daily harvested and refilled with enriched and sterilized sea water). This yield was used to feed the shrimp larvae.The experimental treatments were compared with Chaetoceros muelleri, used as control feed, which was maintained at 21 ± 1 ˚C, continuous light (2 500 lux) usingday-light lamps, and constant aeration. The bacteria strains evaluated were provided by the strain repository of Ciencia y Tecnologia de Alimentos (BCSSU) from UABCS. These strains presented bacillary morphology and non-sporulating, catalase-negative and gram-positive characteristics. Probiotic bacteria were reactivated and cultured in MRS broth at 34˚C. Cultures were conducted at 1 L volumes and centrifuged at 3,500 rpm for 30 min, the supernatant was discarded and bacteria were recovered for resuspension using 500 mL of sterilized seawater to be furtherly added to the tanks (80 mL to each tank, at a density of 1 x 108 UFC mL L-1).
Experimental conditions for Penaeus vannamei
Penaeus vannamei nauplii (stages 4-5) were acquired from a local shrimp hatchery (Granjas Marinas de Sinaloa, S.A. de C.V.: GRANMAR) in Baja California Sur, Mexico. Nauplii were maintained in a 15 L polyethylene bucket with constant air stream and acclimated at 27-28 °C for 3 h. After hand-blending the bucket content, a volumetric count of the larvae was performed in order to estimate the number of nauplii mL-1 considering 10 samples of 10 mL each, nauplii were distributed in the experimental units afterwards.
Table 1 Treatments and description of diets for P. vannamei larvae under culture conditions.
Trial | Description |
---|---|
A. | Chaetocerosmuelleri (control) |
B. | Grammatophora sp. (LPU-7) |
C. | Schizochytrium sp. (LPU-1) |
D. | Navicula sp. (LPU-6) |
E. | Grammatophora sp.+ Schizochytrium sp. |
F. | Grammatophora sp.+Schizochytrium sp.+Probiotic |
G. | Navicula sp.+ Schizochytrium sp. |
H. | Navicula sp.+ Schizochytrium sp.+ Probiotic |
The experiment was conducted in an isolated culture room where the environmental temperature was maintained at 30 ±1 °C using electric heaters. The culture system consisted in 24 units of 120 L flat-bottom glass-fiber tanks placed in a two-row arrangement. All experimental units were supplied with filtered seawater (1 μm), commercial chloride at 35 ppt was added and neutralized for 24 h with sodium thiosulfate. Air was supplied continuously through aquarium diffusers. Photoperiod was 12 -12 h without water exchanges. Seven trials with a control were conducted as follows:
All treatments were performed in triplicate (24 tanks), starting from stage Zoea I to Mysis I. Shrimp larvae were distributed in the treatments of each experiment at a density of 170 Nauplii L-1 (i. e.11,900 organisms per experimental tank). All experimental units were covered with a polyethylene sheet to avoid pollution among tanks. Temperature, pH, dissolved oxygen, and salinity were recorded on a daily basis using a Hanna HI9828 equipment.
The feeding regime comprised the addition of microalgae and microalgae-probiotic mix diets (feeding trial: A - H). Microalgae presented a cell density of 80,000 to 120,000 cells mL-1 and three probiotic bacterial strains were added in equal proportions (1:1:1) to a final density of 114 x103 UFC mL-1. To determine the effect of diet on the zootechnical parameters of Penaeus vannamei larvae, survival and size were recorded from Zoea I to Mysis I stages. Considering all experimental units, the survival of organisms was estimated with the percentage of live organisms recorded at each stage; size was determined on the basis of total length of 50 organisms at the start of the experiment and 20 at the end; identification of the each of the early developmental stages of P. vannamei was made considering morphological characteristics (Kitani, 1986). Length was measured in a stereoscopic microscope using a manual micrometer (Reichert).
To evaluate the effect of diet on larvae composition, a sample of Zoea I was collected using a mesh sieve at the start of the experiment, while the remaining larvae were collected at the end. Larvae were washed with distilled water, placed in 55 mL Falcon flasks, lyophilized and storaged at -40 °C for bromatological analysis, which consisted on ashes, protein (Bradford, 1976), total carbohydrates (Whyte, 1987) and total lipids (Bligh & Dyer, 1959); the latter was also estimated for microalgae.
To determine the presence of probiotic Lactobacillus spp. in the experimental tanks, 2 mL were sampled and spread in Petri dishes (20 μL) containing MRS medium and incubated for 24 h at 34 ˚C for colonies counts. Sampling was performed at the start and at the end of the experiment.
Statistical analyses
Prior to statistical analyses, normality tests and homogeneity of variance of data were performed. Survival percentage and protein, lipids and carbohydrates contents for microalgae and larvae were transformed to arc-sinus; subsequently, one-way variance analysis was performed at all cases. The effect of diet on growth from Zoea I to Mysis I was evaluated by one-way variance analysis at all trials. In cases where significant differences were found, a posteriori Tukey’s HSD analysis was applied. Statistical analyses were carried out using STATISTICA 7.0 for Windows software (Statsoft, USA; α=0.05).
Results and Discussion
Development of P. vannamei in culture
The cell density of microalgae varied among strains. Non-axenic cultures were maintained at semi-continuous conditions since day 4 (Figure 1). Lag phases were observed in all of the curves, this is evidenced by the different growth rates of each microalgae species when compared to the control (C. muelleri), which presented defined temperature and light conditions. Since day 4, cell density was affected in 30 % of the cultures, the highest concentrations were found in C. muelleri. The biomass concentration and growth potential were detected in the semi-continuous cultures of Grammatophora sp., Schizochytrium sp. and Navicula sp. The maximum concentration was shown on day 9 for the control experiment. The mass outdoor culture of microalgae offers the advantage of acquiring a low-cost live feed; in addition, the use of endemic microalgae for shrimp-larvae production decreases the environmental risks of introducing new species to a foreign habitat. In this connection, this type of research allows to support solutions for the current challenges to achieve an economically sustainable aquaculture, given that it integrates the already existing culture systems (microalgae and shrimp larvae) and that it constitutes a lipid-rich highly-nutritional feeding alternative (Crab et al., 2012).

Figure 1 Increase of cell density in four microalgae species under semi-continous controlled (Chaetoceros muelleri) and outdoor (Grammatophora sp., Schizochytrium sp. and Navicula sp.) mass culture of microalgae.
According to the survival values obtained from stages Nauplii to Mysis I fed different diets (A, B, E and F), probiotics and mixed algae, these showed significant differences (p<0.05) among treatments. The best survival was observed in larvae maintained with Grammatophora sp.+ Schizochytrium sp. (Gr+Sk), Grammatophora sp.+ Schizochytrium sp. + Probiotic (Gr+Sk+Prob) and Chaetoceros muelleri (control), while larvae maintained with Navicula sp. presented a mortality near 100 % (Figure 2). Previous publications report good bromatological composition when feeding organisms with these microalgae (Navicula sp.), which have even been considered for the formulation of pellets for fish, such as the species Sparus aurata (Reyes-Becerril et al., 2013; Pacheco-Vega et al., 2015a). The bromatological composition of Navicula sp. was reported by Curbelo et al. (2004), who, given its good composition, proposed a live feed for shrimp larvae using this strain in combination with Artemia salina. In the present research, Navicula sp. cells settled in the bottom forming a film, resulting unavailable to larvae, this is because shrimp have a planktonic behavior during the first life stages and do not feed on settled microalgae (Sainzand-Hernández & Cordova-Murueta, 2009). Thus, in this experiment Navicula sp. was not consumed.
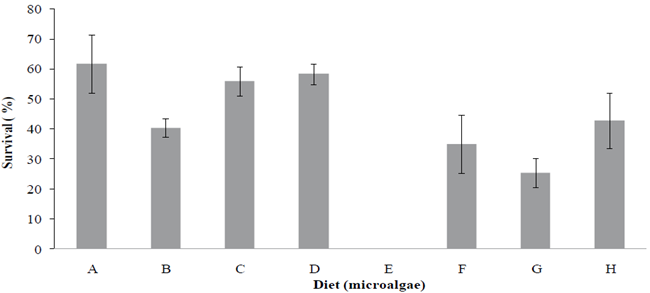
Figure 2 Survival of Penaeus vannamei at the larval stage Zoea I fed with Chaetoceros muelleri (A), Grammatophora sp. (B), Grammatophora sp.+ Schizochytrium sp. (C), Grammatophora sp.+ Schizochytrium sp. + probiotic (D), Navicula sp. (E), Schizochytrium sp. (F), Navicula sp. + Schizochytrium sp. (G), Navicula sp. + Schizochytrium sp. + probiotic (H).
Larvae size presented differences among experiments, the largest size was reached with the control treatment (3.0 mm) and showed significant differences (p<0.05) with regard to the rest of the trials (Figure 3). It is worth noting that the control treatment and those including monoalgal Grammatophora sp., Grammatophora sp. plus Schizochytrium sp., and Grammatophora sp. plus probiotics, reached Mysis I stage in day 9, whereas treatments of single Schizochytrium sp., in combination with Navicula sp. and probiotics, reached the same stage until day 11. According to this result, size may have been influenced by factors such as water quality and characteristics intrinsic to the genera and species of the strains selected.

Figure 3 Penaeus vannamei sizes at the larval stage Mysis I fed with Chaetoceros muelleri (A), Grammatophora sp. (B), Grammatophora sp.+ Schizochytrium sp. (C), Grammatophora sp.+ Schizochytrium sp.+ Probiotic (D), Navicula sp. (E), Schizochytrium sp. (F), Navicula sp. + Schizochytrium sp. (G), Navicula sp. + Schizochytrium sp. + Probiotic (H).
In recent years, it has been reported that in addition to bromatological components, around 10 000 secondary metabolites are known for microalgae (Fusetani, 2000), these are taxon-specific (genera and/or species) and may influence the organisms that feed on them (Roy & Pal, 2015; Van Durme et al., 2013). Moreover, the combination of Grammatophora sp. and Schizochytrium sp. used to feed Penaeus vannamei larvae presented high survival. Diets comprising two microalgae species obtain better survival, this is subsequently reflected on benefits to larvae survival due to the varied nutritional supply provided by several microalgae, different from the limited input of a single microalgal diet (Jamali et al., 2015). In our experiment, the microalgae demonstrated to be feasible for culture in outdoor conditions, without temperature or light control. In the present study, water quality remained homogenous among treatments and in acceptable ranges within culture conditions (Boyd & Tucker, 1998). Larval survival, growth and the elapsed time for metamorphosis are attributed to the biochemical composition of microalgae, given that cell components can present variations (proteins, lipids, carbohydrates).
Bromatological composition in shrimp larvae
The physical characteristics of microalgae-cell membranes are directly related to the activity of highly-digestive carbohydrates. Specifically, in trials with Schizochytrium sp., given this microalga presents flexible and digestible cell walls and high polysaccharides content (Darley et al., 1973). In our study, the physical and chemical characteristics of feed (microalgae) showed a direct effect on larvae digestion and assimilation of nutrients (Table 2).
Table 2 Bromatologic composition (ashes, proteins, carbohydrates and lipids) present in the digestive system of Penaeus vannamei larvae fed with different species of microalgae. Mean values and standard deviation are included.
Treatment (diet) | Proteins (%) | Lipids (%) | Carbohydrates (%) | Ashes(%) |
---|---|---|---|---|
A | 55.97 ± 1.81 | 20.45 ± 2.13 | 3.12 ± 0.92 | 19.33 ± 2.96 |
B | 66.90 ± 4.24 | 16.17 ± 3.41 | 1.76 ± 0.04 | 17.67 ± 3.34 |
C | 55.78 ± 4.36 | 15.73 ± 1.71 | 4.56± 0.96 | 20.09 ± 20.09 |
D | 58.17 ± 1.42 | 17.05 ± 1.82 | 2.91 ± 0.25 | 17.34 ± 1.33 |
E | - | 19.24 ± 0.84 | - | - |
F | 63.26 ± 6.26 | - | 4.57 ± 0.64 | 17.02 ± 0.51 |
G | 55.74 ± 2.88 | 12.51 ± 2.21 | 3.68 ± 0.23 | 18.82 ± 1.66 |
H | 57.65 ± 2.42 | 21.00 ± 3.64 | 6.56 ± 0.77 | 19.09 ± 1.28 |
Where: Chaetoceros muelleri (A), Grammatophora sp. (B), Grammatophora sp.+ Schizochytrium sp. (C), Grammatophora sp.+ Schizochytrium sp. + Probiotic (D), Navicula sp. (E), Schizochytrium sp. (F), Navicula sp. + Schizochytrium sp. (G), Navicula sp. + Schizochytrium sp. + Probiotic (H).
The total protein percentage in Zoea I ranged 55.7-66.9 %, the lowest values were found in the diet Navicula sp. + Schizochytrium sp. + Probiotic and the highest in Grammatophora sp. For shrimp, protein requirements are of major importance in terms of nutrition. In this experiment, a high content of crude protein was detected in the bromatological analysis of microalgae. Therefore, this diet combination is favorable given that, in addition to the proteic contents, it is also easy to digest by P. vannamei larvae. Pacheco-Vega et al., (2015a) reported that these microalgae species present low protein concentrations; however, the protein content is not relevant when analyzed alone, it can be supported in combination with different protein sources (plant or animal) or the presence of bacteria (Ziaei-Nejad et al., 2006). Other factors influence the digestive process of feed in larvae, these can be exogenic factors such as temperature, food transit, pH of larvae, and dissolved oxygen, or endogenic factors, such as larvae size and location of the digestive organs, which provide important information to perform a comprehensive analysis on the use of feed by larvae (McGaw & Curtis, 2013). For instance, determining endogenic factors would allow us to characterize the development of the digestive tract of shrimp larvae until adulthood. Sainz-Hernández & Córdoba, (2009) detected that the feeding habits of shrimp larvae are mainly on the basis of microalgae, these habits are modified when they reach the juvenile stage, given that digestive enzymes such as trypsin and chemotrypsin (proteolytic enzymes) start to develop due to predation on small animals, which increases protein requirements in the diet.
Regarding water quality parameters (dissolved oxygen, temperature, and salinity), these were maintained within the optimal range for shrimp culture, thus these variables did not affect our results. A good and constant water quality is desirable for the development of sustainable aquaculture, where natural-resource exploitation must be avoided (Crab et al., 2012).
The total lipid content observed was lower in larvae fed Navicula sp. + Schizochytrium sp., while higher concentrations were detected in larvae fed Chaetoceros muelleri (control) and Navicula sp. + Schizochytrium sp.+ Probiotic. Pacheco Vega et al. (2015b), found that Grammatophora sp. present a series of polyunsaturated fatty acids, which may enhance lipid digestibility.
Carbohydrates content ranged 1.76-6.75 %, the lowest value was recorded in Mysis I with Grammatophora sp. and the highest percentage was found in larvae fed with Schizochytrium sp. These results indicate that the latter microalgae present a high content of carbohydrates; however, there is no evidence that these were digested by P. vannamei larvae. As commented earlier, this might be due to the enzymatic adaptation in the late Zoea stage that is caused by changes in feeding habits (Sainz-Hernández & Cordova, 2009), or else to the fact that despite there is no evidence indicating that carbohydrates constitute a basic feeding requirement for shrimp (Jamali et al., 2015), it is possible that the carbohydrate content varied given the presence of microalgae, since carbohydrates are present in cell membranes (Sørensen et al., 2016).
The nutrimental input supplied by microalgae and the presence of probiotic bacteria in shrimp larvae cultures enhance carbohydrate digestibility, given that once microalgae cell membranes are broken, the components become available as substrates, allowing the digestion of intracellular proteins and lipids. In this study, similar to lipids, carbohydrate digestibility might have been stimulated by the presence of probiotic bacteria in the culture environment, considering that these Bacteria belonged to the genus Bacillus, which contribute with exogenous enzymes that enhance nutrient digestion (Ziaei-Nejad et al., 2006). As shrimp-digestive tract develops from larvae to juvenile stage, shrimp can process food with high-protein content, thus mixed feed (microalgae and/or lactic acid probiotic bacteria) may promote nutrient digestibility in diets. In this study, the mixed diets of native species balanced growth rates and reduced production costs when compared with the nonnative C. muelleri.
Conclusion
Results in this study suggest that the outdoor culture of P. vannamei shrimp larvae fed with mixed feed consisting of Grammatophora sp. and Schizochytrium sp., native from the Gulf of California, and the probiotic mix of the genus Lactobacillus spp. (Key: TD23, TD219 and R42C), isolated from adult P. vannamei digestive tract, present high potential for larvae culture. The combination of these mixed diets stimulates shrimp digestive enzymes, which promote the optimal use of food. Using native species in culture systems is environmentally friendly.