Introduction
Bison are considered an ecological keystone species as they contribute to heterogeneity and diversity in grasslands through selective grazing, seed dispersal, redistribution of nutrients via faeces, urine and carcasses, trampling of vegetation that accelerates the reintegration of nutrients into the soil, by knocking down trees and shrubs by rubbing on them, and by forming wallows (Hobbs 1996; Knapp et al. 1999; Reynolds et al. 2003; Anderson 2006; Melis et al. 2007). One of the most distinctive behaviors of bison is rolling over lifting its legs, and repeatedly turning from side to side on its back, creating oval depressions in the ground that alter the composition of vegetation in large areas across North American grasslands, these wallows being a distinctive feature of bison-occupied grasslands (Bracke 2011; McMillan et al. 2011).
Several studies have evaluated the impact of bison wallows on the diversity of North American grasslands, both in tall and mixed-grass prairie, considering soil and vegetation variables and analyzing currently used and vestigial wallows (Collins and Uno 1983; Polley and Collins 1984; Polley and Wallace 1986; Trager et al. 2004; McMillan et al. 2011). Significant differences have been reported in soil variables like texture, moisture, pH, N-NH4, Na and Mg, as well as in the net primary production between the center, the margin and the outside of the wallows (McMillan et al. 2011).
In general, it has been found that wallows present less plant diversity than the adjacent grassland area, but there are plant species that only establish themselves within these, increasing the diversity of the grassland (Polley and Wallace 1986; Trager et al. 2004; McMillan et al. 2011). Wallows also have a direct effect on the establishment of other species of animals. Gerlanc and Kaufman (2003) evaluated the use of wallows by anuran species to oviposit, and complete metamorphosis, as wallows are temporary water reservoirs that remain long after the rainy season has ended.
Bison are absent from most of their former range and therefore their ecological effect is nonexistent except for the few places where they are found in significant numbers. Efforts are under way to recover the ecological role of bison at a continental scale (Sanderson et al. 2008). As part of this effort, a herd of bison was reintroduced in November 2009 within the Janos municipality in northern Chihuahua, México (SEMARNAT and CONANP 2009).
The objectives of this work were to determine if within the short-term after the reintroduction, ecological effects due to wallowing were evident in soil properties and vegetation features; to establish the baseline to evaluate the effect of the wallows over time; and to determine if wallowing was associated to a sex, age class, season, and a particular plant association.
We hypothesized that inside wallows the soil would be more compact, retain more moisture, and have higher nutrient contents, especially N due to bison rolling over, urinating and defecating. We further expected to find different plant species inside and outside the wallows, and expected the wallowing activity to be predominant among males.
Materials and methods
Study site. The study was conducted at El Centro, a 1,600 ha pasture (30° 50’ 58.58” N, -108° 27’ 7.33” W; 1,412 masl; Figure 1) inside the El Uno ranch, located within the Janos Biosphere Reserve, in the municipality of Janos, Chihuahua.
The pasture is located within an ancient alluvial fan formed by the direct dragging of sediments from the northern Sierra Madre Occidental. It is characterized by the presence of many shallow desert washes, with a south to north direction, which connect to a greater wash at the limit of the fan west of the site. This wash becomes evident in periods of higher rainfall and causes temporary flooding.
The dominant soils correspond to Xerosols, Regosols, Vertisols, and Phaeozems, according to the classification of INEGI and SEMARNAP 1998 (CONANP and SEMARNAT 2006), where the representative parental material is mainly alluvium. The surrounding mountain ranges are basalt and rhyolite formations formed during the Tertiary and the early Pleistocene (Nordt 2003).
The drought in the northern region of the Chihuahuan Desert, together with overgrazing, has changed perennial into annual grassland (Ceballos et al. 2010; Davidson et al. 2010), with extensive areas invaded by mesquite (Prosopis glandulosa; Humphrey 1987). The predominant vegetation type at the site correspond to shortgrass prairie, represented by species such as: blue grama (Bouteloa gracilis), black grama (B. eriopoda), sideoats grama (B. curtipendula), hairy grama (B. hirsuta), spreading threeawn (Aristida divaricata), fescue (Festuca sp.), and toboso (Hilaria mutica). There are also shrubs such as the ocotillo (Fouquieria splendens), prickly pear (Opuntia sp.), cholla (O. imbricata), tumbleweed (Salsola kali), and longleaf jointfir (Ephedra trifurca) in disturbed areas (Humphrey 1987; List 1997; CONANP and SEMARNAT 2006).
The climate of the region is semiarid temperate with hot summers (maximum average of 50 °C) and cold winters (minimum average of -12 °C; BSok (x'); García 1998). Average annual rainfall is 306.7 mm, of which 65 % falls between July and September (List 1997; Nordt 2003).
Study population. On November 27, 2009, a herd of bison without genetic introgression of cattle and free of diseases (brucellosis, tuberculosis) was reintroduced in El Uno. The herd came from Wind Cave National Park, in South Dakota, United States. Bison were introduced to form a reproductive herd for future reintroductions within the historical range of the species in México (SEMARNAT and CONANP 2019). During the study, the herd consisted of 16 females and 3 males between 3 and 4 years of age, a 2-year-old female, born in 2010, 9 calves born in 2011 and 7 calves born in 2012. The bison conformed a single group, they have access to permanent artificial bodies of water, the property is fenced so they do not coexist with any other ungulate species and competition and predation are not important factors for the population, since the only large carnivore present in the region is the coyote (Canis latrans).
Location and use of the wallows. Four periods of observation of the herd were conducted in 2012-2013: spring (April-May), summer (July-August), autumn (October-November), and winter (December-January), with a duration of 25 days each. To sample the complete daylight period, observation times were alternated: from dawn - 09:59, 10:00 -13:59, and 14:00 - to dusk. The frequency of the rolling behavior, the individual who performed it, the time of the day, and the GPS coordinates of each wallow were recorded.
We mapped the plant associations and the location of wallows using a Google Earth satellite image (2007; 1:25,000) georeferenced in UTM coordinates. For the characterization of the plant associations, the image characteristics were correlated against the vegetation characteristics. 200 reference points were obtained on field to perform a supervised classification with the Spatial Analyst extension, using the maximum likelihood statistic. Plant associations were determined based on the dominant elements (plant species). Additionally, 176 points were recorded on field to corroborate the GIS-generated map. The percentage of the total number of points that coincided was estimated to obtain an accuracy value. The area for each type of plant association (percentage) was determined with respect to the total area of the paddock using the GIS ArcMap 10.1.
The number of wallows within each of the associations was determined. The number of records expected by category (plant association) was calculated based on those observed, and the generation of wallows in the different plant associations was evaluated according to their availability using the X2 statistic. Bonferroni confidence intervals were calculated to determine use.
The frequency of wallowing behavior was analyzed by time of year, period of the day, and sex, using the Kruskal-Wallis non-parametric statistical analysis. A multiple pair comparison test was performed following the methodology proposed by Conover (1999). Plant and soil sampling was performed during the time of maximum biomass accumulation (late August - early September).
Effect of wallows on the diversity and abundance of vegetation. Six wallows were selected in each of the three plant associations most used for this activity. These were randomly selected on the satellite map, considering that they should be separated from other wallows by a minimum distance of 10 m and a distance greater than 20 m from roads and fences, and were characterized considering the length and width of each wallow.
The percentage of plant cover, and the height of the foliage of each plant species were measured at two sampling places: inside the wallow (in five points, corresponding to the cardinal points and the center) and five meters around it (in four points, corresponding to the four cardinal points), using quadrants of 0.25 m2 (Davidson et al. 2010). Vegetation samples were collected and prepared according to standard preparation methods for later identification in the laboratory (Sánchez-González and González 2007).
An analysis of variance (ANOVA) was used to establish if there were significant differences between the inside and the outside of the wallows for the variables height and vegetation cover. To estimate differences between plant species composition, a contingency table was constructed and evaluated with the Χ2 statistic.
Effect of wallows on soil parameters. Soluble salts. During the same period, undisturbed soil samples with 100 ml cylindrical cores were taken. The presence of soluble salts in the soil of the study site was evaluated by measuring the electric conductivity (EC) and the potential of Hydrogen (pH) of soil-water suspensions. Ten grams of dry and sieved soil were weighed, 25 ml of distilled water were added, and the EC and pH were measured in the supernatant with a multiparameter meter (HI2020-01, Hanna Instruments, USA), equipped with a pH electrode and a conductivity probe. Additionally, the soil texture was determined with the finger probe in the field, and the moisture retention at field capacity (FC) was estimated on behalf of the soil texture, estimated organic matter content and the bulk density after Siebe et al. (2006). With these data, the electric conductivity at moisture contents at field capacity (EC pF1.8) was estimated at each site.
Bulk density (BD) and volumetric moisture content. To evaluate compaction and soil moisture the BD was determined. Undisturbed samples were taken at two depths (5 and 10 cm), with 100 cm3 cylinders collected inside the wallow (12 × 2 randomly distributed samples) and around it at a distance of 5 m (12 × 2 samples distributed randomly around the circumference). The cylinders were weighed, dried in the oven at 105 °C for 48 h and reweighed (Siebe et al. 2006; Bélanger and Van Rees 2007).
Nutrients. To determine the concentration of nutrients, composite samples were taken with cores from 2 to 7.5 cm depth inside the wallow (12 randomly distributed samples) and five meters around it (12 randomly distributed samples around the circumference). The individual core samples were pooled and preserved in hermetically sealed plastic bags.
For the determination of nitrates and ammonium, from each sampling point a small sub-sample was kept in the refrigerator at 4 °C, until its subsequent analysis in the laboratory.
Laboratory analysis. A total of 48 composite samples were collected and extracted with 1 M Potassium Chloride (KCl) solution in duplicates. The mean of the duplicates is reported.
Nitrogen: Nitrates (NO3) and Ammonium (NH4). The determination of Nitrates (NO3) and Ammonium (NH4) was done colorimetrically following standard procedures with a nitrogen autoanalyzer (Perkin Elmer 2400). The standard solutions were prepared from Potassium Nitrate (KNO3) and Ammonium Chloride (NH4Cl) and readings were made at wavelengths of 410 and 660 nm, respectively.
Phosphorus. Available phosphorus was determined based on the Bray-Kurtz method (Black 1965a), since it has been considered the most suitable for acid soils. Phosphorous extractions was quantified by colorimetry and determined by spectrophotometry (Perkin Elmer 3110) at a wavelength of 720 nm.
Six of the samples were analyzed by the Olsen method (Black 1965b) because they presented a pH greater than 7. In this case, Phosphorous was extracted with a sodium bicarbonate solution, and quantified by colorimetry. Absorbance was determined by spectrophotometry at a wavelength of 660 nm.
Exchangeable Cations. Exchangeable cations were extracted with 1 N ammonium acetate at pH = 7; Calcium (Ca) and Magnesium (Mg) values were obtained in a Perkin Elmer 3110 atomic absorption spectrometer; Sodium (Na) and Potassium (K) were obtained with a Sherwood emission spectrometer (Van Reeuwijk 1992). A principal component analysis (PCA) was carried out to establish the variables that expressed the variability in the data between plant associations (field capacity (FC), BD, texture (% clay), electric conductivity (EC) and pH. We used PCA as a dimension reduction technique that estimates artificial axes for exploring dispersion graphs, with optimal properties, of the underlying variability and covariability, based on orthogonal rotations given the level of correlation of the original variables and the factors. Biplots and Minimum Path Graphs were generated from the PCA results; i) Biplot was constructed by constructing a scatter diagram of the observations from the PCA on the connections matrix (covariances) of all variables and overlapping the eigenvectors representing each variable scaled on the same space, while; ii) Minimum Path Graphs was constructed with the distances of the point rows of the data matrix in the original space, connecting variables with straight segments securing all points and linked without loops while maintaining the distance of the segments to the minimum (Gower and Ross 1969). The loads of the factors were considered when estimating all the components in order to control both for high influence of each variable and the rotation method.
To evaluate the effect of the location (inside and outside the wallows) and depth (0 to 8 cm or 8 to 13.5 cm) on BD and moisture, the non-parametric statistic Kruskal Wallis was used, since the data did not comply with the assumption of normality. Likewise, a test of multiple pairwise comparisons was carried out following the methodology proposed by Conover (1999).
Based on the concentration of nutrients, the distribution of the data was estimated using the normality test (Shapiro Wilks). A mixed effects model was generated by means of a generalized least squares analysis to evaluate the influence of the location (inside and outside the wallow) and the vegetation on the concentration of each of the analyzed nutrients.
A model with interactions between the location and the vegetation was generated and the graph of the standardized residuals against the adjusted values was obtained, since there was apparent homogeneity of variance. For this, three models with different variance structure were tested and the one with the best fit was selected according to the AKAIKE information criterion.
Results
Location and use of the wallows. A total of seven plant associations and 219 wallows were recorded within the “El Centro” pasture: longleaf jointfir scrub with grassland (41.5 %; 35 wallow records), blue grama grassland (20.1 %; 26 wallow records), annual grassland (14.2 %; 44 wallow records), toboso grassland (13.5 %; 93 wallow records), bare soil (5.4 %; 11 wallow records), vine mesquite (2.8 %; 8 wallow records), and grassland in black-tailed prairie dog colony (2.6 %; 2 wallow records). For the analysis, the three main plant associations used for this activity were selected. These corresponded to toboso grassland (mainly represented by Hilaria mutica), vine mesquite (Panicum obtusum) and annual grassland (Aristida adscensionis; Table 1; X20.05 = 219.71, g.l. = 6P > 0.05) as these three had higher observed values than expected.
Based on the frequency of the behavior of rolling on the ground, significant differences were observed between the different times of the year (H = 86.85, P < 0.0001); individuals wallow more frequently in summer and do less so in the winter and fall. When analyzing behavior by period of the day, significant differences were also found (F = 102.95, P < 0.0001). In the period between 10:00 and 14:00 the frequency of the behavior increased, being more evident during the summer. In spring, winter and autumn, the frequency decreased regardless of the period of the day (Figure 2).
Significant differences were also found between the winter (H = 13.39, P = 0.0328), autumn (H = 15.39, P = 0.0183) and summer (H = 29.77, P = 0.0002) seasons, both between sexes and by period of the day. However, during the spring, no significant differences were found between the sexes and by period of the day (H = 7.04, P = 0.4931). It was determined that during the morning and at noon in winter, females, and calves wallow more than males; in autumn, the frequency of the behavior is very low in the morning and increases at noon. Although this pattern is repeated during the summer, the frequency increases markedly and the differentiation between the sexes is more evident. Males wallow more frequently than females and females in turn wallow more than calves, however, in general the behavior increases at noon. During summer, the frequency of wallowing increased regardless of the sex of the individual. In general, it was observed that calves and females wallow less frequently during the morning and in the afternoon, except for winter, where males present the behavior to a lesser extent (Figure 3).
Table 1 Use of the different plant associations for wallowing.
Plant Association | Number of records | Records % | Z of Bonferroni | ||
---|---|---|---|---|---|
Expected | Observed | ||||
Toboso grassland | 93 | 42.5 | 0.135 | 0.420 | Major use |
Vine mesquite | 8 | 9.6 | 0.028 | 0.096 | Major use |
Annual grassland | 44 | 17.4 | 0.142 | 0.174 | Proportional use |
Blue grama grassland | 26 | 11.9 | 0.201 | 0.119 | Less use |
Longleaf Jointfir scrub with grassland | 35 | 14.2 | 0.415 | 0.142 | Less use |
Bare soil | 11 | 4.1 | 0.054 | 0.041 | Proportional use |
Grassland in black-tailed prairie dog colony | 2 | 0.9 | 0.026 | 0.009 | Less use |
X2 0.05 = 219.71, gl= 6 p>0.05 |
Effect of wallows on the diversity and abundance of vegetation. Twenty-seven species of annual grasses and herbs were recorded, of which 20 were found in the annual grassland association, 17 in the vine mesquite association, and 12 in the toboso grassland.
Wallowing did not affect species richness for any of the plant associations: annual grassland (x2 = 26.449, P = 0.2329), toboso grassland (x2 = 9.991, P = 0.4413) and vine mesquite (x2 = 12.018, p = 0.8463).
Seventeen plant species were present inside and outside of wallows (i. e., 67% of the total number of species). Five different species were found only inside wallows and another five, only outside of wallows.
In the annual grassland association, the plants presented a greater height outside the wallows (F = 6.50, P = 0.0121). However, no differences were found in the coverage for that association nor in any other plant variables measured in the associations inside and outside the wallows (Table 2).
Effect of wallows on soil parameters. Soluble salts. The results obtained from the measurement of FC, BD, % clay, EC and pH of each of the samples are shown in the Annex 1.
In general, the soils presented a medium and medium-high BD (1.23 g/cm3 to 1.49 g/cm3), and the soil texture varied between loam, silty clay loam and silty clay. Annual grasses grew on soils having less clay, while toboso and vine mesquite associations grew on more clayed soil. The pH corresponded mainly to acid values and the EC pF1.8 fluctuated in a range from 0.57 to 2.00 dS/m. Based on the results of X2 of the multivariate analysis of principal components, it is shown that only the first three components present significant differences (Table 3), but with the first two 81.5 % of the variability of the observations can be explained. The first component (FC, BD, % clay) explains 57.7 % of the variability and the second (EC pF1.8 and pH) 23.8 % (Table 3; Figure 4). The differentiation of the association of annual grassland was mainly due to BD, while the associations of toboso grassland and vine mesquite were more related to the rest of the variables, mainly the percentage of clay, FC and pH (Figure 4). The minimum spanning tree shows that samples a and b from the same point do not necessarily have the greatest relationship between them.
Table 2 Analysis of variance between treatments (inside and outside of the wallows) and vegetation variables (height and cover).
df | Sum of squares | Mean squares | F | p | ||
---|---|---|---|---|---|---|
Coverage | ||||||
Toboso grassland | Treatment | 1 | 0.014 | 0.014 | 0.02 | 0.887 |
Error | 63 | 44.748 | 0.71 | |||
Total | 64 | 44.762 | ||||
Annual grassland | Treatment | 1 | 0.047 | 0.047 | 0.286 | 0.594 |
Error | 117 | 19.177 | 0.164 | |||
Total | 118 | 19.224 | ||||
Vine mesquite | Treatment | 1 | 0.004 | 0.004 | 0.007 | 0.933 |
Error | 92 | 51.708 | 0.562 | |||
Total | 93 | 51.712 | ||||
Height | ||||||
Toboso grassland | Treatment | 1 | 54.976 | 54.976 | 1.554 | 0.217 |
Error | 63 | 2,228.277 | 35.369 | |||
Total | 64 | 2,283.254 | ||||
Annual grassland | Treatment | 1 | 130.028 | 130.028 | 6.503 | 0.012* |
Error | 117 | 2,339.325 | 19.994 | |||
Total | 118 | 2,469.353 | ||||
Vine mesquite | Treatment | 1 | 13.743 | 13.743 | 0.218 | 0.641 |
Error | 92 | 5,788.396 | 62.917 | |||
Total | 93 | 5,802.138 |
*Significant differences between treatments
Bulk density (BD) and volumetric moisture content. Based on the laboratory analysis, significant differences were found in soil moisture (H = 247.10, P = <0.0001) and soil BD (H = 157.63, P = <0.0001) between the different plant associations preferred for wallowing. The BD was higher in the annual grassland (1.49 g / cm3) followed by the association of vine mesquite (1.30 g / cm3), and the toboso grassland presented the lowest bulk density in the soil (1.23 g / cm3). The vine mesquite association presented the highest soil moisture content (10.5 %), followed by toboso grassland (7.6 %) and annual grassland (4.8 %).
No significant differences were found in soil moisture inside and outside of the wallows in the vine mesquite association (H = 1.98, P = 0.5764). For the association of annual grassland, significant differences were found based on depth but regardless of the location (inside or outside of the wallow; H = 14.51, P = 0.0023), since higher moisture contents were measured at a depth of 8 to 13.5 cm (inside = 5.4 %; outside = 4.9 %) than in the first few centimeters of the soil (inside = 4.7 %; outside = 4.3 %). On the other hand, significant differences were found in the association of toboso grassland (H = 9.65, P = 0.0218). A higher soil moisture was determined inside the wallow, at both depths (surface = 8.1 %; 8-13.5 cm = 8.6 %), than outside it (surface = 6.5 %; 8-13.5 cm = 7.3 %, Figure 5).

Figure 4 Minimum path tree. An (Annual grassland), Pan (Vine mesquite), Tb (Toboso grassland); a (inside), b (outside).
Significantly higher values of BD were measured within the wallows (surface = 1.54 g / cm3; 8-13.5 cm = 1.54 g / cm3) than outside of these (surface = 1.42 g / cm3; 8-13.5 cm = 1.46 g / cm3) in the association of annual grassland (H = 17.33, P = 0.0007). No significant differences in BD were found inside and outside the wallows in the vine mesquite and toboso grassland associations (H = 6.50, P = 0.0896 and H = 7.58, P = 0.0554, respectively, Figure 5).
Table 3 Results of principal component analysis.
Number of component | Eigenvalue | % | Cumulative percentage | x2 | df | p |
---|---|---|---|---|---|---|
1 | 2.886 | 57.727 | 57.727 | 106.72 | 10.097 | <0.0001 |
2 | 1.188 | 23.768 | 81.494 | 55.622 | 9.276 | <0.0001 |
3 | 0.61 | 12.207 | 93.702 | 28.608 | 5.987 | <0.0001 |
4 | 0.228 | 4.569 | 98.271 | 7.16 | 3.173 | 0.0759 |
5 | 0.086 | 1.729 | 100 | 0 | 0.174 | 0.0759 |
Nutrients. Significant differences in the concentrations of nutrients in the soil were found between the different plant associations (F-value = 2.69, P = 0.0021), except for the concentration of NH4. The association of toboso grassland had the highest average concentrations of Ca, Mg, P and NH4; the vine mesquite had the highest averages in the concentrations of K and NO3 and the association of annual grassland presented the highest average in the concentration of Na. However, the concentration of phosphorous, exchangeable cations, NO3 and NH4, inside and outside the wallows, did not vary significantly (F-value = 0.04, P = 0.9998; Table 4).
Table 4 Mean values of elements by type of plant association.
Plant association/Element | Ca (cmol(+)/kg) | K (cmol(+)/kg) | Mg (cmol(+)/kg) | Na (cmol(+)/kg) | P (mg/kg) | NO3 (mg/kg) | NH4 (mg/kg) |
---|---|---|---|---|---|---|---|
Toboso grassland | 18.91* | 2.4 | 5.24* | 0.07 | 66.31* | 0.77 | 1.30* |
Vine mesquite | 12.61 | 2.69* | 4.81 | 0.10 | 46.58 | 0.89* | 1.27 |
Annual grassland | 6.10 | 1.83 | 2.72 | 0.22* | 42.55 | 0.48 | 1.05 |
* Indicates the maximum value of the mean per element.
Discussion
Location and use of the wallows. As expected, wallowing behavior was more frequent among males. The three plant associations preferred for wallowing were toboso grassland, vine mesquite and annual grassland. The first two associations are found within washes, and 52 % of the total wallows generated by bison were recorded in here (n = 219). These associations are probably selected due to more clay and silty texture, and higher moisture content in the soil which decreases its temperature. It is important to note that the sites selected to wallow within each association generally corresponded to patches of bare soil, that is, the bison do not remove the vegetation from the surface, but rather seek that the site is devoid of vegetation cover.
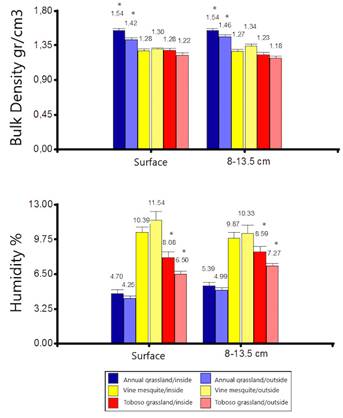
Figure 5 Bulk density (BD) and volumetric soil moisture by type of plant association and inside and outside the wallows. The bars represent the standard error and the asterisks represent significant differences between locations.
Different hypotheses have been suggested that determine the origin of the rolling behavior; it can represent aggression, dominance between males during the mating season, or grooming behavior (Coppedge and Shaw 1998). McMillan et al. (2000), analyzed the possible causes of this behavior and determined that it can be triggered by several factors, such as hair release during molt, group cohesion (mainly to strengthen ties between females and their offspring), behavior of game, thermoregulation or to reduce the burden of ectoparasites and discomfort from insect bites. In our case, we observed a significant increase in frequency during the summer, which is directly related to an increase in the temperature and humidity of the environment. These conditions favor the increase of insect populations, particularly of the horned fly (Haematobia irritans), a dipteran whose bites are irritating to bison. This fly needs a high relative humidity, an air temperature around 32 °C, and a constant source of light (Morgan 1964). Minimum temperatures should not be so low as to inhibit fly activity (such as in winter), so that in summer (July-September), when the temperature remains high (both day and night), the appropriate conditions are found increasing the activity of the horned fly. Bison appeared to be disturbed by the horned flies since they were constantly shaking the head and moving the tail when the flies surrounded them. While it is not possible to discard that ticks could be related with wallowing behavior, it is during Autumn (October) when winter tick larvae are supposed to be blood feeding (Mooring and Samuel 1998, McMillan et al. 2000), but at this time bison wallowed much lower than during other months of the year. Based on this, our results agree with McMillan et al. (2000), whose results were not consistent with the idea that wallowing is a grooming behavior to remove ticks, but to relief horn flies commonly saw during summer months. The fact that the frequency of the rolling behavior increased during the period from 10:00 to 14:00 h, particularly during the summer, when ectoparasites decrease their activity, is consistent with the hypothesis of thermoregulation as when wallowing they remove the soil surface and expose soil of a cooler temperature since a temperature decrease has been found up to 70 cm depth at noon (Singh and Sharma 2017).
Although the fact that males wallow more frequently than females during the summer could also be related to dominance among males at the time of mating, however wallowing behavior was rarely accompanied by agonistic behavior; in addition to that, competition should be reduced, since there are only three sub-adult males and there is a high availability of females. It has been reported that the parasite load of flies is related to the size of the individual, the secretion of stress hormones (cortisol) and the age of the host, which in turn reflects its health (Morgan 1964). A higher parasite load on males is consistent with our observations, and that would explain the increased wallowing. It has been observed that this behavior corresponds mainly to adult individuals. However, it was observed that calves from the first month of age try to develop it, without being able to turn on the back completely but raising their legs. It was also observed that bison frequently rubbed on the burrow mounds of prairie dogs (Cymomys ludovicianus). Sometimes they wallowed on these, but the slope was a factor for them to turn more than 180° on their backs, which appeared to frighten them, discouraging activity. Prairie dog burrow mounds, being made up of loose material, constitute a micro-site selected by bison to rub themselves, as reported by Coppedge and Shaw (2000) for coyote and badger burrows.
Effect of wallows on the richness and abundance of vegetation. When determining the effect that the wallows currently have on the richness of the vegetation, it was observed that there are no differences in the species of plants that grew during the summer inside and outside the wallows. Even though there were some species that were only recorded inside and vice versa, none had specific characteristics that prevent them from establishing inside or outside the wallows, so the differences are possibly due to sampling size rather than an effect of the wallows.
Likewise, the height and coverage variables were not influenced by the effect of the wallows. The only association that presented a difference in the height of the plants between treatments was the association of annual grasses. Under this association the highest values of BD of the soil were observed and it was the only soil variable that was significantly higher inside the wallows than outside of them. An increase in BD of the soil, implies that the pore space diminishes, affecting the aeration capacity, limiting drainage and root penetrability, thus reducing plant growth (Bartholomew and Williams 2010). An increase in BD was related with decreases the number of leaves and the biomass of the shoots and roots (Bradshaw and Chadwick 1980; Bartholomew and Williams 2010; Wilson et al. 2013). Compared to perennial grasses, the growth of annual grasses at sites with high BD could be feasible even though drainage could be reduced and roots could be limited in their ability to penetrate the soil to obtain necessary resources (Singh et al. 2018). However, the increase in BD within the wallow limited pasture growth in this association.
Effect of wallows on BD and volumetric moisture content. Contrary to our predictions, an effect of wallows on soil properties was not detected; the soils in the toboso grassland and vine mesquite associations presented a higher percentage of clays (42.5 %), because runoff water transports the suspended clays to these sites, compared to the soil present in the annual grassland association (24.2 %), and a lower bulk density. Although fine-textured soils tend to be more susceptible to compaction (Barik et al. 2011; Wilson et al. 2013), it has been found that the aggregate size groups in the coarse textured soil has a positive effect on compaction (Barik et al. 2011). Likewise, higher BD values have been reported in sandy soils due to the absence of micropores, and a relationship with the amount of water in the soil has been attributed, where a decrease in the amount of water increases the contact between the particles, increasing the cohesion forces by decreasing the water pressure in the pores (Koolen and Kuipers 1983; Barik et al. 2011). In this way, soils with fine textures that present a good degree of structure, fluvic Cambisol (hypereutric, epilymic, chromic); protovertic Phaeozem (limic, chromic), will form larger aggregates that behave as a single particle, that present greater resistance to compaction than coarse-textured soils that present few and small aggregates, Regosol haplic (hypereutric, thaptoargic).
For this reason, the wallows formed on fine-textured soils that had greater resistance to compaction did not show significant differences when comparing the inside and outside of the wallows. These differences will probably begin to become apparent over a longer period, where the wallows are used under higher humidity regimes (the clay must be at field capacity to compact), which facilitate compaction in this type of soils with low aggregate stability.
The wallows represent a concave structure that allows the accumulation of water (Polley and Collins 1984). Sapling production has been reported to increase significantly with high water potential and moderate bulk density (Tokunaga 2006). These conditions are present within the wallows in the toboso grassland and vine mesquite associations. This type of soils are less water permeable since they have finer textures, and thus presented higher moisture contents. At the Konsa Prairie Biological Station, the differential diversity of plants was evaluated, inside and outside the wallows, and certain species were only found within them (McMillan et al. 2011). During the sampling period in our study site, rainfall was below the annual mean, which did not favor the growth of the vegetation cover. For this reason, it is important to note that a longer-term sampling that includes a representation of the seasonal and annual variability of the environmental parameters would allow to determine whether there are differences attributed to the effect of wallows. During the preliminary observations in 2010 (mean annual rainfall = 279.9 mm; Unifrut 2011), the growth of a marked patch of vegetation was observed within the wallows, contrary to what was observed during the sampled year.
Effect of wallows on Nutrients. The lowest concentrations of nutrients in the annual grassland association where the soils that presented a higher percentage of sand. Nutrient retention is less and leaching tends to be faster in this type of soils since they have a low cation exchange capacity, contrary to what occurs in soils with a higher percentage of clay and silt (Whitehead 2000; Schaetzl and Anderson 2005). In some grassland sites, the losses of Ca, Mg and Na due to leaching are greater than those resulting from harvested forage (Whitehead 2000).
In this way, we would expect that the effect on the accumulation of nutrients due to the biological activities of the bison would be more evident inside the wallows of the associations of toboso grassland and vine mesquite, as during the mating season, sexually mature males urinate in the wallow before rolling to assert their dominance over other males (Gates et al. 2010). However, no significant effect of the wallows was seen.
The leaching rate is reduced when the amount of ammonia produced in the urine is used rapidly, favoring the absorption of cations by plants. The secretion of cations is greater in the urine than in the faeces, additionally; the urine macronutrients tend to infiltrate the soil faster due to the macropores (Whitehead 2000). Each individual of domestic cattle can contribute between 0.05 and 0.1 kg/m2 N in each urine stool (8 to 12 times/day) and between 0.02 and 0.08 kg/m2 N in each fecal deposition (10 times/day; Bellows 2001), and N is the element more quickly recycled in the environment because it is highly mobile in the soil solution, it leaches easily and its availability in the soil is regulated mainly by biotic processes (Wedin 1996), therefore, in order to observe differences, we need a larger number of individuals and to sample in a shorter period, between the time the individuals use the wallows and the samples are taken.
The approximate quantity of cations that are recycled in the urine and excreta of cattle, at a density of 700 ind-day / ha * year, of each macronutrient has been estimated (K = 128 kg/ha; Na = 22 kg/ha; Ca = 49 kg/ha; Mg = 24 kg/ha; Whitehead 2000). If we compare these values with the density of our study group (0.02 ind-day/ha * year), we can observe that the contribution of the recycling of macronutrients by the bison is minimal. If it is also considered that the herd was only 3 years old after being reintroduced to the site, we can conclude that indeed, we cannot expect an appreciable change in the concentration of nutrients in the soil, so we could not observe an impact of the wallows at this time. The amount of nutrients excreted is minimal and most of the cations are used by the plants or retained in the first centimeters of the soil. For this reason, we can attribute that the differences related to the type of soil are mainly due to geomorphological processes that constitute a much larger impact, both on a temporal and spatial scale, than the effect that these number of bison and for the brief period they have been in the pasture. That is, the pastures are responding to soil-geomorphology dynamics and not to the activity of the bison.
However, as the number of individuals increases in the area, it will be necessary to re-evaluate the impact that will be perceptible on a larger scale of time (10, 20, 50 years).
The limiting factor in plant growth on our site is the soil moisture, since the concentrations of nutrients are within adequate ranges to obtain maximum forage yield. In particular, K is the cation that contributes the most to maintaining the osmotic potential (opening and closing of stomata), which allows plants to adapt to drought, a very recurring factor in the study region. The values that we obtained from the K measurements far exceed the concentration value necessary to obtain the maximum yield of the forage in grasslands (> 114 mg/kg; Whitehead 2000).
Based on other studies (Polley and Collins 1984; Polley and Wallace 1986) that analyzed the effect of wallows on soil properties, differences were found inside and outside the wallows in the concentrations of NO3, NH4, P, Mg, pH, texture and soil moisture. However, these studies were conducted at a site with a larger herd (625 individuals), after a longer period (> 50-years) after the reintroduction of the herd, and in an area with higher rainfall.
Because our site is in the middle part of an intermediate-age alluvial fan, it can perform well in the growth of natural vegetation, integrating the impacts that bison activities can generate. For this reason, it is necessary to carry out a prolonged study that allows a greater representation of the environmental variability that influences the growth of vegetation and to obtain a follow-up of the changes generated in the properties of the soil and of the vegetation cover.
Wallowing represents a specific bison’s behavior and they select certain plant associations for wallowing. Wallow formation modifies soil characteristics; however, this occurs rather in the long-term, since in the studied region no significant impact on soil properties (BD, moisture, pH, EC, texture, NO3, NH4, P, Na, Mg, K, Ca) or in the vegetation (cover, high, richness) could be detected after three years of bison reintroduction. At the time of the study, soil texture seems to vary in the landscape due to geomorphological processes and influences BD, moisture and nutrient contents to a greater extent than bison activity.