Servicios Personalizados
Revista
Articulo
Indicadores
-
Citado por SciELO
-
Accesos
Links relacionados
-
Similares en SciELO
Compartir
Revista mexicana de ciencias forestales
versión impresa ISSN 2007-1132
Rev. mex. de cienc. forestales vol.14 no.79 México sep./oct. 2023 Epub 06-Oct-2023
https://doi.org/10.29298/rmcf.v14i79.1339
Scientific article
Carbon and nitrogen stock in a Cambisol soil under two land uses in Linares, Nuevo Leon, Mexico
1Facultad de Ciencias Forestales, Universidad Autónoma de Nuevo León Linares, México.
2Instituto Tecnológico de El Salto. México.
The organic Carbon of the soil is related to the physical, chemical, and biological properties of the soil, while Nitrogen is an essential macroelement in plant growth. To know the Carbon and Nitrogen stock in a Cambisol, a study was carried out in Linares, Nuevo León, Mexico. Four composite samples (n=4) (individual subsamples) were collected at five depths (0-5, 5-15, 15-30, 30-60 and 60-100 cm), in two land uses: Tamaulipan Thorny Scrub (MET) and Induced Grassland for livestock use, obtaining 40 samples in total (20 each land use); the chemical variables analyzed were soil organic Carbon percentage (COS), total Nitrogen (Nt) and the ratio C:N was estimated. Likewise, bulk density (Da) was determined to calculate the COS and Nt storage (Mg ha-1). The mean percentage of COS and Nt for the MET were 2.87 and 0.28 %, and Grassland were 2.21 and 0.21 %. Statistical analyses showed that there are differences in the COS and Nt reservoirs between land uses and depths. The COS and Nt reservoirs in the entire profile analyzed (0-100 cm) for the MET were 164.99 and 19.83 Mg ha-1, and for Grassland 146.07 and 17.81 Mg ha-1, respectively. While the average C:N ratio for the MET was 9.28 and Grassland was 10.73. Change in land use from MET to Grassland caused a decrease in the COS and Nt reservoirs of 11.47 and 10.17 %, respectively.
Key words Storage; strata; Tamaulipan Thornscrub; Grassland; soil profile; C:N ratio
El Carbono orgánico del suelo está relacionado con las propiedades físicas, químicas y biológicas del suelo, y el Nitrógeno es un macroelemento esencial en el crecimiento de las plantas. Con el fin de conocer los reservorios de Carbono y Nitrógeno en un Cambisol, se realizó un estudio en Linares, Nuevo León, México. Se recolectaron cuatro muestras compuestas (n=4) (submuestras individuales) a cinco profundidades (0-5, 5-15, 15-30, 30-60 y 60-100 cm), en dos usos del suelo: Matorral Espinoso Tamaulipeco (MET) y Pastizal inducido de uso pecuario, para un total de 40 muestras (20 por uso de suelo). Las variables químicas analizadas fueron porcentaje de Carbono orgánico del suelo (COS), Nitrógeno total (Nt), y se estimó la relación C:N. Asimismo, se determinó la densidad aparente (Da) para estimar el almacén de COS y Nt (Mg ha-1). Las concentraciones medias de COS y Nt para el MET fueron de 2.87 y 0.28 %, y para el Pastizal de 2.21 y 0.21 %, respectivamente. Los análisis estadísticos mostraron diferencias en los reservorios de COS y Nt entre usos del suelo y profundidades. Los almacenes en el perfil analizado (0-100 cm) fueron de 164.99 y 19.83 Mg ha-1 para el MET, y para Pastizal de 146.07 y 17.81 Mg ha-1, respectivamente. El promedio de la relación C:N para el MET fue de 9.28 y en Pastizal de 10.73. El cambio de uso de suelo del MET a Pastizal provocó una disminución en las reservas de COS y Nt de 11.47 y 10.17 %, respectivamente.
Palabras clave Almacén; estratos; Matorral Espinoso Tamaulipeco; Pastizal; perfil de suelo; relación C:N
Introduction
Soil is an essential resource for environmental sustainability as it is directly related to the hydrological cycle and biogeochemical cycles. However, its deterioration is increasing, due to the pressure exerted by the increase in agricultural and livestock production to satisfy food demand (Burbano-Orjuela, 2016).
One of the ecosystem services that the soil provides is Carbon capture which makes it a key factor for the mitigation of climate change (Wagner-Riddle et al., 2007). Burbano (2016) points out that soils have the ability to store up to 1 500 Pg C at 1 m deep. On the other hand, Batjes (2014) mentions that the C and N contents in the soil are a function of land use, and it is estimated that the availability of soil organic Carbon (SOC) and total Nitrogen (tN) in the first 100 cm of soil varies from 1 462 to 1 548 Pg of C and 133 to 140 Pg of tN, respectively. However, worldwide the soil is subjected to different anthropogenic activities, which are related to soil degradation and alter its capacity as a reservoir.
Land use change affects SOC reservoir and the availability of Nitrogen; these elements are essential to maintain the quality and health of the soil (Cantú and Yáñez, 2018).
It is estimated that 80 % of the Carbon that vegetation and soils exchange with the atmosphere corresponds to forests (Galicia et al., 2016). When the Carbon is incorporated in the growth of the trees, they act as sinks (2.30 Gt C yr-1) and play an important role in the Carbon balance, which contributes to reducing the carbon dioxide (CO2) content in the atmosphere from anthropogenic emissions (Pardos, 2010).
Soil Carbon and Nitrogen reserves fluctuate according to the type of soil, climatic and geographic conditions, types of ecosystems and land uses (Hume et al., 2018). In addition, they are indicators of the quality of organic matter (Luna et al., 2023). In particular, C is a key component in biogeochemical cycles, and N is in charge of defining ecosystem productivity (Di Gerónimo et al., 2018).
On the other hand, the C:N ratio is a good indicator of the quality of soil organic matter, and is associated with the speed of Nitrogen mineralization. Gamarra et al. (2018) point out that ratios between 10 and 14 are generated from rapid mineralization, which produces enough N for microorganisms, while high ratios (>20) indicate slow decomposition.
The most arid places in Mexico are covered by scrublands and grasslands, which is most of the plain of the state of Nuevo León and providing ecosystem services to more than half of the Mexican population (Briones et al., 2020). On the other hand, it is considered that the grasslands and natural habitats have a high potential of Carbon and Nitrogen reservoirs. However, most studies are focused on analyzing the superficial layers (0-40 cm), which leads to uncertainty about the behavior of Carbon and Nitrogen in deeper strata (Ward et al., 2016).
Jurado-Guerra et al. (2021) mention that pastures in Mexico occupy an approximate area of 9 million hectares, and that with a system of moderate grazing use, which on average present SOC reservoirs of 24 to 34 Mg ha-1 in the first few years 30 cm from the ground.
Based on the above, the objective of this study was to determine the effect of land use change on SOC and tN reservoirs and their distribution in different depth strata in a Cambisol.
Materials and Methods
Study area
The study area is located in the San Rafael Ejido, Linares Municipality, Nuevo León, UTM coordinates zone 14: 430500 E and 2737400 N, with 989.4 mm average annual rainfall, 21.3 °C average annual temperature and an altitude of 441 m (García, 2004). Such area belongs to the coastal plain of the North Gulf made up of gentle hills and plains (INEGI, 1986) (Figure 1).
Land uses
Tamaulipan Thorny Scrub: native vegetation of the area, made up of more than 50 tree and shrub species (1 to 5 m high), without intervention, among which prevail Havardia pallens (Benth.) Britton & Rose, Leucaena leucocephala (Lam.) de Wit, Vachellia farnesiana (L.) Wight & Arn., Yucca filifera Chabaud, Ebenopsis ebano (Berland.) Barneby & J. W. Grimes, Prosopis laevigata (Humb. & Bonpl. ex Willd.) M. C. Johnst., Cordia boissieri A. DC., among others (Patiño-Flores et al., 2022).
Induced grassland: it is an intensive grazing system, 20 years old, with rotation in pastures and a stocking rate of 8 AU ha-1, with rest periods of 8 and 5 weeks in the dry and rainy seasons, respectively.
Cambisol soil
A Cambisol soil was selected, whose name comes from the Latin Cambiare, which means to change. They are young soils in which changes in the clays between the horizons are perceived, it does not show a specific distribution in any particular type of climate, it presents moderate accumulations of iron, manganese and clay, it is characterized by good structural stability, high porosity and retention moisture, medium texture and good drainage, neutral to slightly acidic pH, with good fertility and biological activity (INEGI, 2015; IUSS, 2015).
Sample collection
Sampling was carried out in June 2021. Two 400 m2 plots were selected for each land use (MET and Grassland). Four composite samples (n=4) (individual subsamples) of 1.5 kg were collected at five depths (0-5, 5-15, 15-30, 30-60 and 60-100 cm) (Ward et al., 2016; Conafor, 2017; Lefèvre et al., 2017), which added up to 40 samples in total (20 for land use). Simultaneously, 4 undisturbed samples were extracted for the calculation of bulk density (Da, for its acronym in Spanish) at each depth, for which a 5 cm×5 cm metal cylinder was inserted (model 0200, Soilmoisture®), dried at 105 °C for 48 hours (drying oven model CE5F, Shel lab®), and subsequently weighing was carried out to determine its mass (scale model cp2202S, Sartorius®) (Blake and Hartge, 1986). Bulk density is the mass per unit volume including its pore space (Gabriels and Lobo, 2006). In this study, the bulk density was used to calculate the amount of Carbon and Nitrogen stored in the Cambisol soil.
All the samples were taken to the Laboratorio de Suelos y Nutrición de Bosques del Departamento Agroforestal (Soil and Forest Nutrition Laboratory of the Agroforestry Department) which belongs to the Facultad de Ciencias Forestales de la Universidad Autónoma de Nuevo León (Faculty of Forest Sciences of the Autonomous University of Nuevo León) for their analysis.
Analysis of chemical properties
The analysis of the organic Carbon content of the soil was determined with the wet combustion method according to Walkley-Black to obtain organic matter and applying the Van Bemmelen correction factor (Semarnat, 2002; Ramos-Hernández and Martínez-Sánchez, 2020). While the total Nitrogen was obtained by the Kjeldahl digestion method (Bremner and Mulvaney, 1982), using the model UDK159 Velp Scientifica® equipment. The C:N ratio was estimated from the SOC and tN values (Semarnat, 2002).
SOC and tN reservoir
For counting with the SOC (Mg ha-1) and tN (Mg ha-1) reservoir, the percentage of both, the bulk density (Da, g cm-3) and the soil depth stratum (Eps, cm), with the application of the following equations (González et al., 2008):
Where:
SOC = Soil organic Carbon
Da = Bulk density
Eps = Soil depth stratum
tN = Total Nitrogen
In addition, the accumulated reservoir of both variables in the entire profile (0-100 cm) was calculated from the sum of the 5 strata in each land use.
Statistical analysis
With the collected data, Kolmogorov-Smirnov normality tests and Levene homoscedasticity tests were performed (Flores and Flores, 2021). The accumulated reservoir of tN (Mg ha-1) in the 0-100 cm profile did not comply with the normality assumption, Non-parametric tests were used to compare between land uses (Mann-Whitney U) (Steel and Torrie, 1980). On the other hand, the SOC accumulated reservoir variable (Mg ha-1) in the 0-100 cm profile was analyzed with the t-Student test to detect differences between land uses (Sánchez, 2015).
Likewise, the SOC variable (Mg ha-1) by depth strata, did not meet both assumptions of normality and homogeneity of variances, so the Kruskal-Wallis test was applied to detect differences in each land use (Sánchez-López et al., 2015). While for the tN variable (Mg ha-1) by strata, an analysis of variance and its respective Tukey test were used (Gómez et al., 2019).
Regarding the C:N ratio, a t-Student test was used to compare between land uses, as well as an analysis of variance and its respective Tukey test for strata (Berlanga and Rubio, 2012). All analyzes were performed with the SPSS® program (Statistical Package for Social Sciences, SPSS), standard version 22 for Windows (IBM, 2013).
Results
The average values of the percentage of SOC and tN, as well as the bulk density for each land use (MET and Grassland) recorded in general, a decrease in the Carbon and Nitrogen percentages as the depth of the soil increases, where the strata with the highest and lowest values were observed in the 0-5 cm and 60-100 cm depths, respectively, in MET and Grassland. Bulk density showed variations between depths in each land use, in which an increase was detected in the MET and Grassland as the depth of the soil increases, with the exception of the 60-100 cm stratum in Grassland where a slight decrease is outlined (Table 1).
Table 1 Mean values of SOC, tN and bulk density for the two land uses and depth strata.
Variable | Land use | Mean (cm) | Depth | ||||
---|---|---|---|---|---|---|---|
0-5 | 5-15 | 15-30 | 30-60 | 60-100 | |||
SOC (%) | MET* | 5.99 | 5.17 | 1.53 | 0.88 | 0.78 | 2.87 |
Grassland** | 5.09 | 2.57 | 1.46 | 1.05 | 0.90 | 2.21 | |
tN (%) | MET* | 0.61 | 0.30 | 0.21 | 0.15 | 0.13 | 0.28 |
Grassland** | 0.42 | 0.18 | 0.15 | 0.17 | 0.12 | 0.21 | |
Da (g cm-3) | MET* | 0.87 | 0.97 | 1.07 | 1.12 | 1.15 | 1.04 |
Grassland** | 1.13 | 1.14 | 1.16 | 1.17 | 1.06 | 1.13 |
*MET = Tamaulipan Thorny Scrub; ** Induced Grassland of livestock use
SOC reservoir (Mg ha -1 )
The findings obtained through the t-Student test (p≤0.05) indicated that there are significant differences in the SOC reservoir between the different land uses in the profile analyzed from 0 to 100 cm deep.
The MET land use registered an average reserve of 164.99 Mg ha-1 of SOC, while the Grassland use, an average reserve of 146.07 Mg ha-1, which represents a 18.93 Mg ha-1 difference between both uses, as can be seen in Figure 2.

Figure 2 Accumulated Carbon reservoir in the 0-100 cm profile for two land uses: Tamaulipan Thorny Scrub and Induced Grassland for livestock use.
The Kruskal-Wallis test revealed that there are significant differences between the different depth strata for each of the land uses. In addition, it was identified that the SOC reservoir varied between 24.22 and 49.72 Mg ha-1 in different depth strata for the use of MET soil, while in the Grassland this variable fluctuated between 22.26 and 41.07 Mg ha-1 (Table 2).
Table 2 Average SOC reservoirs (Mg ha-1) in the different depth strata in two land uses.
Land use | Depth (cm) | Square chi | P value | ||||
---|---|---|---|---|---|---|---|
0-5 | 5-15 | 15-30 | 30-60 | 60-100 | |||
MET* | 25.89 | 49.72 | 24.22 | 29.26 | 35.91 | 12.75 | 0.013*** |
Grassland** | 22.36 | 24.31 | 23.21 | 35.12 | 41.07 | 14.47 | 0.006*** |
*MET = Tamaulipan Thorny Scrub; **Induced Grassland of livestock use. ***Significant differences among depths (Kruskal-Wallis p≤0.05).
tN reservoir (Mg ha -1 )
The Mann-Whitney U test (p≤0.05) yielded results that indicate that there are significant differences in the accumulated tN reservoir between the different land uses. The MET land use registered an average reserve of 19.83 Mg ha-1, while that of Grassland had an average reserve of 17.81 Mg ha-1, which represents a difference of 2.02 Mg ha-1 between both uses (Figure 3).
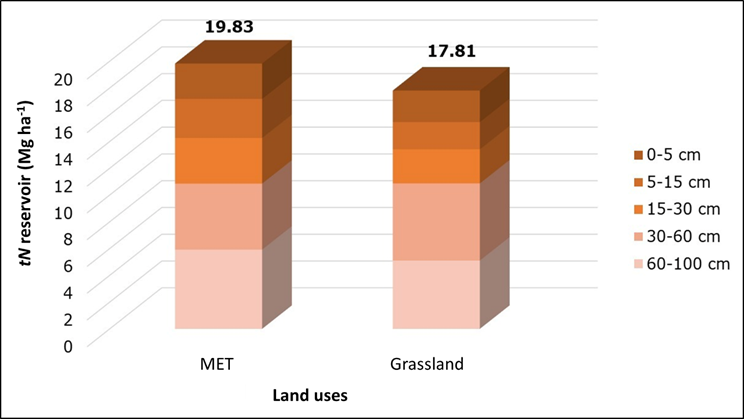
Figure 3 Accumulated Nitrogen reservoir in the 0-100 cm profile for two land uses: Tamaulipan Thorny Scrub (MET) and Induced Grassland for livestock use.
The analysis of variance for the tN reservoir indicated that there are differences between the depth strata in both land uses. Tukey's test revealed that the first three strata of the profile, where the lowest Nitrogen reserves are located, do not show significant differences between them in both land uses. In addition, the tN reservoir in the MET varied between 2.64 and 5.92 Mg ha-1 in the different depth strata, while for the Grassland, between 2.03 and 5.76 Mg ha-1 (Table 3).
Table 3 Average tN (Mg ha-1) reservoirs in different depth strata by land use.
Land use | Depth (cm) | ||||
---|---|---|---|---|---|
0-5 | 5-15 | 15-30 | 30-60 | 60-100 | |
MET* | 2.64b | 2.92b | 3.41b | 4.93a | 5.92a |
Grassland** | 2.36b | 2.03b | 2.55b | 5.76a | 5.11a |
*MET = Tamaulipan Thorny Scrub; ** Induced Grassland of livestock use. Means with different letters are statistically different (Tukey p≤0.05).
C:N ratio
The C:N ratio did not show significant differences between land uses; the averages of the C:N ratio for MET was 9.28 and for Grassland it was 10.73. However, differences were detected in the analysis of variance between the different depth strata for each land use. Tukey's test indicated that the C:N ratio is statistically the same in the last three strata in both MET and Grassland. According to Figure 4, the highest and lowest C:N ratios occurred in the same depth strata (5-15 cm and 30-60 cm) in the two land uses: in the MET it was 17.2 and 5.9, and in the Grassland of 16.3 and 7.0, respectively.
Discussion
Carbon and Nitrogen reservoirs are affected by changes in land use due to the different practices implemented, as well as changes in biogeochemical cycles (Cocotle et al., 2022). In this sense, Franco et al. (2015) stated that the change in land use from native vegetation to grasslands entails a 37 % decrease in SOC and 43 % in tN. However, the results of this investigation indicate that the reduction of these variables was 23 and 26 %, respectively. Likewise, Piñeiro (2009) points out that grazing interrupts the nutrient cycle due to the consumption of the biomass area, which results in decreases in C and N in the soil. Although there are differences between land uses, they are not so noticeable since the accumulation of feces and urine from cattle are a secondary source of C and N deposits (Ortiz, 2021). According to Ward et al. (2016), intensive grazing has a direct effect on SOC contents, and indicates that SOC decreases as depth increases; the highest concentrations are verified in the first 7.5 cm, which agrees with this investigation in which the highest concentration of SOC was recorded in the 0-5 cm depth.
Muñoz-Rojas et al. (2015) point out that the change in land use can generate SOC losses of up to 84 %. In particular, the conversion from MET to Grassland produces a loss of 50 %, mainly in the first 30 cm (Montaño et al., 2016). Results with a similar trend (31 %) were obtained in the present study. However, Campo et al. (2016) point out that said conversion can increase the SOC reservoir in the grasslands, depending on management, a situation that was observed in this study for the last two strata, where the Grassland accumulated 14.4 % more Carbon than the MET. For this reason, Conant et al. (2001) mention that the inclusion of management practices such as fertilization, type of grazing, addition of native vegetation, introduction of legumes and grasses, macro and decomposing microorganisms, and irrigation can increase said reservoir. This can be attributed to the fact that the Scrub presents a greater capacity to accumulate Carbon in the upper layer of the soil due to the greater density of vegetation. However, the Grassland has a greater capacity to store Carbon due to the structure and constant renewal of its roots that can reach deeper layers of the soil.
The depth of the soil plays a very important role in the tN percentages, since the largest number of microorganisms responsible for Nitrogen fixation and decomposition of organic matter, have their greatest activity in the superficial layer and the tN content is greater in depth decreases (Madrigal et al., 2019). As observed in each land use in this study, the 0-5 cm depth presented the highest percentages of tN, while the 60-100 cm depth had the lowest percentages. Likewise, the vegetation cover has a strong influence with high tN percentages, mainly in the first centimeters (Díaz et al., 2021).
These results coincide with those observed in the present investigation when finding a higher percentage of tN in the MET and lower in the Pastizal. These data suggest that the change in land use from Scrub to Pasture had a different effect on nitrogen content throughout the soil profile. In the strata analyzed, the percentage of Nitrogen was higher in MET than in the Grassland, except for the 30-60 cm stratum.
Bulk density has a direct effect on the percentages of SOC and tN, since it can limit the penetration of roots in deeper zones (de Moraes et al., 2020), and therefore, reduces the sources of organic matter. This coincides with the tendency of the percentage of Carbon and Nitrogen in both land uses in the present study, in which bulk density increases as the depth of the soil increases.
The ratio (C:N) in the 1 m profile in the two land uses (MET and Grassland) resulted in average values of 9.3 and 10.73, respectively. According to Porta et al. (2014), Gamarra et al. (2018) and Cantu and Luna (2022), C:N ratios between 10 and 14 show optimal mineralization of organic matter. In this study, the calculated values are above the optimal conditions (10-14) for the right activity of soil microorganisms. In this regard, Yáñez et al. (2017) recorded different soil respiration rates between MET and Grassland in a Vertisol -soil respiration is an indicator of the activity of microorganisms-. The land use with the highest CO2 emission was MET (6.17 µmol CO2 m-2 s-1) compared to Grassland (4.61 µmol CO2 m-2 s-1), which are the most common land uses in northeastern Mexico.
The MET showed a greater capacity to store Nitrogen, which can be attributed to the fall of leaf litter from the trees and the presence of legumes in symbiotic association with microorganisms that fix Nitrogen to the soil, while the roots of the pivot trees cross deeper layers and contribute to the nutrient cycle (Díaz et al., 2021).
If the soils at the National level store 56.1 Mg ha−1 and at the state level in Nuevo León 36.9 Mg ha−1 in a depth stratum of 0-20 cm, the average capacity of the Cambisol Carbon reservoir between both land uses (70 Mg ha-1) can be valued as high (Segura-Castruita et al., 2005).
In general, Cambisol offers a good SOC and tN reservoir capacity, if an average of both land uses is calculated, and a total of 155.53 and 18.82 Mg ha-1 is obtained at 1 m deep, respectively. Based on the above, the 30-100 cm stratum of Cambisol accumulated 39.5 % of the total SOC in MET and 52.2 % in Grassland. Likewise, in the case of the tN reservoir, the importance of the 30-100 cm stratum is even greater, since it accumulated 54.8 % in MET and 61 % in Grassland. This confirms the importance of investigating the entire soil profile in studies of Carbon and tN reservoirs and not only concentrating on the 0-30 cm stratum, where most of the SOC and tN are believed to be found.
Conclusions
The change in land use due to the conversion of the Tamaulipan Thorny Scrub to Grassland significantly affects the Carbon and Nitrogen reservoirs in Cambisol, which causes a decrease of 11.47 and 10.17 %, respectively.
The Cambisol has a good reservoir capacity of SOC and tN in the 1 m deep profile. The tN (Mg ha-1) reserves increase as the depth increases, and are mainly concentrated in the last two depth strata. The 30-100 cm layer is as important, or more, than the 0-30 cm layer with respect to the capacity to store SOC and tN.
The C:N ratio was maintained in an optimal interval of mineralization between the land uses and depth strata and was not affected by the land use change. The above described indicates that the MET and the Grassland have an important influence on the dynamics of Carbon and Nitrogen of Cambisol and, therefore, it is important to consider this in planning and sustainable soil management.
Acknowledgements
The authors thank the owners of the properties of the San Rafael Ejido, Linares, Nuevo León, and Conacyt for the scholarship granted to the first author to carry out such research.
REFERENCES
Batjes, N. H. 2014. Total carbon and nitrogen in the soils of the world. European Journal of Soil Science 65(1):10-21. Doi: 10.1111/ejss.12114_2. [ Links ]
Berlanga S., V. y M. J. Rubio H. 2012. Clasificación de pruebas no paramétricas. Cómo aplicarlas en SPSS. REIRE Revista d'Innovació i Recerca en Educació 5(2):101-113. Doi: 10.1344/reire2012.5.2528. [ Links ]
Blake, G. R. and K. H. Hartge. 1986. Bulk Density. In: Klute, A. (Edit.). Methods of soil analysis. Part 1: Physical and Mineralogical Methods. American Society of Agronomy, Inc. and Soil Science Society of America, Inc. Madison, WI, United States of America. pp. 363-382. [ Links ]
Bremner, J. M. and C. S. Mulvaney. 1982. Nitrogen-Total. In: Page, A. L., R. H. Miller and D. R. Keeney (Eds.). Methods of soil analysis part 2: Chemical and microbiological properties. American Society of Agronomy and Soil Science Society of America. Madison, WI, United States of America. pp. 595-624. [ Links ]
Briones, O., A. Flores-Martínez, A. E. Castellanos, Y. Perroni y A. Hernández-Guerrero. 2020. Población, servicios ecosistémicos, ciclo del carbono y políticas públicas en las zonas secas de México. Elementos para Políticas Públicas 4(2):79-97 Elementos para Políticas Públicas 4(2):79-97 https://www.elementospolipub.org/ojs/index.php/epp/article/view/36/34 . (1 de abril de 2023). [ Links ]
Burbano O., H. 2016b. El Carbono orgánico del suelo en el ámbito de la naturaleza y la sociedad. Suelos Ecuatoriales 46(1-2):89-100. http://unicauca.edu.co/revistas/index.php/suelos_ecuatoriales/article/view/62 . (23 de febrero de 2023). [ Links ]
Burbano-Orjuela, H. 2016. El suelo y su relación con los servicios ecosistémicos y la seguridad alimentaria. Revista de Ciencias Agrícolas 33(2):117-124. Doi: 10.22267/rcia.163302.58. [ Links ]
Campo, J., F. García O., A. Navarrete S. y C. Siebe. 2016. Almacenes y dinámica del carbono orgánico en ecosistemas forestales tropicales de México. Terra Latinoamericana 34:31-38. https://www.scielo.org.mx/scielo.php?pid=S0187-57792016000100031&script=sci_arttext . (23 de febrero de 2023). [ Links ]
Cantú S., I. y E. O. Luna R. 2022. Reservorio de Nitrógeno y relación C:N de un Umbrisol bajo manejo forestal en Durango, México. Revista Mexicana de Ciencias Forestales 13(72):82-111. Doi: 10.29298/rmcf.v13i72.1055. [ Links ]
Cantú S., I. y M. I. Yáñez D. 2018. Efecto del cambio de uso de suelo en el contenido del carbono orgánico y nitrógeno del suelo. Revista Mexicana de Ciencias Forestales 9(45):122-150. Doi: 10.29298/rmcf.v9i45.138. [ Links ]
Cocotle R., L., M. Ramírez S. y B. Hernández C. 2022. Impacto en la calidad del suelo mediada por el cambio de uso en el Cofre de Perote, México. Revista de la Alta Tecnología y Sociedad 14(1):1-8. https://static1.squarespace.com/static/55564587e4b0d1d3fb1eda6b/t/61f477076f645a77f2d7ac45/1643411208182/T207CocotleRomero+-+ATS2022+-+1-8.pdf . (26 de marzo de 2023). [ Links ]
Comisión Nacional Forestal (Conafor). 2017. Inventario Nacional Forestal y de Suelos. Procedimientos de muestreo Versión 19.0. Conafor. Zapopan, Jal., México. 311 p. https://www.conafor.gob.mx/apoyos/docs/externos/2022/DocumentosMetodologicos/2019/ANEXO_Procedimientos_de_muestreo_2019.pdf . (25 de marzo de 2023). [ Links ]
Conant, R. T., K. Paustian and E. T. Elliott. 2001. Grassland management and conversion into grassland: effects on soil carbon. Ecological Applications 11(2):343-355. Doi: 10.1890/1051-0761(2001)011[0343:GMACIG]2.0.CO;2. [ Links ]
de Moraes, M. T., H. Debiasi, J. C. Franchini, A. Antunes M., … and A. Schnepf. 2020. Soil compaction impacts soybean root growth in an Oxisol from subtropical Brazil. Soil and Tillage Research 200:104611. Doi: 10.1016/j.still.2020.104611. [ Links ]
Di Gerónimo, P. F., C. C. Videla y P. Laclau. 2018. Distribución de Carbono y Nitrógeno orgánico en fracciones granulométricas de suelos bajo pastizales, agricultura y forestaciones. Ciencia del Suelo 36(1):11-22. http://www.scielo.org.ar/scielo.php?script=sci_arttext&pid=S1850-20672018000100002 . (23 de febrero de 2023). [ Links ]
Díaz L., M. I., C. C. Gamarra L., M. L. Vera de Ortiz y A. V. Santa Cruz E. 2021. Contenido de Nitrógeno en suelos de sistemas silvopastoriles de Prosopis spp. del Chaco Central paraguayo. Revista Cubana de Ciencias Forestales 9(2):226-240. http://scielo.sld.cu/scielo.php?script=sci_arttext&pid=S2310-34692021000200226 . (24 de febrero de 2023). [ Links ]
Flores T., C. E. y K. L. Flores C. 2021. Pruebas para comprobar la normalidad de datos en procesos productivos: Anderson-Darling, Ryan-Joiner, Shapiro-Wilk y Kolmogórov-Smirnov. Societas Revista de Ciencias Sociales y Humanísticas 23(2):83-106. https://revistas.up.ac.pa/index.php/societas/article/view/2302/2137 . (25 de marzo de 2023). [ Links ]
Franco, A. L. C., M. R. Cherubin, P. S. Pavinato, C. E. P. Cerri, … and C. C. Cerri. 2015. Soil carbon, nitrogen and phosphorus changes under sugarcane expansion in Brazil. Science of the Total Environment 515-516:30-38. Doi: 10.1016/j.scitotenv.2015.02.025. [ Links ]
Gabriels, D. y D. Lobo L. 2006. Métodos para determinar granulometría y densidad aparente del suelo. Venesuelos 14(1):37-48. http://saber.ucv.ve/ojs/index.php/rev_venes/article/view/982 . (23 de marzo de 2023). [ Links ]
Galicia, L., A. M. Gamboa C., S. Cram, B. Chávez V., … y C. Siebe. 2016. Almacén y dinámica del carbono orgánico del suelo en bosques templados de México. Terra Latinoamericana 34:1-29. https://www.scielo.org.mx/scielo.php?script=sci_arttext&pid=S0187-57792016000100001 . (22 de junio de 2023). [ Links ]
Gamarra L., C. C., M. I. Díaz L., M. Vera de Ortíz, M. P. Galeano y A. J. N. Cabrera C. 2018. Relación carbono-nitrógeno en suelos de sistemas silvopastoriles del Chaco paraguayo. Revista Mexicana de Ciencias Forestales 9(46):4-25. Doi: 10.29298/rmcf.v9i46.134. [ Links ]
García, E. 2004. Modificaciones al sistema de clasificación climática de Köppen para adaptarlo a las condiciones de la República Mexicana. Instituto de Geografía de la Universidad Nacional Autónoma de México. Coyoacán, México D. F., México. 98 p. http://www.publicaciones.igg.unam.mx/index.php/ig/catalog/view/83/82/251-1 . (25 de marzo de 2023). [ Links ]
Gómez Q., D., J. Salazar O. y A. Vargas M. 2019. Impacto del desbalance en los tamaños de muestra por tratamiento sobre el desempeño de la prueba de comparaciones múltiples de Tukey. SERENGUETI Revista de Estadística 1(2):38-45. https://serengueti.fce.ucr.ac.cr/media/attachments/2021/12/15/revista-serengueti---vol.1---no.2---dic.-2019.pdf#page=38 . (25 de marzo de 2023). [ Links ]
González-Molina, L., J. D. Etchevers-Barra y C. Hidalgo-Moreno. 2008. Carbono en suelos de ladera: factores que deben considerarse para determinar su cambio en el tiempo. Agrociencia 42(7):741-751. https://www.scielo.org.mx/scielo.php?script=sci_arttext&pid=S1405-31952008000700001 . (23 de febrero de 2023). [ Links ]
Hume, A. M., H. Y. H. Chen and A. R. Taylor. 2018. Intensive forest harvesting increases susceptibility of northern forest soils to carbon, nitrogen and phosphorus loss. Journal of Applied Ecology 55(1):246-255. Doi: 10.1111/1365-2664.12942. [ Links ]
Instituto Nacional de Estadística y Geografía (INEGI). 2015. Guía para la interpretación de la cartografía: Edafología: Escala 1:250 000 Serie III. INEGI. Aguascalientes, Ags., México. 60 p. https://www.inegi.org.mx/contenidos/productos/prod_serv/contenidos/espanol/bvinegi/productos/nueva_estruc/702825076221.pdf . (25 de marzo de 2023). [ Links ]
Instituto Nacional de Estadística, Geografía e Informática (INEGI). 1986. Síntesis Geográfica del Estado de Nuevo León. INEGI. Benito Juárez, México D. F., México. 171 p. [ Links ]
International Business Machines (IBM). 2013. IBM SPSS Statistics for Windows, Version 22.0. Armonk, NY, United States of America. IBM Corp. https://www.ibm.com/support/pages/spss-statistics-220-available-download . (20 de marzo de 2023). [ Links ]
International Union of Soil Sciences (IUSS). 2015. World reference base for soil resources 2014. International soil classification system for naming soils and creating legends for soil maps. Food and Agriculture Organization of the United Nations (FAO). Rome, RM, Italy. 203 p. [ Links ]
Jurado-Guerra, P., M. Velázquez-Martínez, R. A. Sánchez-Gutiérrez, A. Álvarez-Holguín, … y M. G. Chávez-Ruíz. 2021. Los pastizales y matorrales de zonas áridas y semiáridas de México: Estatus actual, retos y perspectivas. Revista Mexicana de Ciencias Pecuarias 12(3):261-285. Doi: 10.22319/rmcp.v12s3.5875. [ Links ]
Lefèvre, C., F. Rekik, V. Alcantara y L. Wiese. 2017. Carbono Orgánico del Suelo: el potencial oculto. Organización de las Naciones Unidas para la Alimentación y Agricultura (FAO). Roma, RM, Italia. 77 p. https://bibliotecadigital.ciren.cl/bitstream/handle/20.500.13082/29070/CarbonoOrganicodelSuelo.pdf?sequence=1&isAllowed=y . (28 de marzo de 2023). [ Links ]
Luna R., E. O., I. Cantú-Silva and S. J. Bejar P. 2023. Soil Organic carbon changes in an umbrisol under different silvicultural treatments in a temperate forest in northwestern Mexico. Journal of Sustainable Forestry 42(4):1-16 Doi: 10.1080/10549811.2022.2043904. [ Links ]
Madrigal R., S., D. C. Acevedo, E. Hernández A. y J. L. Romo L. 2019. Influencia de la cobertura, pendiente y profundidad, sobre el Carbono y Nitrógeno del suelo. Revista Mexicana de Ciencias Forestales 10(51):201-223. Doi: 10.29298/rmcf.v10i51.113. [ Links ]
Montaño, N. M., F. Ayala, S. H. Bullock, O. Briones, … y E. Yépez. 2016. Almacenes y flujos de Carbono en ecosistemas áridos y semiáridos de México: Síntesis y perspectivas. Terra Latinoamericana 34(1):39-59. https://www.redalyc.org/pdf/573/57344471003.pdf . (23 de febrero de 2023). [ Links ]
Muñoz-Rojas, M., A. Jordán, L. M. Zavala, D. De la Rosa., S. K. Abd-Elmabod and M. Anaya-Romero. 2015. Impact of land use and land cover changes on organic carbon stocks in Mediterranean soils (1956-2007). Land Degradation and Development 26(2):168-179. Doi: 10.1002/ldr.2194. [ Links ]
Ortiz C., A. I. 2021. Transformación de carbono y nitrógeno en suelos con pastoreo bufalino y vacuno en la zona sur del estado de Veracruz. Tesis de Maestría en Ciencias en Ecología y Biotecnología. Instituto de Biotecnología y Ecología Aplicada, Universidad Veracruzana. Xalapa, Ver., México. 57 p. https://cdigital.uv.mx/bitstream/handle/1944/50849/OrtizCarmonaAmerica.pdf?sequence=1 . (26 de marzo de 2023). [ Links ]
Pardos, J. A. 2010. Los ecosistemas forestales y el secuestro de Carbono ante el calentamiento global. Instituto Nacional de Investigación y Tecnología Agraria y Alimentaria y Ministerio de Ciencia e Innovación. Madrid, MD, España. 253 p. [ Links ]
Patiño-Flores, A. M., E. Alanís-Rodríguez, V. M. Molina-Guerra, E. Jurado, … y A. Collantes-Chávez-Costa. 2022. Regeneración natural en un área restaurada del matorral espinoso tamaulipeco del Noreste de México. Ecosistemas y Recursos Agropecuarios 9(1):e2853. Doi: 10.19136/era.a9n1.2853. [ Links ]
Piñeiro, G. 2009. Efectos de la ganadería sobre la materia orgánica del suelo en los pastizales del Río de la Plata. In: Morón, A. (Coord.). Efectos de la Agricultura, la Lechería y la Ganadería en el Recurso Natural Suelo: Impactos y Propuestas. Instituto Nacional de Investigación Agropecuaria (INIA) La Estanzuela. Montevideo, MO, Uruguay. pp. 55-59. http://www.inia.uy/Publicaciones/Documentos%20compartidos/112761270809100748.pdf#page=59 . (26 de marzo de 2023). [ Links ]
Porta, J., M. López-Acevedo y R. M. Poch. 2014. Edafología: uso y protección de suelos. Ediciones Mundi-Prensa. Madrid, MD, España. 608 p. https://books.google.com.ec/books?id=7x1fAwAAQBAJ&printsec=copyright#v=onepage&q&f=false . (25 de marzo de 2023). [ Links ]
Ramos-Hernández, E. y J. L. Martínez-Sánchez. 2020. Almacenes de biomasa y carbono aéreo y radicular en pastizales de Urochloa decumbens y Paspalum notatum (Poaceae) en el sureste de México. Revista de Biología Tropical 68(2):440-451. Doi: htt10.15517/rbt.v68i2.37395. [ Links ]
Sánchez T., R. A. 2015. Prueba de Wilcoxon-Mann-Whitney: mitos y realidades. Revista Mexicana de Endocrinología, Metabolismo y Nutrición 2:18-21. https://www.revistadeendocrinologia.com/files/endocrinologia_2015_2_1_018-021.pdf . (25 de marzo de 2023). [ Links ]
Sánchez-López, D., S. R. León-Hernández y C. Barragán-Velázquez. 2015. Correlación de inteligencia emocional con bienestar psicológico y rendimiento académico en alumnos de licenciatura. Investigación en Educación Médica 4(15):126-132. https://www.scielo.org.mx/pdf/iem/v4n15/2007-5057-iem-4-15-00126.pdf . (22 de junio de 2023). [ Links ]
Secretaría de Medio Ambiente y Recursos Naturales (Semarnat). 2002. NOM-021-RECNAT-2000, Que establece las especificaciones de fertilidad, salinidad y clasificación de suelos. Estudio, muestreo y análisis. Diario Oficial de la Federación, Segunda Sección. pp 1-73. http://www.ordenjuridico.gob.mx/Documentos/Federal/wo69255.pdf . (23 de febrero de 2023). [ Links ]
Segura-Castruita, M. A., P. Sánchez-Guzmán, C. A. Ortiz-Solorio y M. C. Gutiérrez-Castorena. 2005. Carbono orgánico de los suelos de México. Terra Latinoamericana 23(1):21-28. https://www.redalyc.org/pdf/573/57323103.pdf . (26 de marzo de 2023). [ Links ]
Steel, R. G. D. and J. H. Torrie. 1980. Principles and procedures of statistics. A biometrical approach. McGraw-Hill Book Company. New York, NY, United States of America. 633 p. [ Links ]
Wagner-Riddle, C., A. Furon, N. L. Mclaughlin, I. Lee, … and J. Warland. 2007. Intensive measurement of nitrous oxide emissions from a corn soybean-wheat rotation under two contrasting management systems over 5 years. Global Change Biology 13(8):1722-1736. Doi: 10.1111/j.1365-2486.2007.01388.x. [ Links ]
Ward, S. E., S. M. Smart, H. Quirk, J. R. B. Tallowin, … and R. D. Bardgett. 2016. Legacy effects of grassland management on soil carbon to depth. Global Change Biology 22(8):2929-2938. Doi: 10.1111/gcb.13246. [ Links ]
Yáñez D., M. I., I. Cantú S., H. González R., J. G. Marmolejo M., E. Jurado y M. V. Gómez M. 2017. Respiración del suelo en cuatro sistemas de uso de la tierra. Revista Mexicana de Ciencias Forestales 8(42):123-149. Doi: 10.29298/rmcf.v8i42.22. [ Links ]
Received: February 23, 2023; Accepted: June 13, 2023