Serviços Personalizados
Journal
Artigo
Indicadores
-
Citado por SciELO
-
Acessos
Links relacionados
-
Similares em SciELO
Compartilhar
Revista mexicana de ciencias forestales
versão impressa ISSN 2007-1132
Rev. mex. de cienc. forestales vol.9 no.50 México Nov./Dez. 2018
https://doi.org/10.29298/rmcf.v9i50.239
Articles
Weathering and process effects on physical, mechanical and energy characteristics of briquettes
1Instituto de Silvicultura e Industria de la Madera, Universidad Juárez del Estado de Durango. México.
2Facultad de Ciencias Forestales, Universidad Autónoma de Nuevo León. México.
3Facultad de Ciencias Biológicas, Universidad Autónoma de Nuevo León. México.
4Facultad de Ingeniería en Tecnología de la Madera, Universidad Michoacana de San Nicolás de Hidalgo. México.
Forest industries produce sawdust waste that is not used and which may accumulate for years, becoming a risk of fire and a potential source of pollution. The main objectives of this study were to compare the energy properties between fresh sawdust and weathered sawdust from Pinus pseudostrobus wood and determine the physical and mechanical properties of briquettes made from both types of sawdust. The sawdust was characterized according to the particle size distribution, moisture content, volatile material, ash, and fixed carbon, in accordance with European standards UNE-EN14774-3 and UNE-EN14775. The briquettes were produced in a laboratory machine (LIPPEL) at pressures of 10-15 MPa and temperatures of 50, 70 and 90 °C. The quality of the briquettes was determined by proximal analysis, and by their physical and mechanical properties. The mean values and standard errors were calculated for all the variables of each type of sawdust, pressure and temperature. Data were statistically analyzed as a randomized complete block, and variance analyses were conducted to determine the existence of statistical differences (p< 0.05) between treatments. When statistical differences were found, Tukey tests were performed. The weathered sawdust showed the best energy properties, as it exhibited the lowest moisture content (9.12 %) and the highest fixed carbon (13.84 %); however, it had the highest percentage of ash (0.84 %). The briquettes with the best quality were obtained by using fresh sawdust at a pressure of 15 MPa and a temperature of 70 °C.
Key words: Weathering sawdust; solid biofuels; ash content; density; higher heating value; Pinus pseudostrobus Lindl
Las industrias forestales generan residuos de aserrín que son subutilizados, frecuentemente estos se acumulan durante años, lo que puede propiciar incendios y contaminación. Los objetivos de la presente investigación fueron comparar las propiedades energéticas entre el aserrín fresco e intemperizado de la madera de Pinus pseudostrobus, y determinar algunas propiedades físicas y mecánicas de briquetas elaboradas a partir de ellos. El aserrín se caracterizó según su distribución granulométrica, contenido de humedad, material volátil, cenizas y carbono fijo, de acuerdo con las normas europeas UNE-EN14774-3 y UNE-EN14775. Las briquetas se elaboraron en una máquina marca LIPPEL a presiones de 10 y 15 MPa, y temperaturas de 50, 70 y 90 ºC; su calidad se determinó mediante análisis proximales, propiedades físicas y mecánicas. Los valores medios y errores estándares se calcularon a las variables: tipo de aserrín, presión y temperatura. Los datos se analizaron estadísticamente como bloques completos al azar y se realizaron análisis de varianza para determinar la existencia de diferencias estadísticas (p<0.05) entre tratamientos. Cuando las hubo, se hicieron pruebas de Tukey. El aserrín intemperizado mostró mejores propiedades energéticas, al presentar menor porcentaje de humedad (9.12 %) y mayor contenido de carbono fijo (13.84 %); sin embargo, presentó un porcentaje superior de cenizas (0.84 %). Las briquetas con mejor calidad se obtuvieron cuando se utilizó aserrín fresco a 15 MPa de presión y temperatura de 70 °C.
Palabras clave: Aserrín intemperizado; biocombustibles sólidos; contenido de ceniza; densidad; poder calorífico; Pinus pseudostrobus Lindl
Introduction
Biomass densification is a pretreatment which allows to reduce the volume and homogenize the material in order to render it more efficient as fuel (Filbakk et al., 2011).
The global output of densified sawdust products in the form of pellets in the year 2014 was slightly over 24 Mt; Europe was the main producer, with approximately 62 %, followed by North America, with 34 % (Purohit and Chaturvedi, 2016).
On the other hand, the forest industry and forest exploitations generate residues that are underutilized; these include branches and tree tips, chips, slab wood, and sawdust. García et al. (2015) point out that the energetic potential of industrial residues amounts to 3.5 % of the total potential production of Mexico, which is 1 713 PJ (petajoules); this percentage is equivalent to 59.9 PJ. However, these residues are not efficiently utilized, and in most cases they are accumulated for years in areas close to the transformation sites (Oyedepo et al., 2014; Ekhuemelo et al., 2016), due to the lack of a market or to the remoteness of the transformation centers (Walker, 2013).
The most common option is to burn the byproducts in the open air, which causes serious problems to humans and to the environment because of the smoke generated by this practice.
A viable alternative for the use of these byproducts is the manufacture of densified products, such as pellets and briquettes. Certain studies have determined the effects of the open-air storage of sawdust at industrial sawmills on the quality of pellets (Arshadi et al., 2008); however, its impact on the quality of briquettes is limited. For this reason, the objectives of the present study were to compare the energy-producing properties of fresh sawdust with those of weathered sawdust of Pinus pseudostrobus Lindl. wood, and to determine the density, resistance to compaction, resistance to impact, and higher heating value of briquettes made with both types of sawdust.
Materials and Methods
Sawdust collection and characterization
After a year of weathering, Pinus pseudostrobus sawdust was collected in the sawmill of ejido La Luz, located in Iturbide municipality, Nuevo León, Mexico. Fresh sawdust was obtained after the sawing of logs. Both types of sawdust were conditioned to 65 % relative moisture and 20 ºC, until they reached a constant weight. The percentage of particles retained in each one of the sieves with No. 4, 6, 8, 20 and 40 meshes was determined; furthermore, the normal distribution of the particle size was estimated using the Kolmogorov-Smirnov procedure (Razali and Wah, 2011). Proximal analyses (of moisture content, volatile matter, ashes, and fixed carbon) and an analysis of the sawdust in bulk were conducted in accordance with the standards ASTM D 1762-84 (2001), UNE-EN-14774 (UNE-EN, 2010 and UNE-EN-15149-2 (UNE-EN, 2011).
Manufacture of briquettes
The briquettes were manufactured in a LippelTM laboratory briquette-producing machine, with a vertical orientation. The equipment has a solid base, with a cylinder with a 20 mm diameter and an in-built thermostat which allows to modify the temperature, and two pistons that apply the pressure and facilitate the extraction of the briquettes. Each briquette was made using 200 cm3 of sawdust; the pressures utilized were of 10 and 15 MPa, and the temperatures, of 50, 70 and 90 ºC; five briquettes with a diameter of 3.3 cm and a length of 8.0 cm were made for each treatment.
Characterization of the briquettes
Density was determined according to the standard UNE-EN-16127 (UNE-EN, 2012). The measurements were carried out 48 hours after the briquettes were manufactured, in order to allow them to stabilize so that the dilation effect would not affect the results (Križan et al., 2015).
Resistance to compaction was obtained by applying a load to the whole length of the briquette, as established by Borowski and Hycnar (2013) and according to the norm ASTM D 143-83 (1994) (Equation 1). The registered value was the force to the breaking point of the briquette; the tests were conducted in a universal Shimadzuc machine with a capacity for 100 kN.
Where:
RC = Resistance to compaction
L f = Force to the point of failure
Resistance to impact was determined by the number of pieces formed when each briquette was dropped onto the ground on three occasions, from a height of two meters (Chaiklangmuang et al., 2008; Rajaseenivasan et al., 2016) (Equation 2).
Where:
RI = Resistance to impact
NP = Number of manufactured pieces
The heating value of the sawdust and of the briquettes was calculated using the equation developed by Cordero et al. (2001), which relates the percentage of fixed carbon to that of the volatile matter (Equation 3).
Where:
SHP = Superior heating power (KJ kg-1)
FC = Fixed carbon (%)
VM = Volatile matter (%)
Statistical analyses
The normality of the data of the variables was determined using the Kolmogorov-Smirnov test (Razali and Wah, 2011). The percentage variables were transformed before the statistical analyses, with the square root of the arc sine (Steel and Torrie, 1960). A factorial design (2 × 2 × 3), in which the sources of variation were: a) type of sawdust, b) pressure, and c) temperature. Each treatment included five repeats.
A variance analysis was applied to the data with a normal distribution in order to determine the existence of statistical differences between treatments (p<0.05); when these occurred, Tukey tests were made. Whenever the distribution was not normal, the Kruskal Wallis test was carried out. All the statistical analyses were performed using the R 3.2.1 statistical package (Bolker, 2012).
Results and Discussion
Particle size
The percentages of particles between the two types of sawdust in the sieves with mesh sizes. 4, 20 and 40 were statistically different (Figure 1). The fresh sawdust exhibited higher values for particle sizes with mesh sizes 4 and 40. According to Žandeckis et al.(2014), particles in the interval of 0.1 to 0.85 mm make products with a better quality. Grover and Mishra (1996) point out that smaller particles are required in order to increase the adhesion with the larger particles. However, the mechanical resistance of the manufactured briquettes was estimated in terms of the balance in the proportion of the smaller and the larger particles.

Porcentaje = Percentage; Núm. de tamiz = Mesh size; Aserrín fresco = Fresh sawdust; Aserrín intemperizado = weathered sawdust.
Bars followed by the same letter in each sieve do not differ statistically for Tukey’s mean comparison test with a 95 % confidence interval.
Figure 1 Percentage of fresh and weathered sawdust of the sieves with mesh sizes Nos. 4 (4.76 mm), 12 (1.68 mm), 20 (0.84 mm), 40 (0.42 mm), and 60 (0.35 mm).
Proximal analyses
The moisture content was lower in weathered sawdust (Table 1), which indicates that this type absorbs less moisture under similar conditions, due to hysteresis and to oxygen emission during storage (Alakoski et al., 2016). The moisture content of both types of sawdust corresponds to the interval of 6.39 % to 12.4 % established by Ghaffar et al.(2015) and Žandeckis et al.(2014) for the manufacture of high-quality briquettes but is 15 % lower than that considered by Grover and Mishra (1996).
Table 1 Moisture content, volatile matter, ashes, and fixed carbon of fresh and weathered Pinus pseudostrobus Lindl. Sawdust.
Sawdust | Moisture content (%) | Volatile matter (%) | Ashes (%) | Fixed carbon (%) |
---|---|---|---|---|
Fresh | 9.83±0.13a | 75.74±0.90a | 0.24±0.04b | 14.19±0.92a |
Weathered | 9.12±0.12b | 73.72±0.78a | 0.83±0.05a | 16.33±0.83a |
Values followed by the same letter in each column do not differ statistically for Tukey’s mean comparison test with a 95 % confidence interval.
The percentage of volatile matter in fresh sawdust coincided with the value established by Brito and Barrichelo (1982), cited by Hansted et al. (2016), of 75 to 85 %, while that of weathered sawdust was slightly lower.
As for to the ash content, the percentage in weathered sawdust was statistically higher (p<0.05) than that found in fresh sawdust, due to the concentration of inorganic elements during the storage and handling (Thek and Obernberger, 2012; Correa-Méndez et al., 2014). The percentage of ash is an important factor to be considered during combustion at high temperatures, due to the formation of slag in the furnaces (Alakoski et al., 2016). A value of 0.83 %, registered for weathered sawdust, exceeds the accepted limit (0.50 %) for the production of briquettes (ÖNORM, 2000), while the result for fresh sawdust was similar to that cited by Correa-Méndez et al.(2014) for Pinus leiophylla Schiede. ex Schltdl. & Cham., P. montezumae Lamb. and P. pseudostrobus.
The percentage of fixed carbon in briquettes made with both types of sawdust exhibited no statistically significant differences; the value for briquettes made from both biomass sources was higher than the one cited by Freitas et al. (2016).
Briquettes
Proximal analyses. The lowest moisture content (6.63 %) was found in briquettes made with fresh sawdust at a pressure of 15 MPa and at 70 ºC (Table 2). The interaction between the sawdust type and the temperature evidenced significant statistical differences (P<0.001) (Table 3). The higher the moisture content, the greater the loss of energy was during combustion, due to water evaporation, which reduced the heating power of the material (Onyenanu et al., 2015). A low moisture content is also desirable in order to reduce the rot caused by fungi, as well as disintegration during transportation.
Table 2 Immediate analyses of briquettes made with fresh and weathered Pinus pseudotrobus Lindl. sawdust under various temperatures and pressures.
Pressure (MPa) | Temperature (°C) | Moisture content (%) | Volatile matter (%) | Ashes (%) | Fixed carbon (%) |
---|---|---|---|---|---|
Fresh sawdust | |||||
10 | 50 | 9.41±0.15a | 78.62±0.23cd | 1.02±0.13a | 10.96±0.23c |
70 | 6.76±0.16c | 79.76±0.34abcd | 0.87±0.05ab | 12.62±0.23ab | |
90 | 7.15±0.14bc | 80.87±0.24ab | 0.88±0.10ab | 11.10±0.31bc | |
15 | 50 | 9.34±0.16a | 78.75±0.45bcd | 0.90±0.09ab | 11.01±0.37c |
70 | 6.63±0.06c | 78.52±0.28cd | 0.77±0.05abc | 14.07±0.29a | |
90 | 7.39±0.27bc | 79.43±1.11abcd | 0.73±0.07bc | 11.56±0.20bc | |
Weathered sawdust | |||||
10 | 50 | 7.72±0.15b | 80.98±0.32a | 0.28±0.05e | 11.03±0.36c |
70 | 7.48±0.09bc | 81.01±0.27a | 0.26±0.05e | 11.25±0.20bc | |
90 | 8.92±0.21a | 78.52±0.26cd | 0.62±0.03cd | 11.94±0.28bc | |
15 | 50 | 7.14±0.14bc | 80.26±0.32abc | 0.28±0.01e | 12.32±0.40bc |
70 | 7.86±0.16b | 80.47±0.48abc | 0.42±0.21de | 11.25±0.28bc | |
90 | 8.86±0.42a | 77.77±0.13d | 0.77±0.07abc | 12.62±0.47ab |
Values followed by the same letter in each column exhibit no statistical difference for Tukey’s mean comparison test with a 95 % confidence interval.
Table 3 Variance analysis of the proximal analyses of briquettes made with fresh and weathered Pinus pseudotrobus Lindl. sawdust at various temperatures and pressures.
Source of variation | Moisture content | Volatile matter | Ashes | Fixed carbon | ||||
---|---|---|---|---|---|---|---|---|
F | p | F | p | F | p | F | p | |
Sawdust type (S) | 1.568 | 0.216 | 3.424 | 0.070 | 76.358 | 0.000 | 0.235 | 0.630 |
Temperature (T) | 1.705 | 0.197 | 2.174 | 0.146 | 7.793 | 0.007 | 1.964 | 0.167 |
Pressure (P) | 0.042 | 0.839 | 7.420 | 0.009 | 0.001 | 0.991 | 5.149 | 0.027 |
Interaction (S × T) | 54.605 | <0.001 | 33.935 | <0.001 | 22.377 | <0.001 | 0.135 | 0.715 |
Interaction (S×P) | 0.083 | 0.775 | 0.091 | 0.764 | 3.711 | 0.060 | 0.002 | 0.967 |
Interaction (T×P) | 0.764 | 0.386 | 1.367 | 0.248 | 0.144 | 0.706 | 0.025 | 0.874 |
Interaction (S×T×P) | 0.048 | 0.827 | 1.311 | 0.257 | 0.485 | 0.489 | 0.609 | 0.439 |
F = F value; p = Level of significance.
The highest percentage of volatile matter (81.01 %) occurred in briquettes made with weathered sawdust at a pressure of 10 MPa and a temperature of 70 ºC (Table 2). The variable pressure and the sawdust:temperature interaction exhibited statistically significant differences (p<0.05) (Table 3).
The reaction index was calculated based on the volatile matter/fixed carbon ratio, and it indicates the potential reaction of the material during pyrolysis (Purohit and Chaturvedi, 2016); therefore, the most reactive briquette was the one made with weathered sawdust at 10 MPa and 50 ºC.
The ash content exhibited significant statistical differences (p<0.05) in the variables type of sawdust, temperature and sawdust: temperature and sawdust: pressure interactions (Table 3). The fixed carbon ranged between 10.96 and 14.07 % (Table 2), which is below the 17.87 % cited by Ballarin et al. (2010) for Cupressus lusitanica Mill. sawdust briquettes.
Heating value
The heating value corresponded to the interval of 17.35 to 18.40 MJ kg-1, of which the highest was for briquettes made with fresh sawdust at a temperature of 70 °C and a pressure of 15 MPa (Figure 2). The results agree with the interval of 16.374 a 19.774 MJ kg-1 by Onyenanu et al. (2015) for briquettes made with sawdust with various particle size distributions; these values were is lower than the 19.678 MJ kg-1 indicated by Cardoso et al. (2016) for conifer sawdust briquettes, and higher than the 14.68 MJ kg-1 documented by Rahaman and Salam (2017) for rice husk briquettes.
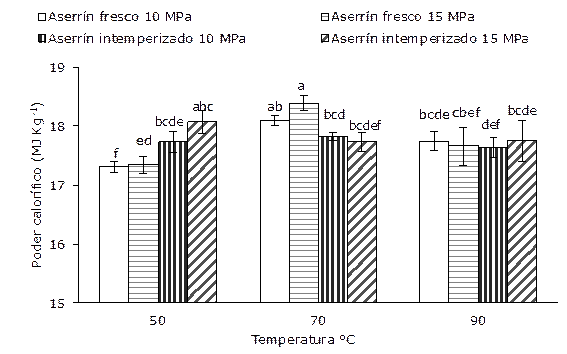
Poder calorífico = Heating value; Temperatura = Temperature; Aserrín fresco = Fresh sawdust; Aserrín intemperizado = weathered sawdust.
Bars followed by the same letter in each temperatura do not differ statistically for Tukey’s mean comparison test with a 95 % confidence interval.
Figure 2 Heating value of briquettes produced with fresh and weathered Pinus pseudostrobus Lindl. sawdust at various pressures and temperatures.
Density
There were significant statistical differences (p<0.05) in the density of the briquettes for the variables type of sawdust, temperature and sawdust:temperature:pressure interaction (Table 4). The highest density was obtained with fresh sawdust at a temperature of 70 ºC and a pressure of 15 MPa (Figure 3a). In a similar assay, Filbakket et al. (2011) reported denser pellets made with fresh sawdust. On the other hand, Silva et al. (2011) point out that different percentages in particle sizes produce differences in the density of biofuels. The higher density is also ascribed to the higher percentage of extracts in fresh sawdust, which may reduce friction during pressing, generating greater mobility of the particles and a better arrangement during pressing.
Table 4 Variance analysis of the physical and mechanical properties of briquettes made with fresh and weathered Pinus pseudotrobus Lindl. sawdust at various temperatures and pressures.
Source of variation | Density | Resistance to compaction | Resistance to impact | |||
---|---|---|---|---|---|---|
F | p | F | p | F | p | |
Type of sawdust (S) | 14.948 | <0.001 | 317.411 | 0.000 | 30.944 | <0.001 |
Temperature (T) | 17.768 | <0.001 | 17.712 | 0.000 | 22.548 | <0.001 |
Pressure (P) | 2.188 | 0.145 | 0.016 | 0.899 | 0.710 | 0.403 |
Interaction (S × T) | 2.194 | 0.145 | 0.559 | 0.458 | 3.452 | 0.069 |
Interaction (S × P) | 2.166 | 0.147 | 0.418 | 0.521 | 4.802 | 0.033 |
Interaction (T × P) | 0.046 | 0.832 | 0.000 | 0.997 | 5.157 | 0.027 |
Interaction (S × T × P) | 5.676 | 0.021 | 5.804 | 0.020 | 0.043 | 0.837 |
F = F value; p = Level of significance.

Aserrín fresco = Fresh sawdust; Aserrín intemperizado = weathered sawdust; Densidad = Density; Resistencia a la compression = Resistance to compaction; Resitencia al impacto = Resistance to impact; Número de piezas = Number of pieces.
a = Density; b = Resistance to compaction; c Resistance to impact.
Bars followed by the same letter for each sieve do not differ statistically for Tukey’s mean comparison test with a 95 % confidence interval.
Figure 3 Physical and mechanical properties of briquettes made with fresh and weathered Pinus pseudostrobus Lindl. sawdust at different pressures and temperatures.
Resistance to compaction
The resistance to compaction of the briquettes exhibited statistical differences (p<0.05) between the sawdust types, the temperature and the sawdust:temperature:pressure interaction (Table 4). Figure 3 shows that the briquettes made with fresh sawdust at 70 ºC and 15 MPa are the most resistant to compaction. The higher resistance of the fresh sawdust briquettes is the result of a greater adhesion between the particles under this condition. Another aspect that affects resistance positively is the higher percentage of particles of less than 0.42 mm (Sieve No. 40). In this regard, Mitchual et al. (2013) and Chin and Siddiqui (2000) point out that the particle size affects the mechanical durability of densified products inversely. The percentage of small particles is considered to improve the mechanical resistance up to a certain limit, after which it decreases as the resistance to impact diminishes.
Resistance to impact
The resistance to impact evidenced statistical differences between the sawdust types, temperatures, and the temperature:pressure interaction (Table 4). The fresh sawdust briquettes made at 50 °C and 10 MPa were more resistant to impact as they generated a smaller number of pieces.
Conclusions
Certain energetic quality parameters, such as the high content of volatile matter, the high reactivity index and the low content of ashes make weathered sawdust a better biofuel than fresh sawdust. However, when both sawdust types are densified under equal conditions, briquettes made with weathered material exhibit a lower quality in certain parameters than those produced with fresh sawdust.
The positive bioenergetic parameters exhibit by weathered sawdust briquettes are a lower content of moisture, fixed carbon and ashes, and a higher percentage of volatile matter. While the negative parameters are less density and a lower resistance to compaction, due to the lower percentage of small particles and to the higher production temperatures, of 70 and 90 ºC.
Notwithstanding, weathered sawdust stored at the facilities of forest industries may produce briquettes with high-quality bioenergetic parameters, such as a low content of ashes, and a high density and resistance to compaction, provided that a selection of particles is carried out, and a temperature of 70 ºC and a pressure of 10 or 15 MPa are utilized.
Acknowledgments
The authors wish to express their gratitude to the Fund for Energy Sustainability, through Project Sener Conacyt 2014 246911, Solid Biofuels Cluster for Thermal and Electrical Energy Generation, and to Conacyt Project 166444 for the support granted for conducting the present study.
REFERENCES
Alakoski, E., M. Jämsén, D. Agar, E. Tampio and M. Wihersaari. 2016. From wood pellets to wood chips, risks of degradation and emissions from the storage of woody biomass-A short review.” Renewable and Sustainable Energy Reviews (54):376-83. [ Links ]
American Society of Testing Materials (ASTM). 1994. D 143-83. 1994. Standard methods of testing small clear specimens of timber.” Num. 9. ASTM. West Conshohocken, PA, USA. 31p. [ Links ]
American Society of Testing Materials (ASTM).1990 D-1762-84. Standard method for chemical analysis of wood charcoal. Philadelphia, PA, USA. 2p. [ Links ]
Arshadi M., G. R., P. Geladi, S. A. Dahlqvist and T. Lestander. 2008. The influence of raw material characteristics on the industrial pelletizing process and pellet quality. Fuel Processing Technology 89:1442-47. [ Links ]
Ballarin, A., P. Wagner, H. Almeida, P. Lara and R. Colenci. 2010. Portable hardness tester for timber classification. In: WCTE 2010-World Conference on Timber Engineering. 20-24 June. Trentino, Italy. 8p. [ Links ]
de Brito, J. O. y L. E. G. Barrichelo. 1982. Aspectos técnicos da utilização da madeira e carvão vegetal como combustíveis. Seminário de Abastecimento Energético Industrial Com Recursos Florestais 2: 101-37. [ Links ]
Bolker, B. 2012. R Development Core Team, bbmle: tools for general maximum likelihood estimation. R package version. Vol. 1. Num. 5.2. https://cran.rproject.org/web/packages/bbmle/index.html (11 de noviembre de 2018). [ Links ]
Borowski, G. and Hycnar, J. J. 2013. Utilization of fine coal waste as a fuel briquettes. International Journal of Coal Preparation and Utilization 33(4): 194-204. [ Links ]
Chaiklangmuang, S., S. Supa and P. Kaewpet. 2008. Development of fuel briquettes from biomass-lignite blends. Chiang Mai Journal of Science 35 (1):43-50. [ Links ]
Chin, O. C. and K. M. Siddiqui. 2000. Characteristics of Some Biomass Briquettes Prepared under Modest Die Pressures. Biomass and Bioenergy 18(3): 223-28. [ Links ]
Cordero, T., F. Marquez, J. Rodriguez-Mirasol and J. J. Rodriguez. 2001. Predicting heating values of lignocellulosics and carbonaceous materials from proximate analysis. Fuel 80(11):1567-71. doi:http://dx.doi.org/10.1016/S0016-2361(01)00034-5. [ Links ]
Correa-Méndez, F., A. Carrillo-Parra, J. G. Rutiaga-Quiñones, F. Márquez-Montesino, H. González-Rodríguez, E. Jurado-Ybarra and F. Garza-Ocañas. 2014. Moisture and inorganic substance content in pine timber products for use in pellets and briquettes. Revista Chapingo Serie Ciencias Forestales y del Ambiente 20(1):77-88. doi:10.5154/r.rchscfa.2013.04.012. [ Links ]
Ekhuemelo, D. O., O. Ademu and E. Terzungwue T. 2016. Physical and strength properties of bricks produced from portland cement and saw dust of danielia oliverii wood. Pro Ligno 12(4):53-60. [ Links ]
Freitas, A. J., A. C. S. Costa, A. C. Oliveira, B. L. C. Pereira, M. F. V. Rocha e A. C. O. Carneiro. 2016. Efeito da pressão e do tempo de compactação nas propriedades de briquetes de resíduos madeireiros de paricá. Pesquisas Agrárias e Ambientais Nativa Sinop 4(6): 380-385. [ Links ]
Filbakk, T., G. Skjevrak, O. Høibø, J. Dibdiakova and R. Jirjis. 2011. The influence of storage and drying methods for scots pine raw material on mechanical pellet properties and production parameters. Fuel Processing Technology 92(5): 871-878. [ Links ]
García, C. A., E. Riegelhaupt, A. Ghilardi, M. Skutsch, J. Islas, F. Manzini and O. Masera. 2015. Sustainable bioenergy options for Mexico: ghg mitigation and costs. Renewable and Sustainable Energy Reviews 43:545-52. doi:https://doi.org/10.1016/j.rser.2014.11.062. [ Links ]
Ghaffar, S., A. Nasir, M. Azhar A. and M. Usman F. 2015. Physico-thermal characterization of biomass materials of different nature of briquettes. Journal of Agricultural Research 53(4):555-565. [ Links ]
Grover, P. D. and S. K. Mishra. 1996. Biomass Briquetting: Technology and Practices. Food and Agriculture Organization of the United Nations. Bangkok, Thailand. 43p. [ Links ]
Hansted, S. A. L., G. Tami N., M. Provedel M., H. Yamamoto and F. Minoru Y. 2016. Comparative analyses of fast growing species in different moisture content for high quality solid fuel production. Fuel 184:180-84. [ Links ]
Cardoso J. R., M. A. Brand, P. D’angelo, A. Bayestorff e G. Allegretti. 2016. Análise da qualidade wnergética da falha de pinhão para a produção de briquetes analysis of the energy quality pinion fails to produce Bbriquettes. Scientia Forestalis 44(112): 821-29. [ Links ]
Križan, P., M. Matú, Ľ. Šooš and J. Beniak. 2015. Behavior of beech sawdust during densification into a solid biofuel. Energies 8(7): 6382-98. [ Links ]
Mitchual, S. J., K. Frimpong-Mensah and N. A. Darkwa. 2013. Effect of species, particle size and compacting pressure on relaxed density and compressive strength of fuel briquettes. International Journal of Energy and Environmental Engineering 4(1):1-6. [ Links ]
Österreichisches Normungsinstitut (ÖNORM). 2000. ÖNORM 7135. Compressed wood or compressed bark in natural state, pellets and briquettes, requirements and test specifications. Österreichisches Normungsinstitut, Vienna, Austria. 10 p. [ Links ]
Onyenanu, I. U., C. E. Ilochonwu and P. N. Atanmo. 2015. Measurement of energy value on different grain sizes of sawdust briquettes. American Academic & Scholarly Research Journal 7(5) 129-135. [ Links ]
Oyedepo, O. J., S. D. Oluwajana and S. P. Akande. 2014. Investigation of properties of concrete using sawdust as partial replacement of sand. Civil and Environmental Research 6(2): 35-42. [ Links ]
Purohit, P. and V. Chaturvedi. 2016. Techno-economic assessment of biomass pellets for power generation in India. Council on Energy, Environment and Water (CEEW) Working Paper. Thapar House. New Delhi, India. 42p. [ Links ]
Rahaman, S. A. and P. A. Salam. 2017. Characterization of Cold Densified Rice Straw Briquettes and the Potential Use of Sawdust as Binder. Fuel Processing Technology 158:9-19. [ Links ]
Rajaseenivasan, T., V. Srinivasan, G. S. Mohamed Q. and K. Srithar. 2016. An Investigation on the performance of sawdust briquette blending with neem powder. Alexandria Engineering Journal 55(3):2833-38. [ Links ]
Razali, N. M. and Y. B. Wah. 2011. Power comparisons of shapiro-wilk, kolmogorov-smirnov, lilliefors and anderson-darling tests. Journal of Statistical Modeling and Analytics 2(1):21-33. [ Links ]
Silva, G. G. D., S. Guilbert and X. Rouau. 2011. Successive centrifugal grinding and sieving of wheat straw. Powder Technology 208(2):266-70. [ Links ]
Steel, R. G. D. and J. H. Torrie. 1960 Principles and Procedures of Statistics. Principles and Procedures of Statistics. McGraw-Hill. New York, NY USA. 633p. [ Links ]
Thek, G. and I. Obernberger. 2012. The pellet handbook: The production and thermal utilization of biomass pellets. Routledge. London, UK. 549p. [ Links ]
Una Norma Española-European Norm (UNE-EN). 2010. UNE-EN-14774. Biocombustibles sólidos. Determinación del contenido de humedad. Método de secado en estufa. Parte 3. H. Asociación Española de Normalización y Certificación. Madrid, España. 8p. [ Links ]
Una Norma Española-European Norm (UNE-EN). 2011. UNE-EN-15149-2. Biocombustibles sólidos. métodos para la determinación de la distribución de tamaño de partícula. Parte 2: Método del tamiz vibrante con apertura de malla inferior o igual a 3.15 mm. Grupo 12. Asociación Española de Normalización y Certificación. Madrid, España. 14 p. [ Links ]
Una Norma Española-European Norm (UNE-EN). 2012. UNE-EN-16127. Solid Biofuels-Determination of length and diameter of pellets. European Committee for Standardization: Brussels, Belgium. 11p. [ Links ]
Walker, J. C. F. 2013. Wood panels: Particleboards and fibreboards. Primary Wood Processing: Principles and Practice. Springer. London, UK. p 419.480. [ Links ]
Žandeckis, A., Fr. Romagnoli, A. Beloborodko, V. Kirsanovs, D. Blumberga, A. Menind and M. Hovi. 2014. Briquettes from mixtures of herbaceous biomass and wood: Biofuel investigation and combustion tests. Chemical Engineering Transactions 42:67-72. [ Links ]
Received: March 15, 2018; Accepted: September 28, 2018