Servicios Personalizados
Revista
Articulo
Indicadores
-
Citado por SciELO
-
Accesos
Links relacionados
-
Similares en SciELO
Compartir
Revista mexicana de ciencias forestales
versión impresa ISSN 2007-1132
Rev. mex. de cienc. forestales vol.7 no.36 México jul./ago. 2016
Articles
1 Instituto Tecnológico del Valle de Oaxaca (ITVO). México.
2 División de Estudios de Posgrado e Investigación-ITVO. México.
3 Campo Experimental Valles Centrales, CIR- Pacífico Sur. INIFAP. México.
The present study highlights the importance of generating information on forest fuels to determine the risk of forest fire in pine, oak and montane cloud forests in San Antonio, Chimalapa, Oaxaca. Its purpose was to calculate the content of aboveground biomass in the various components found on the forest floor of three vegetation types, at risk of fire. In the course of the year 2014, fifteen 1 000 m2 sites and nine 1 m2 subsites were established under supervision, in order to assess the wood, dead leaves, necromass, bushes and weeds compartments. The risk rates were determined by vegetation type. The information was validated using variance analysis and mean tests (Duncan, 0.05), under a fully randomized design. The biomass contents of the forest floor were 8.77 t has-1 for the montane cloud forest, 15.23 t has-1 for the oak forest, and 1.07 t has-1 for the pine forest. The biomass content of the weeds was higher (p = 0.009) in the pine forest than in other vegetation types. However, the total fuel load of the forest floor turned out to be between 2.0 and 2.59 t ha-1, which indicates that the risk of fire in study area is low; in contrast, the montane cloud forest had the highest risk rate.
Key words: Oak forest; pine forest; montane cloud forest; forest fuel load; dry matter compartments; Oaxaca
El presente estudio destaca la importancia de generar información de los combustibles forestales para determinar el riesgo de incendio en bosques de pino, de encino y mesófilo de montaña en San Antonio, Chimalapa, Oaxaca. Su objetivo consistió en calcular el contenido de biomasa aérea en los diferentes componentes encontrados en el piso forestal de tres tipos de vegetación, bajo índice de riesgo de incendio. Durante 2014, se establecieron de manera dirigida 15 sitios de 1 000 m2, y subsitios de 9 y 1 m2 para evaluar los compartimentos de madera, hojarasca, necromasa, arbusto y herbácea; se determinaron índices de riesgo por tipo de vegetación. La información se validó mediante análisis de varianza y pruebas de medias (Duncan, 0.05), bajo un diseño completamente aleatorizado. Los contenidos de biomasa en el piso forestal fueron de 8.77 t ha-1 para el bosque mesófilo de montaña, de 15.23 t ha-1 para bosque de encino y de 1.07 t ha-1 para bosque de pino. La biomasa contenida en herbáceas fue superior (p = 0.009) en el pinar, en comparación con los otros tipos de vegetación; sin embargo, al ponderar la carga total de combustible en el piso forestal, resultó entre 2.0 y 2.59 t ha-1, lo cual indica que el índice de riesgo de incendio forestal en el área de estudio es bajo; en el bosque mesófilo de montaña se registró el valor más alto.
Palabras clave: Bosque de encino; bosque de pino; bosque mesófilo de montaña; carga combustible forestal; compartimentos de materia seca; Oaxaca
Introduction
Forest fires are a product of the climate conditions, mainly of long draughts, of the great amount of accumulated combustible material, and of a natural (lightning and volcanism) or, when induced, a human ignition factor (Estrada and Ángeles, 2007). For this reason it is important to understand the mechanisms controlling the regimens of these accidents (Zinck et al., 2011; Rodríguez, 2012).
Forest fuel is one of the main elements involved in the occurrence of these phenomena and a key factor for determining the risk of the event, as the degree of hazard is in terms of the amount and the type of combustible material present in each of the forest areas (Rentería et al., 2005).
Because of its location, the Los Chimalapas region has mostly warm, temperate, humid, subhumid, warm humid, semiwarm subhumid and temperate subhumid climates; this is due to its relief with pronounced differences in altitude (200 to 2 000 m), orientation and topography. In this area the mean annual temperature ranges between 22 and 24 °C, and the mean annual precipitation is between 200 and 2 000 mm (Rodríguez, 2014). On the other hand, it is a complex mosaic of vegetation types, which enhances the biological value of the area, considered to be rich in biodiversity and to have a high rate of potential endemicity (Contreras, 2009). The main types of vegetation are: high, medium and low rain forests, bush, montane cloud forest, and pine, oak and pine-oak forests (Rojas and Ríos, 2012).
The most frequent causes of forest fires in the Los Chimalapas region, Oaxaca, are anthropogenic: agricultural and livestock breeding activities, intentional burnings, hunting and agrarian problems, while a low percentage of fires are attributed to natural causes (lightning) (Grupo Mesófilo A. C., 2006). In these territories, the impact has been increasingly greater in the last few years; it was most critical in 1998, when reportedly 77 fires affected 210 564 ha (Anta and Plancarte, 2001). In the municipality of San Miguel Chimalapa, the damaged area was 37 806 ha, and particularly affected areas were located, notably in the montane cloud, conifer and oak forests (Asbjornsen and Gallardo, 2004).
The anthropogenic action and climate change have altered the fire regimen in various forest ecosystems; therefore, the fuel loads (biomass) must be estimated, as they are the only factor in the fire triangle that can be manipulated (Rubio et al., 2016). This information makes it possible to implement strategies for the management and reduction of those materials that can burn in order to diminish the risk of catastrophic fires (Chávez et al., 2016). Also, the longer the forest fire interval, the more fuel will be accumulated, and therefore, the impact of a fire on these ecosystems will be greater (Xelhuantzi et al., 2011).
On the other hand, the amount and quality of the surface fuels in the forests are indicative of the conditions of vulnerabilty and the risks of fires in specific localities (Castañeda et al., 2015). The information thus generated will also contribute to make decisions regarding the management of the fire, as well as to implement preventive actions such as prescribed burnings, and for the assessment of the effects of fires on the ecosystems in the region (Rodríguez et al., 2011; Villers et al., 2013).
Based on all the former, the objective of this study was to evaluate the content of the biomass of bushes, weeds and dead leaves and the necromass on the forest floor for three vegetation types as indicators of fire risk in the forests of San Antonio, Municipality of San Miguel Chimalapa, Oaxaca.
Materials and Methods
Description of the study area
The research was developed in the locality of San Antonio, in the Eastern area of the Municipality of San Miguel Chimalapa, Juchitán, Oaxaca, between the coordinates 16°39’27.56” N and 94°1’34.74” W, at a mean altitude of 1 075 m. The climate is AC(w2)(w) semiwarm subhumid, with a relatively high humidity, summer rains with a mean annual precipitation of 576 to 3 300 mm, and a mean annual temperature of 20 °C (Rodríguez, 2014).
The vegetation associated with the oak forest is characterized by including specimens of Liquidambar styraciflua L., Pinus pseudostrobus Lindl., Quercus corrugata Hook. and Q. peduncularis, Née with a normal diameter (ND) of 20.0 ± 15.0 cm and a total height (TH) of 14.6 ± 6.2 m. The arboreal stratum of the montane cloud forest has a higher variability in ND (19.0 ± 13.0 cm) and a lower variability in TH (12.1 ± 3.4 m), due to the great diversity of the species it includes, among which the most prominent are: L. styraciflua, Cornus disciflora Moc. & Sessé ex DC., Cinnamomum effusum (Meisn.) Kosterm., Capparis sp, Ficus pertusa L. f., Podocarpus sp, Pinus pseudostrobus, Q. corrugata, Rheedia macrophylla (Mart.) Planch. & Triana, Sweetia panamensis Benth. and Ternstroemia pringlei (Rose) Standl. Finally, the pine forest, characterized by the presence of L. styraciflua, P. oocarpa, P. pseudostrobus, Q. corrugata and Q. crassifolia Humb. et Bonp., has the highest dimensions for ND (24.1 ± 18.5 cm) and TH (14.8.0 ± 6.5 m).
Site selection and biomass sampling
In 2014, several visits were made to the area with the highest frequency of fires, with a surface area of 1 200 ha, where 15 sampling sites were established under supervision based on the variation of the topographic factors and the distribution and vegetation type; contrasts in the tree density (dense, semi-dense and fragmented) were sought in areas with 387, 347 and 261 trees ha-1, respectively, according to the classification by Castañeda et al. (2015).
The sampling units of 0.1 ha were evenly distributed in three types of forests: montane cloud, pine and oak forests, based on the slope, exposure, altitude and topographic position (Figure 1).
In order to estimate the biomass on the forest floor, subsites of 9 and 1 m2 were established within the 1.0 ha sites. The site number, exposure, slope (%), geographic coordinates and altitude were registered for each parcel of 0.1 ha (the last two data -coordinates and altitude- were obtained using a Garmin eTrex 20 GPS). The trees were inventoried based on the following data: species, normal diameter (cm) measured using a caliper, and total height (m) measured with a Suunto PM5/66PC clinometer. The diameter of the bushes (<2.5 cm) was measured at soil level using a 1/128” Foy vernier. The topographic data of the sites were also verified.
In both the montane cloud and oak forests a tree of each of the species found in the 15 sites was chosen at random for destructive biomass analysis, based on the mean diameter (20 cm); in the case of the pine forest, trees with a diameter of 25 cm were felled. A slice with a thickness of approximately 5 cm and a sample of a shaving made with a Pressler drill were taken from a height of 1.30 m; both were weighed in field in order to obtain the fresh weight (g) using a CS5000 Ohaus scale with capacity for 5 kg. The samples were identified and taken to the laboratory of Instituto Tecnológico del Valle de Oaxaca (Technological Institute of the Valley of Oaxaca, ITVO) in order to estimate their fresh or green volume (GV, cm3) by the displacement-in-water method; they were then dried in a Memmert Beschickung loading model 100-800 convection oven at a temperature of ±102 °C, until the pieces of interest attained a constant weight and reached their dry weight (DW, g). The dry-weight/green volume ratio gave as a result the specific gravity (SG, g cm-3) per species, which was used to convert the tree volume, estimated based on the volume tables of the Forest Inventory of the State of Oaxaca, to biomass (Conafor- Semarnat, 2013).
In the 9 m2 sampling units, the aerial part of the bushes was cut in order to quantify their total green weight (g); a sample was subsequently selected and weighed in field (GV, g). The weeds, dead leaves and necromass were sampled in the 1 m2 site. The total weight of each component and the green weight (g) of the samples stored in paper bags were estimated in field. The data of these samples were recorded for identification purposes, and they were taken to the laboratory, where they were dried. The samples of bushes, necromass, dead leaves and herbs were dried in the same oven at ±70 °C until they reached a constant weight, and then they were weighed again to calculate the dry weight (g) of each component using the scale that was utilized in field.
The conversion factor (CF) had to be estimated, in order to quantify the biomass in the compartments of bushes, dead leaves, weeds and necromass, by dividing the dry weight by the green weight of the samples. The biomass of each of these compartments was estimated by multiplying the fresh weight of the vegetal material in the site by the CF.
Fuel load
The estimated fuel loads are consolidated in two strata: 1) living fuels (weeds and bushes) and 2) dead surface fuels (dead leaves and necromass). This variable was estimated in the sites by compartment, using the equations proposed by Morfín et al. (2012) (Table 1).
Table 1 Equations utilized to determine fuel loads by forest floor compartment (Morfín et al., 2012).

L = Load or biomass (t ha-1); DW = Dry weight (kg); N = Plot size (m2); dl = Depth of dead leaves (mm); d = Apparent density (t ha-1); k = Constant (1.234); SG = Specific gravity (kg m-3); QD = Quadratic diameter (cm); L = Transect length (m).
Statistical analysis
The database of the biomass of the wood and of the compartments (dead leaves, necromass, bushes and weeds) was formed. These data of the compartments by vegetation types were subjected to a variance analysis and to Duncan’s mean comparison test (P < 0.05). The SAS software package (SAS, 2005) was used for the analysis routine. Due to the high variability found in the biomass and in the fuel loads, the Log(x) had to be used in order to fulfill the variance normality and homogeneity assumptions and to detect the statistical differences.
Results
Surface and aerial biomass
The assessed vegetation types showed significant differences (p < 0.009) in the biomass of the weeds, which was considerably higher (Duncan, 0.05) in the pine forest (2.11 ± 0.67 t ha-1) than in the montane cloud forest (0.79 ± 0.41 t ha-1). The highest wood biomass contents were found in the oak forest (135.5 t ha-1), and the lowest (43.31 t ha-1), in the pine forest. Due to the high variability of the wood, it was not possible to detect significant differences using Duncan’s mean comparison test (Table 2).
Table 2 Biomass by surface and aerial compartment in each vegetation type.

Different letters in the same row indicate statistical differences (Duncan, 0.05). The mean is accompanied by ± standard deviation.
On the other hand, no significant statistical differences (Duncan, p > 0.05) were found between the three vegetation types in the biomass accumulated in the bushes, dead leaves or necromass. Of these, the dead leaves of the oak forest had the highest proportion (7.33 t ha-1) (Table 2).
The total surface biomass accumulation, excluding the wood of the trees, presented a statistically significant difference (p = 0.08) in each vegetation type. In the oak and pine forests, the figures were 15.248 and 11.192 t ha-1, respectively, while in the montane cloud forest the total mean value was 8.778 t ha-1 (Table 2 and Figure 2).
Forest fuel load, total and by compartment
The assessed vegetation types showed differences in the forest fuel load accumulated in the weeds (Duncan, p = 0.009), with higher values in the montane cloud forest. This compartment accumulated a larger amount of fuel in this vegetal association than oak and pine forests; the value for the weeds was 0.56 t ha-1, and 0.66 t ha-1 for the necromass. In contrast, the dead leaves and bushes compartments (p ≥ 0.08) did not confirm significant differences, with a fuel average of 1.26 and 0.13 t ha-1, respectively; the necromass registered the highest variability (Table 3).
Table 3 Surface fuel loads by compartment and vegetation type.
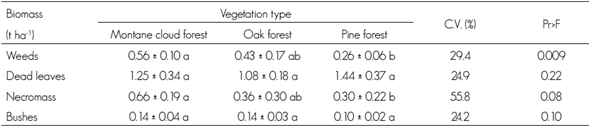
Different letters in the same row indicate statistical differences (Duncan, 0.05). The mean is accompanied by ± standard deviation. Pr>F= Significance.
When the biomass present in the various compartments (weeds, dead leaves, necromass and bushes) was added, the total biomass turned out to be 2.61 t ha-1 in the montane cloud forest, and 2.01 t ha-1 in the oak forest; these values are significantly different (Duncan, 0.05) (Figure 3). The topographic characteristics of the sites and, primarily, their steep slopes, ranging between 28 and 60 %, influence the scarce accumulation of fuel, which, probably during the rainy seasons, cause the dead leaves and the necromass to be dragged downhill, preventing excessive amounts of fuel to accumulate in the sites. The mountainside where the study area is located is oriented mainly toward the northeast, east, north, northwest and southwest, while the latitude ranges from 1 323 to 1 504 masl.
Discussion
In a pine-oak forest in Pueblo Nuevo, Durango, Rentería et al. (2005) determined that the highest concentrations of fuels are located in areas with a low slope (>20 %); this factor ranged between 28 and 60 % in the area of the present study; furthermore, the mean annual precipitations in this area, of 1 900 mm, must be taken into account in order to explain the presence of conditions that sweep away the dead leaves and necromass toward sites located downhill, whereby the excessive concentration of biomass on the forest floor of the studied sites is prevented.
Aguilar et al. (2011) determined the dry biomass accumulated as dead leaves in forests with a variation range of 1.70 to 20.10 t ha-1. The results obtained for this compartment in the present study show a variation range of 2.81 to 7.33 ha-1; the highest value corresponds to the oak forest. Rodríguez et al. (2011) estimated the fuel loads in the rain forest, in which the variation range for dead leaves was of 0.023 to 6.811 t ha-1. These values are similar to those reported by Rocha and Ramírez (2009), with a load of 6.58 ± 0.27 t ha-1 for oak forests in Chiapas; the present study yielded values within the expected interval based on the studies carried out by these authors.
According to Arnaldos et al. (2004), the supply of dead leaves depends on the production rate of each type of vegetal community, while Villers et al. (2012) mention that the accumulation of dead leaves is related rather to the percentage of adult trees in the site than to the orientation of the mountainside or the makeup of the vegetation. On the other hand, Aguilar et al. (2011) determined the accumulation of necromass at an interval of 0.15 a 22.64 t ha-1. In the present study, the obtained values showed a variation range of 1.85 to 2.71 t ha-1; these values are within the lower boundaries of the values obtained by the author for this compartment.
The total surface biomass ranged between 8.778 and 15.248 t ha-1; the latter value corresponds to the oak forest. According to the international norms of biomass loads mentioned by Porrero (2001), these figures are low and do not entail high risks of forest fires. The surface fuel load estimated by Bonilla et al. (2013) in a pine-oak forest for the compartments of dead leaves, weeds and shrubs was 1.73±6.17, 0.36±0.57 and 0.26±0.60 t ha-1, respectively. The values mentioned above by the author are similar for the compartments assessed in the present study (Table 2).
On the other hand, Castillo et al. (2003) point out that the largest loss of biomass is due to forest fires, and, we should add, to the effects of their combustion, such as the emission of gases (carbon dioxide, carbon monoxide, nitric oxide). According to Llovet (2006), the soil is also severely impacted by the phenomenon due to the increase in temperature and the deposition of ashes; furthermore, the vegetation and the organic horizons disappear partially or totally, modifying the incidence of rain on the soil surface.
Rodríguez (2014) determined the fuel loads in San Miguel Chimalapa; his results are similar to those obtained in the present study for the weeds and bushes compartment, except for the dead leaves, which have a higher load in the montane cloud and pine forests (Tables 3 and 4).
Rojas and Villers (2008) estimated 2.09 t ha-1 of total biomass for the oak forest; this value is similar to the one calculated in the present study (Figure 3). The international norms regard 20 to 30 t ha-1 as high risk, 15 a 20 t ha-1 as medium risk, and less than 15 t ha-1 as low risk (Porrero, 2001). Thus, the interval of 2.008 a 2.59 t ha-1 determined in this study -the highest found in the montane cloud forest- suggests that the risk of fire is low.
The climate in the study area is semiwarm, subhumid (A) C (w2) (w), with relatively high humidity; the mean annual temperature is 20 °C, and the mean annual precipitation ranges between 576 and 3 300 mm, which helps reduce the risk of fire, as, according to Muñoz et al. (2005), the weather conditions (temperature, relative humidity and precipitation) are the main factors that determine the hazard and the occurrence of forest fires. However, Torres et al. (2007) establish that the state of Oaxaca has a high risk of fires, which may be explained by both the conditions of the physical environment and the strong incidence of the human factor.
Conclusions
The total biomass of the weeds, bushes, dead leaves and necromass compartments among the vegetation types of the montane cloud, oak and pine forests was 8.77, 15.23 and 11.07 t ha-1, respectively. The first had the highest risk indicators (2.61 t ha-1) in relation to the total fuel load on the forest floor. The risk of forest fires in the study area is low for all three vegetation types.
Acknowledgements
The authors wish to express their gratitude especially to the communal authorities of San Antonio, Chimalapa, Oaxaca, and to the forest fire brigade assigned to this community, for the facilities granted.
REFERENCES
Aguilar A., H., E. Ortiz M., B. Vílchez A. y R. L. Chazdon. 2011. Biomasa sobre el suelo y carbono orgánico en el suelo en cuatro estadios de sucesión de bosque en la Península de Osa, Costa Rica. Revista Mesoamericana Kurú 9: 22-31. http://dx.doi.org/10.18845/rfmk.v9i22.360 (2 de marzo de 2015). [ Links ]
Anta F., S. y A. Plancarte B. 2001. Los incendios forestales en Chimalapas: La última oportunidad. World Wildlife Fund. Programa México. Secretaría del Medio Ambiente, Recursos Naturales y Pesca. Oaxaca, Oax., México. pp. 121-138. [ Links ]
Asbjornsen, H. y C. Gallardo. 2004. Impactos de los incendios de 1998 en el bosque mesófilo de montaña de Los Chimalapas, Oaxaca. In: Villers, L. y J. López (eds.). Incendios forestales. Métodos de evaluación. UNAM. México, D.F., México. pp. 125-145. https://books.google.com.mx/books?id=CiPQd6FSecC&pg=PA141&lpg=PA141&dq=Los+incendios+forestales+en+Chimalapas:+La+%C3%BAltima+oportunidad&source=bl&ots=CfkGyJYlDi&sig=79fS2znFOJESSIOI050pKpkIVhw&hl=es419&sa=X&ved=0ahUKEwjSxOya_bHMAhXB5KYKHdNuAjsQ6AEIGjAA#v=onepage&q=Los%20incendios%20forestales%20en%20Chimalapas%3A%20La%20%C3%BAltima%20oportunidad&f=false (1 de marzo de 2014). [ Links ]
Arnaldos V., J., X. Navalón N., E. Pastor F., E. Planas C. y L. Zárate L. 2004. Manual de ingeniería básica para la prevención y extinción de incendios forestales. Mundi Prensa. Madrid, España. 414 p. http://www.mundiprensa.com/catalogo/9788484761204/manual-de-ingenieria-basica-para-la-prevencion-y-extincion-de-incendios-forestales (23 de agosto de 2015). [ Links ]
Bonilla P., E., D. A. Rodríguez T., A. Borja de la R., C. Cíntora G. y J. Santillán P. 2013. Dinámica de combustibles en rodales de pino-encino de Chignahuapan, Puebla. Revista Mexicana de Ciencias Forestales 4 (19): 20-33. [ Links ]
Castañeda R., M. F., A. R. Endara A., M. L. Villers R. y E. G. Nava B. 2015. Evaluación forestal y de combustibles en bosques de Pinus hartwegii en el Estado de México según densidades de cobertura y vulnerabilidad a incendios. Madera y Bosques 21(2): 45-58. http://www.scielo.org.mx/scielo.php?script=sci_arttext&pid=S140504712015000200003 (10 de febrero de 2016). [ Links ]
Castillo, M., P. Pedernera y E. Peña. 2003. Incendios forestales y medios ambiente: una síntesis global. Revista Ambiente y Desarrollo de CIPMA 19(3): 44-53. http://linfor.forestaluchile.cl/wp-content/uploads/2014/03/ID-05-2003-Incendios-forestes-y-M.amb_.pdf (25 de agosto de 2015). [ Links ]
Chávez D., Á. A., J. Xelhuantzi C., E. A. Rubio C., J. Villanueva D., H. E. Flores L. y C. Mora O. 2016. Caracterización de cargas de combustibles para el manejo de reservorios de carbono y la contribución al cambio climático. Revista Mexicana de Ciencias Agrícolas 13: 2589- 2600. http://www.redalyc.org/articulo.oa?id=263144472012 (6 de marzo de 2016). [ Links ]
Comisión Nacional Forestal-Secretaría de Medio Ambiente y Recursos Naturales (Conafor-Semarnat) 2013. Inventario estatal forestal y de suelos-Oaxaca 2013-2014. Conafor-Semarnat. México, D.F., México. 166 p. [ Links ]
Contreras A., R. 2009. Estudio de inventario de combustible y generación de información base para el programa de manejo integrado de fuego en Los Chimalapas. Región Frontera Sur, Istmo y Pacífico Sur. Comisión Nacional de Áreas Naturales Protegidas. Oaxaca, Oax., México. 47 p. http://www.conanp.gob.mx/rendicion_cuentas/pdf/ESTUDIOS%202009/RAFAEL%20CONTRERAS%20AGUADO%20Inventario%20de%20Combustibles%20y%20base%20programa%20MIF%20Chimalapas.pdf (28 de agosto de 2015). [ Links ]
Grupo Mesófilo A. C. 2006. Diagnóstico para la elaboración de planes comunitarios de prevención de incendios forestales y manejo del fuego en Santa María y San Miguel Chimalapas, Oaxaca. Oaxaca, Oax., México. 49 p. http://www.camafu.org.mx/index.php/prevencion-y-combate-de-incendios-forestales-/articles/diagnostico-para-la-elaboracion-de-planes-comunitarios-de-preven.html (26 de abril de 2015). [ Links ]
Estrada C., I. y D. R. Ángeles C. 2007. Evaluación de combustibles forestales en el Parque Nacional “El Chico”, Hidalgo. Ecología y biodiversidad, claves de la prevención. Pachuca, Hgo., México. 17 p. http://www.fire.unifreiburg.de/sevilla2007/contributions/doc/SESIONES_TEMATICAS/ST3/Estrada_Angeles_SPAIN_Zaragoza.pdf (4 de marzo de 2014). [ Links ]
Llovet L., J. 2006. Degradación del suelo posterior al fuego en condiciones mediterráneas. Identificación de factores de riesgo. Revista Científica y Técnica de Ecología y Medio Ambiente 15(3): 199-202. http://www.revistaecosistemas.net/index.php/ecosistemas/article/view/503 (8 de marzo de 2014). [ Links ]
Morfín R, J. E., E. J. Jardel P., E. Alvarado C. y J. M. Michel F. 2012. Caracterización y cuantificación de combustibles forestales. Comisión Nacional Forestal-Universidad de Guadalajara. Guadalajara, Jal., México. 94 p. http://www.conafor.gob.mx:8080/biblioteca/ver.aspx?articulo=459 (8 de marzo de 2014). [ Links ]
Muñoz R., C. A., E. J. Treviño G., J. Verástegui C., J. Jiménez P. y O. A. Aguirre C. 2005. Desarrollo de un modelo espacial para la evaluación del peligro de incendios forestales en la Sierra Madre Oriental de México. Investigaciones Geográficas 56: 101-17. http://dx.doi.org/10.14350/rig.30099 (8 de marzo de 2014). [ Links ]
Porrero R., M. A. 2001. Incendios forestales. Investigación de causas. Tomo I. Mundi Prensa. Madrid, España. 158 p. http://www.casadellibro.com/libro-incendios-forestales-1investigacion-de-las-causas/978847149541/763679 (23 de agosto de 2015). [ Links ]
Rentería A., J. B., E. J. Treviño G., J. de J. Návar Ch., O. A. Aguirre C. e I. Cantú S. 2005. Caracterización de combustibles leñosos en el Ejido Pueblo Nuevo, Durango. Revista Chapingo Serie Ciencias Forestales y del Ambiente 1(1): 51-56. http://biblat.unam.mx/pt/revista/revista-chapingo-serie-ciencias-forestales-y-delambiente/articulo/caracterizacion-de-combustibles-lenosos-en-el-ejido-pueblo-nuevo-durango (15 de marzo de 2015). [ Links ]
Rocha L., A. G. y N. Ramírez M. 2009. Producción y descomposición de hojarasca en diferentes condiciones sucesionales del bosque de pino-encino en Chiapas, México. Boletín de la Sociedad Botánica de México (84): 1-12. http://www.redalyc.org/articulo.oa?id=57712091001 (26 de septiembre de 2015). [ Links ]
Rojas C., M. C. y A. Ríos V. 2012. Proyecto: Sistemas productivos sostenibles y biodiversidad. Comisión Nacional para el Conocimiento y Uso de la Biodiversidad. México. 260 p. http://www.conabio.gob.mx/web/pdf/SPSB_InformeEvaluacionAmbiental.pdf (24 de febrero de 2016). [ Links ]
Rojas G., F. y L. Villers R. 2008. Estimación de la biomasa forestal del parque nacional Malinche: Tlaxcala-Puebla. Revista Ciencia Forestal en México 33(104): 59-85. [ Links ]
Rodríguez T., D. A., H. Tchikoué, C. Cíntora G., R. Contreras A. y A. de la Rosa V. 2011. Modelaje del peligro de incendio forestal en las zonas afectadas por el huracán Dean. Agrociencia 45: 593-608. [ Links ]
Rodríguez T., D. A. 2012. Génesis de los incendios forestales. Revista Chapingo Serie de Ciencias Forestales y del Ambiente 18(3): 358-373. http://www.scielo.org.mx/scielo.php?script=sci_arttext&pid =S01863231201200030008 (4 de marzo de 2014). [ Links ]
Rodríguez C., E. A. 2014. Programa del manejo integrado del fuego de San Miguel Chimalapa. Comunidad de Aprendizaje y Manejo del Fuego (CAMAFU). Oaxaca, Oax., México. 80 p. [ Links ]
Rubio C., E. A., M. A. González T., J. D. Benavides S., Á. A. Chávez D. y J. Xelhuantzi C. 2016. Relación entre necromasa, composición de especies leñosas y posibles implicaciones del cambio climático en bosques templados. Revista Mexicana de Ciencias Agrícolas 13: 2601-2614. [ Links ]
Statistical Analysis System (SAS). 2005. The SAS for Windows. V. 9.01. SAS Institute. Cary, NC, USA. 480 p. [ Links ]
Torres R., J. M., O. S. Magaña T. y G. A. Ramírez F. 2007. Índice de peligro de incendios forestales a largo plazo. Agrociencia 41(6): 663-674. http://www.redalyc.org/articulo.oa?id=30241608 (28 de febrero de 2014). [ Links ]
Villers G., S., L. Villers R. y J. López B. 2012. Modelos que relacionan las características biofísicas del terreno con la presencia de combustibles forestales en las montañas centrales de México. Boletín de la Asociación de Geógrafos Españoles 59: 369- 388. http://www.scielo.org.mx/scielo.php?script=sci_arttext&pid=S14050471201500020003 (8 de marzo de 2014). [ Links ]
Villers, L. , M. Castañeda y A. Endara. 2013. Alerta temprana a incendios forestales en México. In: 4o Congreso Forestal Argentino Latinoamericano Iguazú. http://www.scielo.org.mx/scielo.php?script=sci_nlinks&ref=3234389&pid=S1405-0471201500020000300070&lng=es (14 de marzo de 2014). [ Links ]
Xelhuantzi C., J., J. G. Flores G. y A. A. Chávez D. 2011. Análisis comparativo de cargas de combustibles en ecosistemas forestales afectados por incendios. Revista Mexicana de Ciencias Forestales 2(3): 37-52. [ Links ]
Zinck, D., R., M. Pascual and V. Grimm. 2011. Understanding shifts in wildfire regimes as emergent threshold phenomena. The American Naturalist 178(6): 150-161. http://www.journals.uchicago.edu/doi/full/10.1086/662675 (6 de marzo de 2014). [ Links ]
b Contribution by author: Jorge Hernández García: field sampling; Gerardo Rodríguez Ortiz: statistical data analysis, revision and editing of the manuscript; José Raymundo Enríquez del Valle: laboratory analyses; Gisela Virginia Campos Ángeles: laboratory analyses; Adán Hernández López: identification of vegetal species.
Received: April 27, 2016; Accepted: June 19, 2016