Serviços Personalizados
Journal
Artigo
Indicadores
-
Citado por SciELO
-
Acessos
Links relacionados
-
Similares em SciELO
Compartilhar
Revista mexicana de ciencias pecuarias
versão On-line ISSN 2448-6698versão impressa ISSN 2007-1124
Rev. mex. de cienc. pecuarias vol.14 no.3 Mérida Jul./Set. 2023 Epub 08-Set-2023
https://doi.org/10.22319/rmcp.v14i3.6255
Reviews
Bm86 protein and its potential use as an anti-tick vaccine in cattle. Review
a Universidad Autónoma del Estado de México. Centro de Investigación y Estudios Avanzados en Salud Animal, Facultad de Medicina Veterinaria y Zootecnia. Autopista Toluca-Atlacomulco Km 15.5, San Cayetano de Morelos, Toluca, México.
b Centro de Investigación y de Estudios Avanzados del Instituto Politécnico Nacional (CINVESTAV), México.
c Universidad Tecnológica del Valle de Toluca, México.
d Biomedical Research Unit. Zacatecas, México.
Tick infestations represent a major difficulty for cattle production in tropical and subtropical countries around the world. The traditional ways to combat this pest are chemicals (acaricides) and integrated management of grasslands. Nevertheless, these methods have not been able to conclusively control the presence of this ectoparasite. This has led to the search for vaccines that allow the control of these parasites. Currently, there are immunogens containing the gut antigen Bm86 of Rhipicephalus microplus, which have proven to be an effective alternative, as they have reduced tick populations and the use of synthetic acaricides. However, the polymorphism of this locus in ticks from different geographical areas represents a limitation in its effectiveness. A significant number of studies have been conducted on the Bm86 gene as a vaccine antigen, so this paper presents an update on studies conducted with this antigen and its potential use as an immunological control to reduce tick infestations in cattle.
Keywords Cattle; Bm86; Ticks; Rhipicephalus microplus; Vaccines
Las infestaciones de garrapatas representan una gran dificultad para la producción de ganado bovino en países tropicales y subtropicales alrededor del mundo. Las formas tradicionales de combate a esta plaga son químicos (acaricidas) y manejo integral de pastizales. Sin embargo, estos métodos no han logrado controlar de manera contundente la presencia de este ectoparásito. Lo anterior ha llevado a la búsqueda de vacunas que permitan el control de estos parásitos. Actualmente, se cuenta con inmunógenos que contienen el antígeno intestinal Bm86 de Rhipicephalus microplus, los cuales han demostrado ser una alternativa efectiva, al reducir las poblaciones de garrapatas y el uso de acaricidas sintéticos. No obstante, el polimorfismo de este locus en garrapatas de distintas zonas geográficas representa una limitante en su efectividad. Se han realizado un número importante de investigaciones acerca del gen Bm86 como antígeno vacunal, por lo que, en este documento, se presenta una actualización de las investigaciones realizadas con este antígeno y su potencial uso como control inmunológico para reducir las infestaciones de garrapatas en el ganado bovino.
Palabras clave Bovino; Bm86; Garrapatas; Rhipicephalus microplus; Vacunas
Introduction
Immunological control of ticks in cattle, compared to chemical control, has the advantage of being a relatively inexpensive strategy with no environmental impact. It helps prevent the development of resistance to acaricides, since when these are used in combination with vaccines based on the recombinant protein Bm86 (rBm86), the periodicity of their application can be reduced. Additionally, it has the ability to control various species of ticks and contributes to the prevention of the transmission of the causative agents of diseases such as bovine babesiosis and anaplasmosis1. The first proposed anti-tick vaccine was developed from the Bm86 antigen present in epithelial cells of the gut of R. microplus2,3, whose biological function is associated with endocytosis mechanisms1,2,4. The success of this vaccine lies in the fact that it induces a reduction in the number of organisms that complete their life cycle after feeding on vaccinated animals, and even reduces the fertility of the surviving tick population5. The Bm86 antigen has been field-tested with acceptable success and is commercially available under four brand names: Gavac® from Cuba, TickGuard-Plus from Australia, Go-Tick from Colombia and Bovimune Ixovac® from Mexico6,7. The general recommendation is that they be used in combination with ixodicides, which can significantly reduce the need for the latter. Nevertheless, vaccines may have a variable efficacy that depends on the polymorphism of the Bm86 gene in open populations of ticks, which may also have a negative effect on the strategy of combined use of vaccine-ixodicides8. This demonstrates the need to continue research on the Bm86 gene to develop vaccines with a higher degree of efficacy. Therefore, this paper aims to present a current overview of the characteristics of the Bm86 antigen and its use as a vaccine in cattle.
Bm86 protein
The Bm86 protein is a glycoprotein identified and isolated from epithelial cells of the gut of ticks (Rhipicephalus microplus), Yeerongpilly strain9. It contains 650 amino acids and a predicted molecular weight of approximately 80 kDa2,4; three sites of function are recognized: 1) the N-terminal signal peptide (1-19aa), which is the chain that appears first when the polypeptide chain is synthesized and contains the information on the secretion efficiency, pathway and destination of the protein, 2) the Bm86 antigen or glycoprotein (20-627aa), which is the polypeptide chain of the mature protein after processing, 3) a propeptide (628-650aa), which is the part of the protein that is cleaved during maturation or activation. It is known that the mature protein contains a hydrophobic transmembrane region that encompasses 23aa, located in the region of the C-terminal end, the rest of the protein exhibits high hydrophilic potential. The main characteristic of the protein sequence is the repeated pattern of 6 cysteine residues, which suggests the presence of various domains of the EGF (Epithelial Growth Factor) type (Figures 1 and 2)2,10-12.

The following are shown: signal peptide (blue arrow); Epithelial Growth Factor-type domains (EGF- brown arrows); the glycosylphosphatidylinositol anchor (GPI-green arrow); Cysteines for the formation of disulfide bridges (grey boxes-arrowhead); N-glycosylation sites (isolated arrowheads).
Figure 1 Primary structure of the Bm86 protein
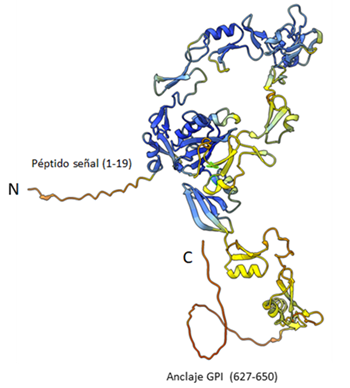
The Bm86 protein is formed by a polypeptide chain of 650 amino acids that contains a signal peptide (1-19), several domains of the Epithelial Growth Factor (EGF) type (20-600) and is associated with the membrane by a GPI anchor at the C-Terminal. The figure shows the 3D model generated by Alpha-Fold, obtained from the Uniprot database (https://www.uniprot.org/uniprotkb/P20736/entry#structure) and visualized with ChimeraX (https://www.cgl.ucsf.edu/chimerax/).
Figure 2 3D model of the Bm86 protein
Bm86 polymorphism
Discontinuous genetic variations in the Bm86 gene have been reported, present in different populations of R. microplus ticks13,14. Two or more of these variants common in a natural population are called polymorphisms (from Greek, many forms)15. The sequence of the Bm86 gene of three reference strains has been compared (Yeerongpilly, Australia and Camcord from Cuba: these have identical amino acid sequences, except for residue 111, which is isoleucine in the Camcord strain and threonine in the Australian strain)16,17. Likewise, in several studies it has been observed that, in some genetic regions, the vast majority of strains present a series of mutations (residues 89, 109, 142, 206, 210, 226, 248, 257 and 272) that are conserved in the strains of continental lands of the Americas but that differ from the reference strains (Table 1)16,17.
Table 1 Comparative analysis of conserved amino acid sequences in the Bm86 gene from strains from Texas, South America and the Bm95 gene (derived from an Argentine strain) that differ from the Yeerongpilly strain
Position of the amino acid residue in the Bm86 antigen in the Yeerongpilly strain |
Bm86 (Texas) (a) |
Bm86 (South America) (b) |
Bm95 (Argentina) (c) |
---|---|---|---|
17 | + | Na | - |
89 | + | + | + |
95 | - | - | + |
109 | + | + | - |
142 | + | + | + |
206 | + | + | + |
210 | + | + | + |
226 | + | + | + |
248 | - | + | - |
251 | + | - | + |
257 | + | + | + |
272 | + | + | + |
334 | - | Na | + |
358 | + | Na | + |
365 | - | Na | + |
400 | + | Na | + |
444 | + | Na | + |
498 | + | Na | + |
502 | + | Na | + |
507 | + | Na | + |
560 | + | Na | + |
568 | + | Na | + |
570 | - | Na | Stop codon |
593 | - | Na | |
597 | + | Na | |
605 | + | Na | |
610 | + | Na | |
624 | + | Na | |
650 | Stop codon | ¿Stop codon? |
Taken from Martínez-Arzate:21. Only the positions of the amino acids where there are mutations of the analyzed strains in relation to the originally cloned Bm86 antigen (Yeerongpilly) are shown. +, there is a point mutation that modifies the amino acid, -, it does not present the mutation in that site, Na= not analyzed by the authors of the publication. a) (16), b) (17), c) Genbank with accession number AF150891.2. The sequences were analyzed with the Mega 4 program22.
In Argentina, the Bm86 gene was cloned and named Bm95 (genbank with accession number AF150891.2), it has almost the same variants as the South American and Texan genes mentioned above18. On the other hand, variations of 3.1-7.4 % have been found among Mexican strains in the nucleotides of the Bm86 protein, finding 145 point polymorphisms along the gene19. Similarly, in a study conducted in Thailand, they sequenced Bm86 from 29 tick samples, obtaining similarities between 91.5-97.0 % between Thai strains and the reference sequence (Yeerongpilly)20. According to the author, the phylogenetic analysis revealed geographical patterns among the Bm86 sequences from Thailand, which were classified into 5 or 9 groups depending on the sequences of nucleotides or amino acids. This contrasts with what has been reported in Mexico, where haplotypes are not limited to one region, which could be explained by the occurrence of gene flow between different regions, perhaps due to the mobility of infested animals that redistribute genotypes19.
The construction of a cladogram with some sequences of the Bm86 gene obtained from reports available in GenBank allows to observe that there is a large genetic polymorphism in specimens from different regions of the world (Figure 3). The cladogram was made using the Neighbor-joining algorithm, its arrangement shows the evolutionary direction and the relationship of sequences of the Bm86 gene. Three major clades, A, B and C, can be distinguished, each of which presents a polyphyletic arrangement. Likewise, a common ancestor seems to give rise to the outgroup formed by the only sequence of R. appendiculatus and the different branches that form clade A, it shows the terminal dichotomies: China-Yeerongpilly (Australian vaccine strain TickGARD®) and Mozambique-XM, in addition to a pair of unique terminal sequences, corresponding to sequences from Texas. One of the ramifications of the common ancestor for all sequences also gives rise to the putative ancestor for clades B, C and for the only loose terminal corresponding to one of the Mexican isolates, and which fits in a different evolutionary direction from clades B and C. In clade B, unique terminals are observed for 3 of the Mexican sequences and a dichotomy for 2 of the Texan sequences. Clade C shows the dichotomies Mexico-Zapata and Texas-Texas, as well as unique terminals such as Zapata 5, Zapata 1 and Argentina. Although some branches are supported by relatively low bootstrap values, the polyphyletic arrangement of the cladogram helps explain the polymorphism of the Bm86 gene which could have a direct impact on the efficacy of the commercial vaccine in Mexico, in addition, reports for the Bm86 gene from Mexico and Texas show relatively close relationships, which could be due to the commercial relationship of cattle and the natural migration of wild animals between the two countries16.
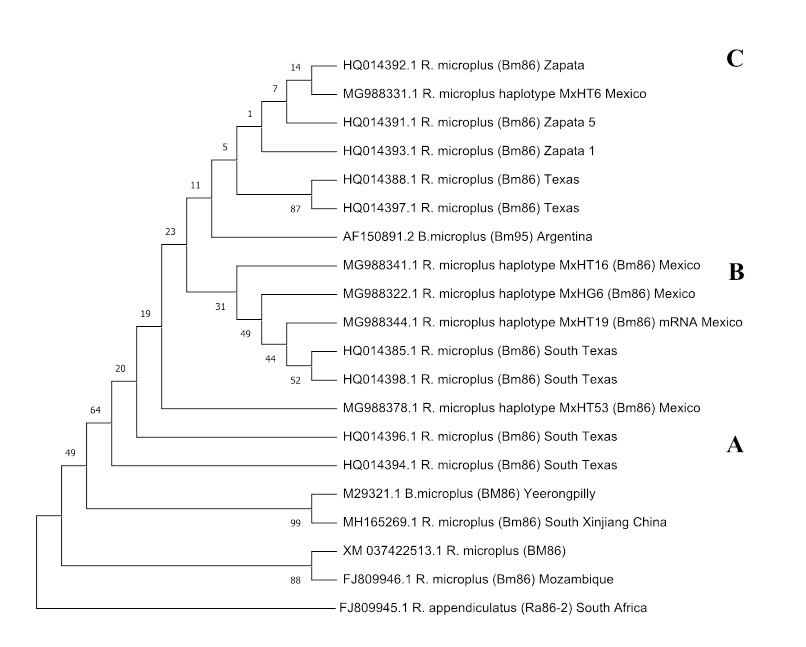
The cladogram shows the phylogenetic relationship between different sequences of the Bm86 gene. The strain of Rhipicephalus appendiculatus homologous to Bm86 (Ra86-2) was used as an outgroup. The numbers on the nodes indicate bootstrap values. The boot support percentage of 10,000 pseudo-replicates is shown next to the branches, which are based on evolutionary distances calculated with Jukes-Cantor. Evolutionary analyses were performed in MEGAX22.
Figure 3 Neighbor-joining cladogram of the Bm86 gene of Rhipicephalus microplus
Commercial vaccines
Anti-tick vaccines began to be developed around the 1980s, when animals were immunized with proteins from crude extracts of semi-engorged females23,24. It was observed that the proteins affect the midgut of the tick and cause permanent damage to both epithelial cells and basement membrane. This has negative effects on the metabolism, digestive system and reproductive performance of ticks23,24. In this context, the protein of interest was isolated from the midgut and identified as a membrane glycoprotein, which was purified, characterized and named Bm869.
Based on the above, the first vaccines against ticks in cattle were registered between 1993 and 1997 in Latin America (Gavac®) and Australia (TickGARD®)4,6,25. The TickGARD® vaccine was developed by the GCSIRO (Commonwealth Scientific and Industrial Research Organization) industry in collaboration with Biotech Australia Pty. Ldt., coming to market in 1994 by Hoechst Animal Health. To prepare it, they isolated the Bm86 protein gene from the gut of the Rhipicephalus microplus tick and cloned it in Escherichia coli26. In 1995, they expressed Bm86 in Pichia pastoris and it was released on the market as TickGARD®PLUS27. The reason was that Bm86 demonstrated improved expression in P. pastoris due to glucan residues added during protein maturation in yeast (reviewed by Willadsen in a report of the industry)28.
In the first four years after its launch, it became the best-selling treatment in that country. However, near the end of the decade, its sale ceased due to the separation and closure of the companies in charge of its production. Years later it was reintroduced to the market by Intervet Australia Pty. Ltd., but after a short period its public sale was ceased6.
The results of the application of the TickGARD® vaccine (Table 2) were first reported in 1995, obtaining a significant reduction in the use of acaricides and a 72 % decrease in the reproductive capacity of ticks29. Another study reported 56 % reductions in the number of ticks in the field over one generation and a 72 % reduction in the reproductive capacity of R. microplus females under laboratory conditions30. On the other hand, the Cuban group developed the Gavac® vaccine at the Center for Genetic Engineering and Biotechnology in Havana Cuba by Heber Biotec SA., which was registered in Cuba in 1993, in Colombia and the Dominican Republic in 1994 and in Brazil, Paraguay and Bolivia in 19956,31,32. They used the rBm86 protein expressed in the methylotrophic yeast Pichia pastoris, because it has been shown to be a suitable host for the industrial production of heterologous proteins33. It was estimated that the amount of rBm86 secreted by P. pastoris is approximately 1.5-2.0 g/L, higher than that obtained in fermentations of E. coli34,35. Its vaccination schedule consists of three primary applications of 2 ml each (100 μg rBm86), intramuscularly (IM), with intervals of three weeks in each application, with a booster every 6 mo36. The effectiveness of the vaccine has been determined using several parameters such as: the number of adult females; the effect on the weight of semi-engorged ticks; the survival of adult female ticks; the number of eggs and their fertility32.
Table 2 Summary of the efficacies of vaccines containing the Bm86 antigen against different tick species
Tick species (strain) against which the vaccine was tested |
Vaccine | Efficacy (%) | References |
---|---|---|---|
R. microplus (Yeerongpilly) | Gavac® | 75 | 45 |
R. microplus (Cenapa) | Gavac® | 84 | 45 |
R. microplus (Tuxpan) | Gavac® | 51 | 45 |
Boophilus annulatus | Gavac® and TickGARD® | > 99.9 | 46 |
R. microplus (Campo Grande) | TickGARDPLUS® | 46.4 | 39 |
R. microplus (Campo Grande | Gavac® | 49.2 | 39 |
R. microplus (Colombia) | Go-Tick | 80 | 47 |
R. microplus (Media Joya) | Bovimune Ixovac® | 86 | 42 |
(%), Efficacy expressed in the reduction of the number of adult female ticks per head of cattle, reducing of oviposition and fertility.
For example, in a controlled study, the effectiveness of the Gavac® vaccine against different strains of R. microplus was evaluated and in different breeds of cattle, it was found that the efficacy was >50 %32. The vaccine has also been shown to be effective in controlling artificial infestations of B. annulatus, B. decoloratus, and strains of B. microplus, whether sensitive or resistant to chemicals in Australia, Africa, the Americas, and Iran (Table 2)3. In addition, in a cost-effectiveness analysis, the vaccine demonstrated a 60 % reduction in the number of acaricidal treatments, as well as the control of tick infestations and babesiosis transmission, which resulted in savings of 23.4 dollars per animal/yr37. Similarly, in controlled field trials in Cuba, 588,573 dairy cows were vaccinated, which showed an 87 % reduction in the use of acaricides over 8 yr (1995-2003)38.
In addition, in a study, cattle were inoculated with TickGARDPLUS® and Gavac® and the animals were challenged with a Brazilian strain of R. microplus “Campo Grande”, the results showed an effectiveness of 46.4 and 49.2 %, respectively39. Considering the above, variations in the efficacy of vaccines can be observed, which can be explained by the genetic diversity (polymorphism) of tick populations and host factors8. Some authors suggest that the variation in the sequence of the Bm86 locus (> 3 % in the amino acid sequence) is one of the main reasons why commercial vaccines have limited efficacy in certain R. microplus populations13.
In 2018, a vaccine was developed in Mexico from the germplasm of the R. microplus strain “Media Joya”, it was cloned in the expression vector Pichia pastoris. The vaccine was registered under the name Bovimune Ixovac® (Lapisa S.A., Guadalajara, Mexico), with registration SAGARPA B-2083-03, and is being used in the state of Guerrero where there are populations of multidrug-resistant ticks40. Its vaccination schedule consists of three primary applications of 2 ml each (120 ± 20 μg of rBm86), IM, at weeks 0, 4 and 7, followed by biannual revaccination (at a single dose)41.
The treatment has made it possible to maintain infestations of less than 10 ticks per animal, and to reduce the application of tick baths from 14 to 3 per year, in addition to reducing fertility of female ticks by 86 %42. So far there is no more information published on the sequence and effectiveness of the vaccine in other regions of Mexico.
Similarly, in Colombia, the LIMOR laboratory developed the Go-Tick vaccine against R. microplus with registration No. 6774-BV of the Colombian Agricultural Institute, based on the Bm86 antigen extracted from tick larvae. The same vaccine is marketed under the brand name Tick-Vac by the TECNOQUÍMICAS laboratory. Its vaccination schedule consists of three initial vaccinations of 2 ml each, subcutaneously or IM, on days 0, 20 and 60, followed by biannual revaccination43. In a field trial in Colombia, the vaccine showed a combined effect of 80 % protection7. Whereas in clinical field studies, it has demonstrated protection from 64 to 96 %, reducing parasite load in production systems located in different national agroecosystems in Colombia44.
Humoral immune response against Bm86 antigen
Anti-tick vaccines stimulate the humoral immune response of cattle through the production of protective antibodies, which allows a sustained immune response for a period of time after the application of the vaccine, even when the natural Bm86 antigen of the ectoparasite never comes into contact with the host48,49. When cattle are inoculated with an initial dose of vaccine containing the Bm86 antigen, antigen-presenting cells (APCs), such as dendritic cells and macrophages50, capture, process and present the antigen in the lymph node via bovine leukocyte antigen (BoLA)51. Activated dendritic cells interact with naive T cells that differentiate into effector helper T cells. These cells migrate to the lymphoid germinal centers, where they interact with naive B cells, which leads to the formation of plasma and memory B cells. Plasma cells migrate to the bone marrow52 and induce the production of antigen-specific antibodies, while memory B cells are found in the spleen and lymph nodes53,54, where they await subsequent inoculation (booster dose) of the antigen (Figure 4).
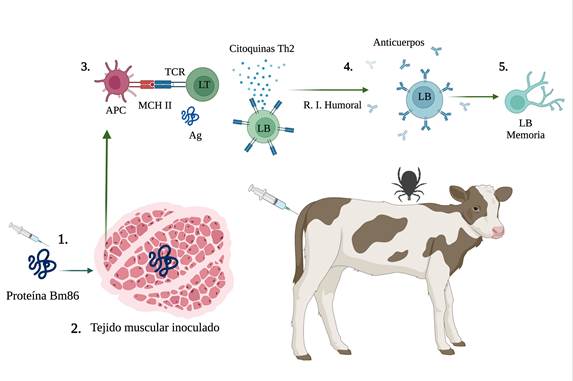
1) The vaccine contains the recombinant protein Bm86. 2) It is inoculated intramuscularly (IM). 3) The T-cell receptor (TCR) recognizes Bm86 epitopes bound to Major Histocompatibility Complex (MHC II) class II molecules and expresses itself on the surface of antigen-presenting cells (APCs). 4) Helper CD4+ T lymphocytes (TL) recognize the MHC II + peptide complex and, activated by cytokines, they begin the humoral immune response. 5) The antigen binds to a B lymphocyte (BL) receptor, stimulating proliferation and differentiation into plasma cells, antibody-producing cells and memory cells51. Created with BioRender.com
Figure 4 Mechanism of action of immunity induced by the Bm86 antigen in vaccinated animals
Anti-Bm86 antibodies present in the blood of immunized cattle reach the gut of the tick when they feed on vaccinated animals. The antibodies (IgM and IgG) bind to the Bm86 antigen and activate the complement system (classical pathway), culminating in the lysis of enterocytes, disrupting their function of digesting and absorbing nutrients from the blood, thus affecting the gut and general health of the tick55,56.
Immunofluorescence and immunohistochemistry studies have shown that antibodies recognize the plasma membrane of the enterocyte; site associated with the Bm86 protein57. In other experiments, feeding ticks with serum from cattle inoculated with Bm86 demonstrated inhibition in endocytosis, an effect that precedes detectable enterocyte damage58.
Related to the above, in a study they used the plasmid pBMC2 encoding the Bm86 antigen of the strain of the Colombian tick R. microplus to perform immunization tests in mice and cows. Mice immunized with pBMC2 showed high levels of anti-Bm86 antibodies. When evaluating immunogens, significant levels of IgG2a and IgG2b were observed in mice immunized with 50 mg of pBMC2. They also assessed the persistence of the antibody response to vaccination as serum antibody levels must remain high for the vaccine to be effective. Antibody responses were present 56 d after the first immunization in mice and 70 days in cattle. In addition, cattle immunized with 2 mg (1 ml) of pBMC2 had a mean tick count below 50, while other groups (immunized with PBS or 1 mg of pBMC2) exceeded this level59. Similarly, the recombinant form of the Bm86 antigen of the Campo Grande strain (rBm86-CG) of R. microplus has been used to stimulate humoral immunity in cattle. Immunoblot results showed that vaccinated cattle developed significant levels of antibodies against rBm86-CG (P<0.05), which peaked 21 d after the third vaccination60.
The Bm86 protein as a hidden antigen
Hidden antigens are components of remarkable physiological importance for ectoparasites, which do not usually participate in host-parasite interaction and in their native form have no contact with the host during natural infestation, so, naturally, no immune response of any kind develops against them. However, they have been shown to be able to trigger a protective immune response when the host is exposed to them artificially, by inoculating them into a vaccine61. The Bm86 antigen is found naturally in the gut wall of the R. microplus tick; this protein has a transmembrane sequence that keeps it bound to the epithelial cells of the tick gut, so that, in its natural form, it never has contact with the immune system of the tick host55. Nevertheless, when this protein is used in its recombinant form as a vaccine antigen, it causes a humoral immune response in the animal; Bm86-specific immunoglobulins bind to the antigen presented on tick enterocytes, causing lysis of the tick’s gut wall, which interferes with blood digestion and nutrient absorption, which in turn reduces egg production (Figure 5). The use of these antigens in vaccine design has the hypothetical advantage of avoiding parasitic evasion mechanisms, that is, thanks to the lack of contact between the hidden antigens and the immune system, the tick cannot develop a strategy to escape the animal’s immune action61. Nonetheless, since a large polymorphism of this gene has been demonstrated, this supposed advantage of Bm86 as a hidden antigen is compensated, therefore it is necessary to consider this polymorphism in the development of vaccines based on the recombinant protein Bm86. Additionally, one of the disadvantages of Bm86 as a hidden antigen is that it induces a permanent response. Therefore, the protective immunity induced by Bm86 derivatives in vaccines is short-lived2,62 and requires booster doses at 6-mo intervals63.
The anti-Bm86 antibodies present in the blood of the immunized cattle penetrate through the mouth of the tick until they reach the gut. The antibodies bind to the Bm86 antigen and activate the complement system (classical pathway)51. Complement activation begins when two or more Fc (fragment crystallizable) fragments of the antibody react with the C1 component (subcomponents C1q, C1r and C1s)64. Cell damage is mediated by the membrane attack complex (MAC), which consists of a polymer of components C5b, C6, C7, C8 and a polymer of C9. MACs cause lytic damage to cells, facilitating the separation of lipids from the membrane and creating pores through which water, ions and macromolecules can enter and exit64,65.
In-silico analysis of Bm86
The identification of B-cell epitopes in target antigens is a crucial step for the development of vaccines based on these, immunodiagnostic tests, antibody production and disease therapy64. Vaccines against R. microplus containing the Bm86 antigen are based on synthetic subunits or peptides, whose mechanism focuses on epitopes that are recognized by B and T cells and bovine leukocyte antigen (BoLA) with which a supposed specific immune response is predicted65. Since experimental methods for mapping B-cell epitopes are time-consuming, expensive and laborious; in-silico (computer design) methods for predicting both linear and conformational B-cell epitopes64 are a versatile tool that allows researchers to easily manage variables in a DNA sequence, such as: select promoter sequences; terminator sequences; genes; untranslated regions; signal peptides; insert or remove restriction sites that facilitate manipulation in the laboratory66. This tool allows evaluating which regions of the proteins of interest could be candidates for the creation of new vaccines or treatments67. This was the case of three peptides (SBm4912, SBm7462 and SBm19733) generated by in-silico trials of the Bm86 glycoprotein from the gut of R. microplus, with which they immunized cattle and evaluated the immune response by detecting anti-Bm86 antibodies. The results revealed an efficacy greater than 80 % in cattle immunized with the synthetic peptide SBm7462. Likewise, they observed a decrease in the number, weight and oviposition capacity of engorged females in the tick population that had fed on immunized animals68. In one study, they used the synthetic peptide BD86 (a Bm86 ortholog of Rhipicephalus decoloratus) to immunize mice. The results showed that the antibodies generated were able to recognize Bm86 orthologs in four species: R. microplus, R. decoloratus, Hyalomma anatolicum anatolicum and R. appendiculatus, and bound to the ticks’ gut69.
Globally, three antigenic determinants of protein sequences homologous to Bm86 were identified. Phylogenetic analysis of isolated epitopes and the Bm86-CG protein (GenBank: ACA57829) revealed geographic patterns between R. microplus tick isolates and those collected from the database. The authors propose the possibility of achieving effective vaccination against ticks in cattle using a single universal antigen based on Bm86. Therefore, as genomic technologies continue to be developed, it will be possible to identify new candidate vaccines against different tick species worldwide70. On the other hand, there are publications that propose a reduction in the efficacy of the vaccine based on the Bm86 protein due to the natural polymorphism of the gene in open tick populations, which suggests that strategies should be used that cover a greater variety of antigens derived from the polymorphism of target populations of ticks71.
Bm86 alleles and orthologous genes
The high degree of polymorphism of Bm86 has led to the identification of multiple alleles of the protein. Recent studies have discovered new proteins that share structural similarities with Bm86, and that could be good candidate vaccines against cattle ticks. For example, an allele of the Bm86 gene identified in Argentina and that has been used as a vaccine is Bm95. This allele was cloned and expressed in P. pastoris yeasts, and the recombinant protein was shown to induce protection in cattle against strains resistant and sensitive to the GAVAC® vaccine72. Likewise, controlled immunization trials have shown that vaccines containing Rhipicephalus microplus Bm86 also protect against related tick species, such as; Rhipicephalus annulatus and Rhipicephalus decoloratus73,74.
In this sense, some authors suggest that the efficacy (Table 3) of a vaccine based on Bm86 may be improved when the orthologous recombinant Bm86 antigen (genes that share the last common ancestor and whose divergence is due to speciation) is used75. This is the case of the Bm86 orthologs of R. annulatus (Ba86) and R. decoloratus (Bd86) that were used to immunize rabbits. The results showed cross-immune reactivity of epitopes of orthologous Bm86 proteins48. These trials provide a starting point for the development of improved vaccine formulations, incorporating several tick-protective antigens11.
Table 3 Comparison of efficacy of Bm86 alleles and its orthologs
Tick species (strain) against which alleles and orthologous genes of Bm86 were tested |
Bm86 alleles and orthologous genes |
Efficacy (%) | Reference |
---|---|---|---|
Rhiphicephalus haemaphysaloides | Bm95 | Larvae: 98.7 Nymphs: 84.6 Adults: 78.9 | 75 |
Rhipicephalus microplus | Bm95 | 81.27 | 77 |
B. annulatus (strain Mercedes, Texas, EE. UU.) | Ba86 | 83.0 | 78 |
B. microplus (susceptible strain, Mexico) | Ba86 | 71.5 | 78 |
Rhipicephalus appendiculatus | Ra86 | Egg hatching: 50 | 76 |
(%), Efficacy expressed in the reduction of the number of adult female ticks per head of cattle, reduction of oviposition and fertility.
Likewise, the Bm86 ortholog of R. appendiculatus (Ra86) has been used to vaccinate cattle. The results showed a significant decrease in the molting success of nymphs, which suggests that repeated vaccinations with Ra86 could reduce tick populations in successive generations and could be part of comprehensive tick control strategies76.
Conclusions
Immunological control is one of the most promising methods for controlling tick infestations in cattle. Nevertheless, as mentioned, vaccines based on the Bm86 protein sometimes have an effectiveness differential (46.4 - 99.9 %) when the vaccine protein sequence is obtained from ticks in a certain region and used to fight ticks in a different geographical region. This aspect is attributed to the differences in the sequences that exist in the Bm86 protein throughout the world (polymorphism). Therefore, multiple efforts have been made in the search for new proteins, antigens based on other alleles of the Bm86 gene or orthologous genes that can stimulate the immune response in cattle and allow the development of more effective recombinant vaccines.
Such is the case of Colombia and Mexico, which have designed vaccines from national or local strains of ticks, which have shown promising results in the country of origin. Similarly, in Argentina, administration of the Bm95 allele as a vaccine antigen has been shown to confer greater than 80 % protection79 against tick strains susceptible or resistant to ixodicides. Greater antigen effectiveness has been observed when it comes from local tick alleles, which suggests that the use of native antigens is important to improve the effectiveness of vaccines against tick infestation. Finally, trials of multiantigen vaccines to date have been few and limited to controlled trials due to difficulties in antigen formulation. Therefore, it is important that future studies delve into these issues to effectively combat tick infestations.
REFERENCES
1. Bastos RG, Ueti MW, Knowles DP, Scoles GA. The Rhipicephalus (Boophilus) microplus Bm86 gene plays a critical role in the fitness of ticks fed on cattle during acute Babesia bovis infection. Parasites Vectors 2010;3(1). [ Links ]
2. Willadsen P, Jongejan F. Immunology of the tick-host interaction and the control of ticks and tick-borne diseases. Parasitol Today 1999;15(7):258-262. [ Links ]
3. de La Fuente J, Rodríguez M, Montero C, Redondo M, García-García JC, Méndez L, et al Vaccination against ticks (Boophilus spp.): the experience with the Bm86-based vaccine Gavac. Genetic Analysis 1999;15(3-5):143-148. [ Links ]
4. Rand KN, Moore T, Sriskantha A, Spring K, Tellamt R, Willadsent P, Cobon GS. Cloning and expression of a protective antigen from the cattle tick Boophilus microplus. Proc Natl Aca Sci 1989;86(24):9657-9661. [ Links ]
5. Jonsson N, Matschoss AL, Pepper P, Green PE, Albrecht MS, Hungerford J, Ansell J. Evaluation of TickGARD (PLUS), a novel vaccine against Boophilus microplus, in lactating Holstein-Friesian cows. Vet Parasitol 2000;1(88):275-285. [ Links ]
6. de la Fuente J, Almazán C, Canales M, Pérez de la Lastra JM, Kocan KM, Willadsen P. A ten-year review of commercial vaccine performance for control of tick infestations on cattle. Anim Health Res Rev 2007;8(1):23-28. [ Links ]
7. Betancourt JA. Control integrado de la garrapata Rhipicephalus microplus (garrapata común del ganado) con base en la vacuna Go Tick en fincas lecheras de diferentes regiones de Colombia. Acovez 2019;48. [ Links ]
8. Parizi LF, Pohl PC, Masuda A, Vaz Ida S Jr. New approaches toward anti-Rhipicephalus (Boophilus) microplus tick vaccine. Rev Bras Parasitol Vet 2009;18(1):1-7. [ Links ]
9. Willadsen P, Riding GA, McKenna RV, Kemp DH, Tellam R L, Nielsen JN, et al. Immunologic control of a parasitic arthropod. Identification of a protective antigen from Boophilus microplus. J Immunol 1989;143(4):1346-1351. [ Links ]
10. Azhahlanambi P, de la Fuente J, Suryanarayana VVS, Ghosh S. Cloning, expression and immunoprotective efficacy of rHaa86, the homologue of the Bm86 tick vaccine antigen, from Hyalomma anatolicum anatolicum. Parasite Immunol 2009;31(3):111-122. [ Links ]
11. Nijhof AM, Balk JA, Postigo M, Rhebergen AM, Taoufik A, Jongejan F. Bm86 homologues and novel ATAQ proteins with multiple epidermal growth factor (EGF)-like domains from hard and soft ticks. Int J Parasitol 2010;40(14):1587-1597. [ Links ]
12. Riding GA, Jarmey J, McKenna RV, Pearson R, Cobon GS, Willadsen P. A protective “concealed” antigen from Boophilus microplus. Purification, localization, and possible function. J Immunol 1994;153(11):5158-5166. [ Links ]
13. Garcı́a-Garcı́a JC, Montero C, Redondo M, Vargas M, Canales M, Boue O, et al. Control of ticks resistant to immunization with Bm86 in cattle vaccinated with the recombinant antigen Bm95 isolated from the cattle tick, Boophilus microplus. Vaccine 2000;18(21):2275-2287. [ Links ]
14. Fuente J de la, Garcı́a-Garcı́a JC, González DM, Izquierdo G, Ochagavia ME. Molecular analysis of Boophilus spp. (Acari: Ixodidae) tick strains. Vet Parasitol 2000;92(3):209-222. [ Links ]
15. Eberhard Passarge . Genética texto y atlas. Ed. Médica Panamericana, editor. 2009. [ Links ]
16. Freeman JM, Davey RB, Kappmeyer LS, Kammlah DM, Olafson PU. Bm86 midgut protein sequence variation in South Texas cattle fever ticks. Parasit Vectors 2010;3(1):101. [ Links ]
17. Sossai S, Peconick AP, Sales-Junior PA, Marcelino FC, Vargas MI, Neves ES, et al. Polymorphism of the bm86 Gene in South American strains of the cattle tick Boophilus microplus. Exp Appl Acarol 2005;37(3-4):199-214. [ Links ]
18. de la Fuente J, Rodríguez M, García-García JC. Immunological control of ticks through vaccination with Boophilus microplus gut antigens. Ann NY Acad Sci 2000;916(1):617-621. [ Links ]
19. Martínez-Arzate SG, Sánchez-Bermúdez JC, Sotelo-Gómez S, Diaz-Albiter HM, Hegazy-Hassan W, Tenorio-Borroto E, et al. Genetic diversity of Bm86 sequences in Rhipicephalus (Boophilus) microplus ticks from Mexico: Analysis of haplotype distribution patterns. BMC Genet 2019;20(1):56. [ Links ]
20. Kaewmongkol S, Kaewmongkol G, Inthong N, Lakkitjaroen N, Sirinarumitr T, Berry CM, et al. Variation among Bm86 sequences in Rhipicephalus (Boophilus) microplus ticks collected from cattle across Thailand. Exp Appl Acarol 2015;66(2):247-256. [ Links ]
21. Martínez-Arzate SG. Análisis filogenético molecular de la secuencia de la proteína Bm86 de la garrapata Rhipicephalus (Boophilus) microplus de Colima, México. [Toluca]: Universidad Autónoma del Estado de México; 2014. [ Links ]
22. Kumar S, Stecher G, Li M, Knyaz C, Tamura K. MEGA X: Molecular evolutionary genetics analysis across computing platforms. Mol Biol Evol 2018;1;35(6):1547-1549. [ Links ]
23. Kemp DH, Agbada RIS, Johnston LAY, Gough JM. Immunization of cattle against Boophilus microplus using extracts derived from adult female ticks: Feeding and survival of the parasite on vaccinated cattle. Int J Parasitol 1986;16(2):115-120. [ Links ]
24. Willadsen P, McKenna RV, Riding GA. Isolation from the cattle tick, Boophilus microplus, of antigenic material capable of eliciting a protective immunological response in the bovine host. Int J Parasitol 1988;18(2):183-189. [ Links ]
25. Willadsen P, Bird P, Cobon GS, Hungerford J. Commercialization of a recombinant vaccine against Boophilus microplus. Parasitology 1995;6;110(S1):43-50. [ Links ]
26. Estrada-Peña A, Venzal JM, Nava S, Mangold A, Guglielmone AA, Labruna MB, et al. Reinstatement of Rhipicephalus (Boophilus) australis (Acari: Ixodidae) with redescription of the adult and larval stages. J Med Entomol 2012;49(4):794-802. [ Links ]
27. Tabor AE. A review of Australian tick vaccine research. Vaccines 2021;9(9):1030. [ Links ]
28. Willadsen P. The development of a new or improved vaccine against Boophilus Microplus: Opportunities for R&D investment; Meat & Livestock Australia. Sydney, Australia; 2008. [ Links ]
29. Cobon G HJ, Woodrow M, Smith D, Willadsen P. Vaccination against Boophilus microplus: the Australian field experience. Elfos Scientiae 1995;163-176. [ Links ]
30. Jonsson NN, Matschoss AL, Pepper P, Green PE, Albrecht MS, Hungerford J, Ansell J. Evaluation of tickGARD (PLUS), a novel vaccine against Boophilus microplus, in lactating Holstein-Friesian cows. Vet Parasitol 2000;88(3-4):275-285. [ Links ]
31. Massard CL, da Fonseca AH, Pinheiro VRE, de Olveira. Evaluation of recombinant rBm86- “GAVAC” vaccine against the tick Boophilus microplus in Brazil. R Bras Med Vet 1995;17:167-173. [ Links ]
32. de la Fuente J, Rodríguez M, Fragoso H. Efficacy of vaccination with Gavac in the control of Boophilus microplus infestations. Elfos Scientiae 1995;177-185. [ Links ]
33. Paifer E, Margolles E, Cremata J, Montesino R, Herrera L, Delgado JM. Efficient expression and secretion of recombinant alpha amylase in Pichia pastoris using two different signal sequences. Yeast 1994;10(11):1415-1419. [ Links ]
34. Canales M, Enriquez A, Ramos E, Cabrera D, Dandie H, Soto A, Falcón V, Rodríguez M, de la Fuente J. Large-scale production in Pichia pastoris of the recombinant vaccine Gavac against cattle tick. Vaccine 1997;15(4):414-422. [ Links ]
35. Rodríguez M, Rubiera R, Penichet M, Montesinos R, Cremata J, Falcón V, et al. High-level expression of the B. microplus Bm86 antigen in the yeast Pichia pastoris forming highly immunogenic particles for cattle. J Biotechnol 1994;33(2):135-146. [ Links ]
36. Jaramillo Hernández DA. Importancia de la vacunación dentro del manejo integrado de Rhipicephalus microplus en bovinos. Rev Sistemas Prod Agroecol 2022;13(1):48-63. [ Links ]
37. de la Fuente J, Rodríguez M, Redondo M, Montero C, García-García JC, Méndez L, et al. Field studies and cost-effectiveness analysis of vaccination with Gavac against the cattle tick Boophilus microplus. Vaccine 1998;16(4):366-373. [ Links ]
38. Valle MR, Méndez L, Valdez M, Redondo M, Espinosa CM, Vargas M, et al. Integrated control of Boophilus microplus ticks in Cuba based on vaccination with the anti-tick vaccine Gavac TM. Exp Appl Acarol 2004;34(3-4):375-382. [ Links ]
39. Andreotti R. Performance of two Bm86 antigen vaccine formulation against tick using crossbreed bovines in stall test. Rev Bras Parasitol Vet 2006;15:97-100. [ Links ]
40. Lagunes Quintanilla RE, Bautista-Garfias CR. El control inmunológico: Una alternativa contra garrapatas del ganado bovino. Ecosist Recursos Agropecu 2019;7(1). [ Links ]
41. LAPISA. Bovimune ixovac la vacuna contra garrapatas. Bovimune Ixovac. [cited 2023 Jan 14]. https://lapisa.com/productos/bovimune-ixovac . [ Links ]
42. Guerra Liera JE, Ruíz Lang CG, Saltijeral Oaxaca JA. Repensando la ganadería. 1a Ed. México, D.F. Universidad Autónoma Metropolitana Xochimilco; 2016. [ Links ]
43. Enríquez A, Boué O, Redondo M, Montero C, Machado H, Joglar M, et al. Desarrollo y aplicación en bovinos de la nueva formulación vacunal GavacPlus contra la garrapata Boophilus microlplus. Biotecnología Aplicada 1999;16:15-17. [ Links ]
44. Betancourt A. Las vacunas contra la garrapata común del ganado: componente fundamental para control integrado del parásito. Acovez 2017;46:15-20. [ Links ]
45. Penichet M, Rodriguez M, Castellano O, Mandado S, Rojas Y, Rubiera R, Sanchez P, Lleonart R, De La Fuente J. Detection of Bm86 antigen in different strains of Boophilus microplus and effectiveness of immunization with recombinant Bm86. Parasite Immunol 1994;16(9):493-500. [ Links ]
46. Fragoso H, Rad PH, Ortiz M, Rodríguez M, Redondo M, Herrera L, et al. Protection against Boophilus annulatus infestations in cattle vaccinated with the B. microplus Bm86-containing vaccine Gavac. Vaccine 1998;16(20):1990-1992. [ Links ]
47. Betancourt A, Patiño F, Torres O, Eugenio B. Prueba de establo para evaluar la efectividad de la vacuna Tick Vac®, contra la garrapata Boophilus microplus. Acovez 2005;34(3):18-25. [ Links ]
48. Zinkernagel RM. On natural and artificial vaccinations. Annu Rev Immunol 2003;21(1):515-546. [ Links ]
49. Sallusto F, Lanzavecchia A, Araki K, Ahmed R. From vaccines to memory and back. Immunity 2010;33(4):451-463. [ Links ]
50. Reichardt P, Dornbach B, Gunzer M. APC, T cells, and the immune synapse. Curr Top Microbiol Immunol 2010;340:229-249. [ Links ]
51. Ndawula C. From bench to field: A guide to formulating and evaluating anti-tick vaccines delving beyond efficacy to effectiveness. Vaccines (Basel) 2021;9(10):1185. [ Links ]
52. Manz RA, Thiel A, Radbruch A. Lifetime of plasma cells in the bone marrow. Nature 1997;388:133-134. [ Links ]
53. Moran I, Nguyen A, Khoo WH, Butt D, Bourne K, Young C, et al. Memory B cells are reactivated in subcapsular proliferative foci of lymph nodes. Nat Commun 2018; 9(1):3372. [ Links ]
54. Phan TG, Tangye SG. Memory B cells: total recall. Curr Opin Immunol 2017;45:132-140. [ Links ]
55. Rodríguez JG, Olivares JL. Vacunas parasitarias: un recuento bibliográfico. Rev Salud Anim 2019;41(3). [ Links ]
56. Hamilton SE, Kemp DH, McKenna RV, Willadsen P. Gut cells of the tick Boophilus microplus: the effects of vaccination on digest cells and experiments on blood meal absorption by these cells. In: Academia/SPB Academic Publishing, editor. Modern Acarology 1991:341-351. [ Links ]
57. Penichet M, Rodríguez M, Castellano O, Mandado S, Rojas Y, Rubiera R, et al. Detection of Bm86 antigen in different strains of Boophilus microplus and effectiveness of immunization with recombinant Bm86. Parasite Immunol 1994;16(9):493-500. [ Links ]
58. Willadsen P, Kemp DH. Vaccination with 'concealed' antigens for tick control. Parasitol Today 1988;4(7):196-198. [ Links ]
59. Ruiz LM, Orduz S, López ED, Guzmán F, Patarroyo ME, Armengol G. Immune response in mice and cattle after immunization with a Boophilus microplus DNA vaccine containing bm86 gene. Vet Parasitol 2007;144(1-2):138-145. [ Links ]
60. Cunha RC, Pérez de León AA, Leite FP, Pinto Lda S, Dos Santos Júnior AG, Andreotti R. Bovine immunoprotection against Rhipicephalus (Boophilus) microplus with recombinant Bm86-Campo Grande antigen. Rev Bras Parasitol Vet 2012;21(3):254-262. [ Links ]
61. Valle Rodríguez, Manuel. Respuesta inmunológica contra garrapatas. Biotecnología Aplicada. Elfos Scientiae 2000;17:215-220. [ Links ]
62. Frisch JE. Towards a permanent solution for controlling cattle ticks. Int J Parasitol 1999;29(1):57-71. [ Links ]
63. Vargas M, Montero C, Sánchez D, Pérez D, Valdés M, Alfonso A, et al. Two initial vaccinations with the Bm86-based Gavac plus vaccine against Rhipicephalus (Boophilus) microplus induce similar reproductive suppression to three initial vaccinations under production conditions. BMC Vet Res 2010;6(1):43. [ Links ]
64. Sun P, Guo S, Sun J, Tan L, Lu C, Ma Z. Advances in In-silico B-cell Epitope prediction. Curr Top Med Chem 2019;19(2):105-115. [ Links ]
65. Ben-Yedidia T, Arnon R. Design of peptide and polypeptide vaccines. Curr Opin Biotechnol 1997;8(4):442-448. [ Links ]
66. Li X, Li S, Lang Z, Zhang J, Zhu L, Huang D. Chloroplast-targeted expression of the codon-optimized truncated cry1Ah gene in transgenic tobacco confers a high level of protection against insects. Plant Cell Rep 2013;32(8):1299-1308. [ Links ]
67. Tarca AL, Carey VJ, Chen X wen, Romero R, Drăghici S. Machine learning and its applications to biology. PLoS Comput Biol 2007;3(6):116. [ Links ]
68. Patarroyo JH, Portela RW, de Castro RO, Couto Pimentel J, Guzman F, Patarroyo ME, et al. Immunization of cattle with synthetic peptides derived from the Boophilus microplus gut protein (Bm86). Vet Immunol Immunopathol 2002;88(3-4):163-172. [ Links ]
69. Kopp N, Diaz D, Amacker M, Odongo DO, Beier K, Nitsch C, et al. Identification of a synthetic peptide inducing cross-reactive antibodies binding to Rhipicephalus (Boophilus) decoloratus, Rhipicephalus (Boophilus) microplus, Hyalomma anatolicum anatolicum and Rhipicephalus appendiculatus BM86 homologues. Vaccine 2009;28(1):261-269. [ Links ]
70. Blecha IMZ, Csordas BG, Aguirre AAR, Cunha RC, Garcia MV, Andreotti R. Analysis of Bm86 conserved epitopes: is a global vaccine against cattle tick Rhipicephalus microplus possible? Rev Bras Parasitol Vet 2018;27(3):267-279. [ Links ]
71. Nava S. An overview of systematics and evolution of ticks. Frontiers in Bioscience 2009;(14):2857. [ Links ]
72. Garcı́a-Garcı́a JC, Montero C, Redondo M, Vargas M, Canales M, Boue O, et al. Control of ticks resistant to immunization with Bm86 in cattle vaccinated with the recombinant antigen Bm95 isolated from the cattle tick, Boophilus microplus. Vaccine 2000;18(21):2275-2287. [ Links ]
73. de la Fuente J, Kocan KM. Strategies for development of vaccines for control of ixodid tick species. Parasite Immunol 2006;28(7):275-283. [ Links ]
74. de Vos S, Zeinstra L, Taoufik A, Willadsen P, Jongejan F. Evidence for the utility of the Bm86 antigen from Boophilus microplus in vaccination against other tick species. Exp Appl Acarol 2001;25(3):245-261. [ Links ]
75. Sugumar P, Chandran D, Sudha Rani G, Shahana PV, Maske DK, Rangarajan PN, et al. Recombinant mid gut antigen (Bm95) as a vaccine against Indian Rhiphicephalus haemaphysaloides in Bos indicus cattle. Res Vet Sci 2011;90(2):262-268. [ Links ]
76. Olds C, Mwaura S, Crowder D, Odongo D, van Oers M, Owen J, et al. Immunization of cattle with Ra86 impedes Rhipicephalus appendiculatus nymphal-to-adult molting. Ticks Tick Borne Dis 2012;3(3):170-178. [ Links ]
77. Kumar A, Garg R, Yadav CL, Vatsya S, Kumar RR, Sugumar P, et al. Immune responses against recombinant tick antigen, Bm95, for the control of Rhipicephalus (Boophilus) microplus ticks in cattle. Vet Parasitol 2009;165(1-2):119-124. [ Links ]
78. Canales M, Almazán C, Naranjo V, Jongejan F, de la Fuente J. Vaccination with recombinant Boophilus annulatus Bm86 ortholog protein, Ba86, protects cattle against B. annulatus and B. microplus infestations. BMC Biotechnol 2009;31;9(1):29. [ Links ]
79. Kumar A, Garg R, Yadav CL, Vatsya S, Kumar RR, Sugumar P, et al. Immune responses against recombinant tick antigen, Bm95, for the control of Rhipicephalus (Boophilus) microplus ticks in cattle Vet Parasitol 2009;165(1-2):119-124. [ Links ]
Received: June 07, 2022; Accepted: March 16, 2023