Servicios Personalizados
Revista
Articulo
Indicadores
-
Citado por SciELO
-
Accesos
Links relacionados
-
Similares en SciELO
Compartir
Revista mexicana de ciencias agrícolas
versión impresa ISSN 2007-0934
Rev. Mex. Cienc. Agríc vol.14 no.1 Texcoco ene./feb. 2023 Epub 19-Jun-2023
https://doi.org/10.29312/remexca.v14i1.3385
Articles
Silicon as a salinity mitigator in the physiological variables of germination of three varieties of Solanum lycopersicum
1Maestría en Innovación y Producción Orgánica en Ambientes Áridos y Costeros-Universidad Autónoma de Baja California Sur. Carretera al Sur km 5.5, La Paz, Baja California Sur, México. AP. 19-B. CP. 23080.
2Universidad Autónoma de Nuevo León-Facultad de Agronomía. Av. Francisco Villa s/n, Ex Hacienda el Canadá, General Escobedo, Nuevo León, México. CP. 66450.
3División de Estudios de Posgrado de la Facultad de Agricultura y Zootecnia-Universidad Juárez del Estado de Durango. Ejido Venecia, Gómez Palacio, Durango, México. (cirvaz60@hotmail.com , gshd40@hotmail.com).
At present, agricultural soils are affected by salinity due to the excessive use of synthetic fertilizers and poor quality of the water for agricultural irrigation. Currently, products based on silicon (Si) are being applied to help the sustainability of agriculture, in addition, Si benefits plants to have greater tolerance to pests and diseases, helps against heavy metal toxicity and acts against water and salt stress. Therefore, the aim of the study was to evaluate the effect of silicon as a salinity mitigator on seed germination and initial growth of tomato seedlings. The work was carried out in the Germplasm laboratory of the Autonomous University of Baja California Sur, Mexico. The selected tomato varieties were cherry (Solanum lycopersicum var. Cerasiforme), beef (Solanum lycopersicum var. Floradade) and saladette (Solanum lycopersicum var. Río Grande). The experimental design was completely randomized with a 2 x 3 factorial arrangement, where factor A was saline concentrations (0, 25, 50 mM) of sodium chloride (NaCl) and factor B was silicon dilutions (0, 1, 2 mM). Each treatment included four repetitions of 25 seeds each. Tomato cultivars showed different effects regarding salinity. The cherry cultivar showed decreases in its germinative variables with increasing salinity, indicating that the Floradade and Río Grande cultivars are more tolerant to salinity. In this same sense, silicon presented a protective effect in the interaction (NaCl + Si), showing positive effects by increasing the variables evaluated.
Keywords: Solanum lycopersicum L.; silicon dioxide; sodium chloride.
En la actualidad, los suelos agrícolas están afectados por salinidad debido al uso excesivo de fertilizantes y la mala calidad del agua para el riego agrícola. En la actualidad, los productos a base de silicio (Si) se están aplicando para ayudar a la sostenibilidad de la agricultura; además, el Si beneficia a las plantas a tener mayor tolerancia a plagas y enfermedades, ayuda contra la toxicidad por metales pesados y actúa contra el estrés hídrico y salino. Por lo tanto, el objetivo del estudio fue evaluar el efecto del silicio como atenuante de la salinidad en la germinación de semillas y crecimiento inicial de plántulas de tomate. El trabajo se realizó en el laboratorio de Germoplasma de la Universidad Autónoma Baja California Sur. Las variedades de tomate seleccionadas fueron: cherry (Solanum lycopersicum var. Cerasiforme), bola (Solanum lycopersicum var. Floradade) y saladette (Solanum lycopersicum var. Río Grande). El diseño experimental fue completamente al azar con arreglo factorial de 2 x 3, donde el factor A fueron las concentraciones salinas de cloruro de sodio (NaCl) (0, 25, 50 mM) y el factor B, las diluciones de silicio (0, 1, 2 mM). Cada tratamiento incluyó cuatro repeticiones de 25 semillas cada una. Los cultivares de tomate mostraron efectos diferentes respecto a la salinidad. El cultivar cherry mostró disminuciones en sus variables germinativas al incrementarse la salinidad, lo que indica que los cultivares Floradade y Río Grande son más tolerantes a salinidad. En este sentido el silicio presentó un efecto protector en la interacción (NaCl + Si), mostrando efectos positivos al incrementar las variables evaluadas.
Palabras claves: Solanum lycopersicum L.; cloruro de sodio; dióxido de silicio.
Introduction
Tomato (Solanum lycopersicum L.) is the most preferred vegetable worldwide (Infoagro, 2017). It has high contents of bioactive compounds such as: folate, ascorbate, polyphenols, carotenoids, vitamins, and other essential nutrients, which is why it is palatable for human health (Ahmed et al., 2017). FIRA (2019) mentions that, worldwide, the tomato is grown on approximately 5 million hectares (ha), with a production of 170.08 million tonnes (t) of fresh tomato, where China ranks first in the world with a tomato production of 46.2% of the total, while Mexico ranks tenth with a production of 1 878 289 t year-1.
Salinity affects 1 125 million ha worldwide (Hossain, 2019). This condition generates problems that agriculture faces worldwide, particularly due to the presence of NaCl, which limits the optimal development of plants of commercial interest (Durukan and Demirbas 2018). Mexico has an area of 54% of arid zones throughout the national territory, located mainly in the northern and northeastern regions of the country (Briones et al., 2018), which are affected with a high degree of salinity, where sodium (Na+) cations displace calcium (Ca+) of the clay-humic complex, thus increasing the osmotic potential of the soil solution (Cisneros et al., 2020).
Currently, various alternatives are being sought to mitigate the negative effects of salinity, such as obtaining tolerant varieties, natural products such as biofertilizers and biostimulants that favor the growth and development of plants and benefit the environment (Mazón et al., 2020).
Si is a mineral element that protects the plant against biotic and abiotic stress, in addition, chemically active Si restores damage and optimizes soil fertility, keeps nutrients available to the plant and improves cation exchange capacity, especially at pH greater than 7 (Meunier et al., 2018). Therefore, the objective was to evaluate the effect of Si as a salinity mitigator on germination and initial growth in seedlings of three tomato varieties.
Materials and methods
The research was conducted in the germplasm laboratory of the Autonomous University of Baja California Sur (UABCS, for its acronym in Spanish). The biological material used was three tomato cultivars: cherry (Solanum lycopersicum var. Cerasiforme), beef (Solanum lycopersicum var. Floradade) and saladette (Solanum lycopersicum var. Río Grande), the beef and saladette varieties are commercial varieties, while cherry is a landrace reproduced at the Autonomous University of Baja California Sur. Germination tests were carried out in sterilized Petri dishes (150 x 15 mm), covered at the bottom with a layer of absorbent paper. Each dish was moistened with 5 ml of the solution resulting from the combination of sodium chloride (NaCl) (0, 25 and 50 mM) and amorphous silicon dioxide (SiO2) (0, 1 and 2 mM), the application of the solution was made every four days, distilled water was used for the control treatment. The germination tests were performed for 14 days, in a germination chamber (Seedburo, Model 549, NY, USA) at a temperature of 25 °C ±0.5 °C and 80% relative humidity, which were monitored daily if applicable.
Variables evaluated
Germination percentage. it was recorded daily using the following formula of Al-mudaris (1998). Where:
Seeds were considered germinated when the radicle had a minimum length of 2 mm. The germination rate was calculated using the formula of Maguire (1962), where n1, n2, n30 are the number of seeds germinated at times t1, t2, t7 (in days). While the germination energy was calculated using the following formula of Maguire (1962):
Where: N= indicates the number of seeds germinated on the counting date; D= the number of days. In turn, the mean germination time was carried out by the formula proposed by Orchard (1977)
Where: N= indicates the number of seeds germinated on day D.
For its part, the germination index was calculated by the formula proposed by Scott et al. (1962),
Where: n= indicates the number of seeds germinated on day 1; t1= number of days after sowing; N= is the total number of seeds sown. While the germination speed was determined by the formula of Maguire (1962),
Where: n= indicates the number of seeds germinated on day 1, t= is the germination time from sowing to germination of the last seed.
Germinated seeds were kept for 14 days and 10 seedlings per repetition were randomly selected. Each seedling was measured stem and radicle length with a digital caliper (AutoTec, Rhos, 2002 EC). Subsequently, the fresh and dry biomass was weighed, using an analytical balance (Ohaus, model PA224C). The plant tissues were placed in paper bags and placed in a drying oven (Ríos Rocha model EO-50) at a temperature of 70 °C for 72 h until complete dehydration.
Experimental design
The experimental design was completely randomized with a 32 factorial arrangement, where factor A was the saline concentrations of NaCl (0, 25 and 50 mM) and factor B was the dilutions of Si (0, 1 and 2 mM). Each treatment had four repetitions of 25 seeds each.
Statistical analysis
The data obtained were analyzed for variance. A test of orthogonal polynomials was performed in the variables where there were significant differences. Differences between the mean treatments were compared with Tukey’s multiple range test (p≤ 0.05) of confidence level. Statistical analyses were performed with the Statistical Package for the Social Sciences (SPSS) version 22.0 (IBM Corp, 2013).
Results and discussion
The statistical analysis showed that the effect of NaCl, Si and the interaction between these factors was statistically different (p≤ 0.05) in each tomato variety (Table 1).
Table 1 Values of F (F) and significance levels (p) observed in the analyses of variance for germination and growth variables.
Variables | Cherry | Floradade | Río grande | ||||||||
NaCl | Si | NaCl*Si | NaCl | Si | NaCl*Si | NaCl | Si | NaCl*Si | |||
%GP | |||||||||||
F | 3.737 | 0.712 | 1.017 | 1.004 | 0.049 | 1.25 | 2.497 | 0.113 | 1.061 | ||
p | 0.037 | 0.5 | 0.416 | 0.38 | 0.952 | 0.314 | 0.101 | 0.893 | 0.395 | ||
GE | |||||||||||
F | 12.339 | 2.932 | 1.624 | 0.454 | 0.125 | 1.309 | 1.5 | 0.001 | 0.891 | ||
p | <0.001 | 0.07 | 0.197 | 0.64 | 0.883 | 0.292 | 0.241 | 0.999 | 0.483 | ||
GRI | |||||||||||
F | 11.124 | 9.312 | 6.387 | 5.562 | 8.677 | 2.558 | 7.926 | 2.138 | 1.637 | ||
p | <0.001 | <0.001 | <0.001 | 0.009 | <0.001 | 0.061 | 0.002 | 0.137 | 0.194 | ||
MGT | |||||||||||
F | 3.154 | 0.673 | 1.051 | 0.785 | 0.06 | 0.936 | 3.052 | 0.031 | 1.054 | ||
p | 0.059 | 0.519 | 0.399 | 0.466 | 0.942 | 0.458 | 0.064 | 0.969 | 0.398 | ||
GI | |||||||||||
F | 7.903 | 1.144 | 0.917 | 1.004 | 0.049 | 1.25 | 2.497 | 0.113 | 1.061 | ||
p | 0.002 | 0.333 | 0.468 | 0.38 | 0.952 | 0.314 | 0.101 | 0.893 | 0.395 | ||
GS | |||||||||||
F | 3.737 | 0.712 | 1.017 | 1.004 | 0.049 | 1.25 | 2.497 | 0.113 | 1.061 | ||
p | 0.037 | 0.5 | 0.416 | 0.38 | 0.952 | 0.314 | 0.101 | 0.893 | 0.395 | ||
RL | |||||||||||
F | 8.301 | 2.098 | 6.109 | 4.621 | 5.434 | 1.571 | 13.076 | 10.335 | 0.667 | ||
p | 0.002 | 0.142 | <0.001 | 0.019 | 0.01 | 0.211 | <0.001 | <0.001 | 0.62 | ||
SL | |||||||||||
F | 222.456 | 119.31 | 38.123 | 8.786 | 12.858 | 5.975 | 33.489 | 10.317 | 2.152 | ||
p | <0.001 | <0.001 | <0.001 | <0.001 | <0.001 | <0.001 | <0.001 | <0.001 | 0.102 | ||
FB | |||||||||||
F | 15.374 | 1.508 | 0.786 | 18.816 | 4.517 | 3.268 | 78.338 | 12.499 | 4.93 | ||
p | <0.001 | 0.239 | 0.544 | <0.001 | 0.02 | 0.026 | <0.001 | <0.001 | 0.004 | ||
DB | |||||||||||
F | 16.487 | 4.737 | 7.643 | 6.09 | 0.89 | 0.578 | 4.454 | 3.794 | 0.588 | ||
p | <0.001 | 0.017 | <0.001 | 0.007 | 0.422 | 0.681 | 0.021 | 0.035 | 0.674 |
Germination percentage (%GP); germination energy (GE); germination rate (GRI); mean germination time (MGT); germination index (GI); germination speed (GS); root length (RL); stem length (SL); fresh biomass (FB) and dry biomass (DB).
For the cherry variety, the concentration of 50 mM NaCl increased germination energy and germination index. Meanwhile, fresh biomass increased at concentrations of 25 and 50 mM NaCl. However, germination percentage, germination energy, germination index and germination speed were affected at the concentration of 25 mM NaCl, with respect to the control treatment (Table 2).
Table 2 Main effects of NaCl and Si concentrations on the physiological variables of germination of cherry tomato (Solanum lycopersicum var. Cerasiforme).
Dose | Variables evaluated | |||||
%GP | GE | MGT | GI | GS | FB | |
NaCl | ||||||
0 mM | 89.667 a | 11.716 ab | 0.219 | 0.152 a | 0.897 a | 1.41 b |
25 mM | 82.667 b | 10.723 b | 0.207 | 0.14 b | 0.827 b | 1.47 ab |
50 mM | 89.667 a | 13.002 a | 0.219 | 0.161 a | 0.897 a | 1.67 a |
±SE | 2.091 | 0.325 | 0.004 | 0.004 | 0.021 | 0.001 |
Linear | ns | 0.009 | ns | ns | ns | < 0.001 |
Quadratic | 0.011 | < 0.001 | ns | 0.001 | 0.009 | 0.012 |
Si | ||||||
0 mM | 86.667 | 12.348 | 0.215 | 0.154 | 0.867 | 1.6 |
1 mM | 89.333 | 11.855 | 0.218 | 0.152 | 0.893 | 1.48 |
2 mM | 86 | 11.237 | 0.212 | 0.147 | 0.86 | 1.47 |
±SE | 2.091 | 0.325 | 0.004 | 0.004 | 0.021 | 0.001 |
Linear | ns | ns | ns | ns | ns | ns |
Quadratic | ns | ns | ns | ns | ns | ns |
Different literals between the means indicate a significant difference. Germination percentage (%GP); germination energy (GE); mean germination time (MGT); germination index (GI); germination speed (GS); fresh biomass (FB); NS= not significant; ±SE= standard error.
Cuartero et al. (2006) reported that the genus lycopersicum has a heritable salinity tolerance trait. Vítámvá et al. (2007) mentioned that there is a group of proteins inducible by abscisic acid (ABA), which are associated with salinity tolerance and water deficit, which could be explained to what happened in the present work.
Several studies show that increases in the percentage, energy and index of germination have been found at concentrations of 50 mM NaCl in crops such as: spinach Spinacia oleracea (Turhan et al., 2011), tomato Solanum lycopersicum (Doğan et al., 2008) and eggplant Solanum melongena (Akıncı et al., 2004).
On the other hand, Eitel (2021) evaluated the effect of salt stress on the production and quality of tomato seeds, in different concentrations, where it was observed that the germination index decreased significantly. For their part, Ruiz et al. (2014) evaluated the germination of eight genotypes of tomato (Solanum lycopersicum L.), which were exposed to different levels of NaCl. Where they observed that the fresh and dry biomass of the aerial part increased in the presence of NaCl. Similarly, Can et al. (2017) evaluated the germination and initial growth of beans (Phaseolus vulgaris) in response to salinity induced with NaCl, sodium sulfate (Na2SO4) and sodium bicarbonate (NaHCO3), where they observed that fresh biomass was decreased with the salt type NaHCO3, while NaCl increased it.
Although the impact of Si was not indicated as the main factor, there was a significant effect in the interaction with NaCl. The presence of Si in the germination rate indicates a quadratic effect in the presence of NaCl (Figure 1A). Similar results were found by Haghighi et al. (2012), who evaluated the interaction factor of Si + NaCl in the germination of tomato seeds, where they observed that Si stimulated germination variables, especially the germination rate, which increased around 20% with respect to the control treatment. Likewise, Sun et al. (2021) observed the effect of Si on corn (Zea mays) germination, which significantly increased germination percentage (GP), germination rate (GR), germination index (GI) and vigor index (VI) compared to the control treatment.
The presence of Si in saline conditions (50 mM) generated an increase in root length (Figure 1B). Similar results were reported by Asgari et al. (2018), who found a greater increase in stem length, fresh and dry biomass of shoots and roots in oat (Avena sativa) plants treated with Si.
However, Gong et al. (2006) evaluated the interaction of Si + NaCl in rice (Oryza sativa) seedlings. Where they observed that Si did not mitigate the adverse effects of NaCl. A positive effect of Si was observed in the development of the stem (Figure 1C), particularly seedlings in saline conditions (50 mM) increased stem length with the presence of Si. While, in the production of dry biomass (Figure 1D), a positive impact of Si was observed in the non-saline situation, showing a quadratic effect. Haghighi and Pessarakli (2013) evaluated the interaction factor of Si + NaCl in cherry tomato. They observed that Si mitigated the adverse effects of NaCl and increased stem length, fresh and dry biomass, photosynthetic rate and water content.

Figure 1 Effect of the interaction of sodium chloride (NaCl) and silicon (Si) on germination rate (A); root length (B); stem length (C); and dry biomass (D) of cherry tomato seedlings after 14 days of establishment of the experiment. The asterisks indicate the levels of significance among treatments 0.01** - 0.05*.
Wang et al. (2015) observed that Si promoted the increase in the length of stem, root, and fresh biomass of cucumber (Cucumis sativus) plants subjected to NaCl stress. On the other hand, Carballo et al. (2019) evaluated the interaction factor of Si + NaCl in the germination of moringa (Moringa oleifera). These results showed a positive effect of Si by increasing biomass, vigor index, total phenols, total flavonoids, and antioxidant capacity.
In the Floradade variety, it was observed that the concentration of 50 mM NaCl reduced the germination rate by 14%. Contrary to the above, the concentration of 25 mM NaCl increased the root length by 19%, while the concentration of 50 mM NaCl increased the dry biomass by 19%, both with respect to the control (Table 3).
In this sense, Camejo and Torres (2000) observed an increase in dry biomass in tomato plants under saline conditions. Plants, when exposed in a saline medium, activate their defense mechanism, and raise the levels of enzymes, prolines, amino acids, and antioxidants. The presence of Si, regardless of the concentration, increased germination rate and root length (Table 3).
Table 3 Main effects of NaCl and Si on germination of Floradade tomato (Solanum lycopersicum var. Floradade).
Dose | Variables evaluated | |||||||
% GP | GE | GRI | MGT | GI | GS | RL | DB | |
NaCl | ||||||||
0 mM | 72.333 | 13.404 | 6.5 a | 0.132 | 0.09 | 0.283 | 4.034 b | 1.44 b |
25 mM | 72.667 | 13.658 | 6.211 ab | 0.133 | 0.091 | 0.284 | 4.814 a | 1.5 b |
50 mM | 68 | 12.925 | 5.585 b | 0.128 | 0.085 | 0.266 | 3.948 b | 1.71 a |
±SE | 2.599 | 0.553 | 0.198 | 0.003 | 0.003 | 0.01 | 0.222 | 0 |
Linear | ns | ns | 0.003 | ns | ns | ns | ns | 0.003 |
Quadratic | ns | ns | ns | ns | ns | ns | 0.005 | ns |
Si | ||||||||
0 mM | 70.333 | 13.523 | 5.458 b | 0.13 | 0.088 | 0.275 | 3.675 b | 1.57 |
1 mM | 71.333 | 13.333 | 6.235 a | 0.131 | 0.089 | 0.279 | 4.479 a | 1.58 |
2 mM | 71.333 | 13.132 | 6.603 a | 0.132 | 0.089 | 0.279 | 4.642 a | 1.49 |
±SE | 2.599 | 0.553 | 0.198 | 0.003 | 0.003 | 0.01 | 0.222 | 0 |
Linear | ns | ns | <0.001 | ns | ns | ns | 0.005 | ns |
Quadratic | ns | ns | ns | ns | ns | ns | ns | ns |
Different literals between the means indicate a significant difference. Germination percentage (%GP); germination energy (GE); germination rate (GRI); mean germination time (MGT); germination index (GI); germination speed (GS); root length (RL); dry biomass (DB); ns= not significant; ±SE= standard error.
The NaCl-Si interaction showed that the presence of Si increased root length and fresh biomass (Figure 2). Khan et al. (2020) evaluated thermotolerance induced by Si in tomato plants at doses of 1 and 2 mM. The results showed that Si significantly improved plant growth, fresh biomass, and root length, compared to the control treatment. Similarly, Mushinskiy et al. (2018) found that the application of Si increased the root length of tomato seedlings by 27.8% compared to the control treatment.
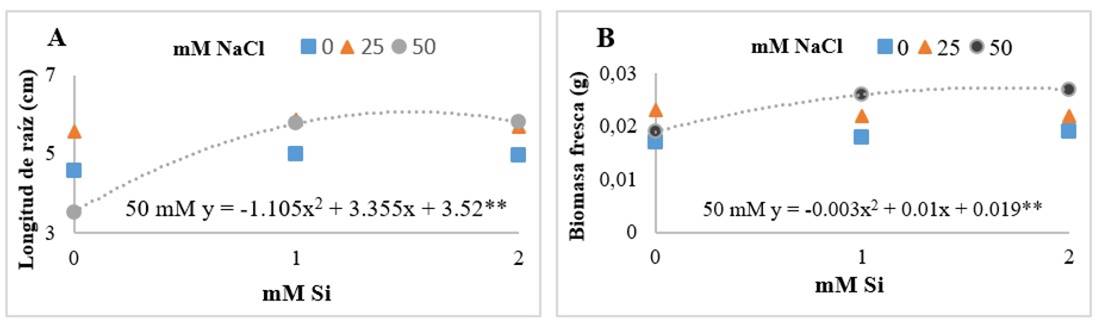
Figure 2 Effect of the interaction of sodium chloride (NaCl) and silicon (Si) on root length (A); and fresh biomass (B) of Floradade tomato seedlings after 14 days of establishment of the experiment. The asterisks (*) indicate significance levels among treatments 0.01** - 0.05*.
In the present study, it was observed that the concentration of 50 mM NaCl significantly reduced the germination rate by 31% compared to the control treatment. Contrary to the above, it was observed that doses of 25 and 50 mM stimulated root length by 36.02 and 27.57% compared to the control treatment. In addition, stem length was also increased by 40% and 26% at these NaCl concentrations (Table 4).
Table 4 Main effects of NaCl and Si on the germination of Río Grande saladette tomato (Solanum lycopersicum var. Río Grande).
Dose | Variables evaluated | ||||||||
% GP | GE | GRI | MGT | GI | GS | RL | SL | DB | |
NaCl | |||||||||
0 mM | 71 | 13.253 | 6.169 a | 0.132 | 0.089 | 0.277 | 5.744 b | 5.351 c | 1.85 b |
25 mM | 67 | 12.879 | 5.13 ab | 0.128 | 0.084 | 0.262 | 7.813 a | 7.494 a | 2 ab |
50 mM | 59.333 | 11.579 | 4.253 b | 0.116 | 0.074 | 0.232 | 7.328 a | 6.766 b | 2.13 a |
±SE | 3.752 | 0.718 | 0.341 | 0.005 | 0.005 | 0.015 | 0.299 | 0.188 | 0 |
Linear | ns | ns | < 0.001 | ns | ns | ns | < 0.001 | < 0.001 | 0.006 |
Quadratic | ns | ns | ns | ns | ns | ns | 0.002 | < 0.001 | ns |
Si | |||||||||
0 mM | 64.333 | 12.558 | 4.61 a | 0.124 | 0.08 | 0.251 | 5.942 b | 5.873 b | 2.11 a |
1 mM | 66.333 | 12.553 | 5.442 a | 0.125 | 0.083 | 0.259 | 7.091 a | 6.68 a | 1.86 b |
2 mM | 66.667 | 12.601 | 5.501 a | 0.126 | 0.083 | 0.26 | 7.853 a | 7.058 a | 2.02 ab |
±SE | 3.752 | 0.718 | 0.341 | 0.005 | 0.005 | 0.015 | 0.299 | 0.188 | 0 |
Linear | ns | ns | ns | ns | ns | ns | < 0.001 | < 0.001 | ns |
Quadratic | ns | ns | ns | ns | ns | ns | ns | ns | 0.016 |
Different literals between the means indicate a significant difference. Germination percentage (%GP); germination energy (GE); germination rate (GRI); mean germination time (MGT); germination index (GI); germination speed (GS); root length (RL); stem length (SL); dry biomass (DB); ns= not significant; ±SE= standard error.
Our results coincide with those obtained by Loudari et al. (2020), who observed the effect of NaCl on tomato, which increased the length and diameter of the root. On the other hand, Batista et al. (2017) evaluated the effect of NaCl on the germination of three varieties of basil (Ocimum basilicum L.). Where they observed a decrease in the percentage and rate of germination. Similarly, González et al. (2020) studied the effect of NaCl on the germination of seeds of tomato cv ‘Río Grande’. They observed that the germination rate decreased significantly compared to the control treatment.
The concentrations of 1 and 2 mM Si had a significant impact by increasing root and stem length, both concentrations were higher than the control treatment (Table 4). It has been shown that Si, despite not being an essential element for plants, favors the growth and development of some species. In this sense, Chourasiya et al. (2021) mention that the effect of Si on the germination and initial growth of wheat (Triticum) has a positive effect on the variables evaluated.
Si significantly increased fresh biomass (Figure 3). Particularly at the concentration of 50 mM NaCl, the presence of Si generated a positive linear effect. Ahmad et al. (2019) evaluated the interaction factor of Si + NaCl in bean seedlings, which improved the adverse effects of NaCl on growth parameters, fresh and dry biomass, pigment synthesis and relative water content.
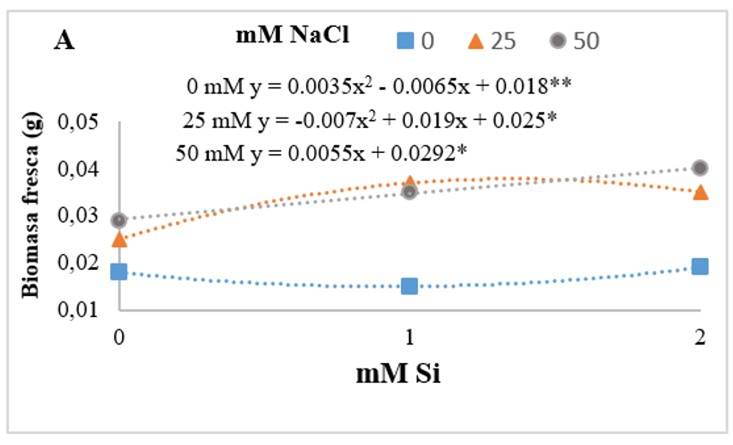
Figure 3 Effect of the interaction of sodium chloride (NaCl) and silicon (Si) on the fresh biomass (A) of Río Grande tomato seedlings after 14 days of establishment of the experiment. The asterisks (*) indicate significance levels among treatments 0.01** - 0.05*.
In addition, Torabi et al. (2012) reported that Si positively impacted on the growth of borage (Borago officinalis) seedlings, thus increasing their fresh and dry biomass. Therefore, Si, once inside the plant, is found in different structures, diffuses through the tissues, and forms a continuous layer between the cuticle and the epidermis composed of two sublayers, one as Si gel and another in a silicon-cellulose complex, which isolates and defends the plant from adverse environmental factors.
In this sense, Emamverdian et al. (2018) mentions that Si has the ability to activate the Lsi1, Lsi2 and Lsi6 genes in plants, the Lsi2 gene is considered an anion transporter and has the ability to express itself in the root endodermis, while Lsi1 and Lsi6 are genes that belong to the aquaporin family and their function is to transport silicon to the tissues of shoots and roots. In addition, when genes express themselves, multiple enzymes are activated, such as: catalases, peroxidases, superoxide dismutases, ascorbate peroxidases and glutathione reductases, which contribute with the plant to relieve stress (biotic or abiotic).
Conclusions
Tomato cultivars showed different effects regarding salinity. The cherry cultivar showed decreases in its germinative variables with increasing salinity, which indicates that the Floradade (beef) and Río Grande (saladette) cultivars had greater tolerance to salinity. Silicon (Si) had a protective effect in the interaction (NaCl + Si), showing positive effects by increasing the variables evaluated. Therefore, Si could be considered as a salinity mitigating agent.
Literatura citada
Ahmad, P. S.; Ahanger, M. A.; Alam, P.; Alyemeni, M. N.; Wijaya, L.; Ali, S. and Ashraf, M. 2019. Silicon (Si) supplementation alleviates NaCl toxicity in mung bean Vigna radiata (L.) Wilczek. Through the modifications of physio-biochemical attributes and key antioxidant enzymes. J. Plant Growth Regul. 38(1):70-82. Doi:10.1007/s00344-018-9810-2. [ Links ]
Ahmed, B.; Zaidi, A.; Khan, M. S.; Rizvi, A.; Saif, S. and Shahid, M. 2017. Perspectives of plant growth promoting Rhizobacteria in growth enhancement and sustainable production of tomato. In: Zaidi, A., Khan, M. Ed. Microbial strategies for vegetable production. Springer, Cham. 125-149 pp. https://doi.org/10.1007/978-3-319-54401-4-6. [ Links ]
Asgari, F.; Majd, A.; Jonoubi, P. and Najafi, F. 2018. Effects of silicon nanoparticles on molecular, chemical, structural and ultrastructural characteristics of oat (Avena sativa L.). Plant Physiol. Biochem. 127(1):152-160. https://doi.org/10.1016/j.plaphy.2018.03.021. [ Links ]
Batista, S. D.; Murillo, A. B.; Nieto, G. A.; Alcaraz, M. L.; Troyo, D. E.; Hernández, M. L. y Ojeda, S. C. 2017. Mitigación de NaCl por efecto de un bioestimulante en la germinación de Ocimum basilicum L. Terra Latinoam. 35(4):309-320. https://www.scielo.org.mx/pdf/ tl/v35n4/2395-8030-tl-35-04-00309.pdf. [ Links ]
Briones, V. O. L.; Búrquez, M. J. A.; Martínez, Y. A.; Pavón, H. N. P. and Perroni, V. Y. 2018. Biomass and productivity in Mexican arid lands. Madera y Bosques. 24(1):1-19. Doi: 10.21829/myb.2018.2401898. [ Links ]
Camejo, L. D. y Torres, E. W. 2000. La salinidad y su efecto en los estados iniciales del desarrollo de los cultivares de tomate (Lycopersicum esculemtum Mill). Cultivos Tropicales. 21(2):23-26. https://www.redalyc.org/pdf/1932/193215024004.pdf. [ Links ]
Can, C. A.; Cruz, C. E.; Ortega, E. H.; Sánchez, B. E.; Madueño, M. A.; Bojórquez, S. J. y Mancilla, V. O. 2017. Respuesta de Phaseolus vulgaris a la salinidad generada por NaCl, Na2SO4 y NaHCO3. Rev. Mex. Cienc. Agríc. 8(6):1287-1300. http://www.redalyc.org/articulo.oa?id=263153306005. [ Links ]
Carballo, M. F. J.; Olivares, S. E.; Bolivar, D. M.; Antonio, B. A.; Vázquez, B. M. E. and Nino, M. G. 2019. Effect of silicon on germination of moringa Oleifera Lam. In different types of salts. Fresenius Environmental Bulletin. 28(11):8823-8830. [ Links ]
Chourasiya, V. K.; Nehra, A.; Shukla, P. S.; Singh, K. P y Singh, P. S. 2021. Impacto de la nanosílice mesoporosa (SiO2) en la germinación de semillas y el crecimiento de plántulas de semillas de trigo, guisantes y mostaza. Diario de nanociencia y nanotecnología. 21(6):3566-3572. Doi: https://doi.org/10.1166/jnn.2021.19013. [ Links ]
Cuartero, J. M.; Bolarín, M. C.; Asíns, M. J. and Moreno, V. V. 2006. Increasing salt tolerance in the tomato. J. Exp. Bot. 57(5):1045-1058. Doi: https://doi.org/10.1093/jxb/erj102. [ Links ]
Doğan, M.; Avu, A.; Can, E. N. and Aktan, F. A. 2008. Farklı domates tohumlarının çimlenmesi üzerine tuz stresinin etkisi. SDÜ Fen Edebiyat Fakültesi Fen Dergisi. 3(2):174-182. https://dergipark.org.tr/tr/download/article-file/116274. [ Links ]
Durukan, D. H. and Demirbas, A. A. 2018. The effects of different salt doses on yield and nutrient uptake of tomato plant. Sci. Pap. Ser. A Agron. 61(1):71-76. [ Links ]
Eitel, C. C. 2021. Efecto del estrés salino en la producción y calidad de semillas de tomate. Tesis para optar al grado de Magister en Fisiología y producción Vegetal. 1-47 pp. https://bibliotecadigital.oducal.com/Record/ir-11534-52676. [ Links ]
Emamverdian, A.; Ding, Y.; Xie, Y. y Sangari, Y. 2018. Mecanismos de silicio para mejorar el estrés por metales pesados en plantas. Biomed. Res. Int. 1(1):1-10. Doi:10.1155/2018/ 8492898. [ Links ]
FIRA. 2019. Fideicomisos Instituidos en Relación con la Agricultura (FIRA). Panorama Agroalimentario. Dirección de Investigación y evaluación económica y sectorial. https://www.inforural.com.mx/wp-content/uploads/2019/06/Panorama-Agroalimentario-Tomate-rojo-2019.pdf. [ Links ]
Gong, H. J.; Randall, D. P. y Flores, T. J. 2006. La deposición de silicio en la raíz reduce la absorción de sodio en las plántulas de arroz (Oryza sativa L.) al reducir el flujo de derivación. Planta, Célula y Medio Ambiente. 29(10):1970-1979. Doi: https://doi.org/10.1111/j.1365-3040.2006.01572.x. [ Links ]
González, G. P.; Suárez, N. T. and Marín, J. O. 2020. Effect of salinity and seed salt priming on the physiology of adult plants of Solanum Lycopersicum cv. ‘Río Grande’. Braz. J. Bot. 43(4):775-787. Doi: https://doi.org/10.1007/s40415-020-00636-1. [ Links ]
Haghighi, M.; Afifipour, Z. and Mozafarian, M. 2012. The effect of N-Si on tomato seed germination under salinity levels. J. Biol. Environ. SCI. 6(16):87-90. https://uludag.edu.tr/dosyalar/jbes/16/mak12.pdf. [ Links ]
Haghighi, M. and Pessarakli, M. 2013. Influence of silicon and nano-silicon on salinity tolerance of cherry tomatoes (Solanum lycopersicum L.) at early growth stage. Sci. Hortic. 161(1):111-117. https://doi.org/10.1016/j.scienta.2013.06.034. [ Links ]
Infoagro. 2017. El cultivo del tomate. http://www.infoagro.com/hortalizas/tomate.htm. [ Links ]
Khan, A. M.; Khan, A. L.; Imran, M. A.; Asaf, S. A.; Kim, Y. H.; Bilal, S.; Numan, M.; Al-Harrasi, A.; Al-Rawahi, A. and Lee, I. J. 2020. Silicon induced thermotolerance in Solanum lycopersicum L. via activation of antioxidant system, heat shock proteins, and endogenous phytohormones. BMC Plant Biol. 20(1):248-267. https://doi.org/10.1186/ s12870-020-02456-7. [ Links ]
Loudari, A.; Benadis, C.; Naciri, R.; Soulaimani, A.; Zeroual, Y.; Gharous, M.; Kalaji, H. M. and Oukarroum, A. 2020. Salt stress affects mineral nutrition in shoots and roots and chlorophyll a fluorescence of tomato plants grown in hydroponic culture. J. Plant Interac. 15(1):398-405. Doi:10.1080/17429145.2020.1841842. [ Links ]
Mazón, S. M.; Ojeda, S. C.; García, B. M.; Batista, S. D. y Abasolo, P. F. 2020. La Homeopatía incrementa la tolerancia al estrés por NaCl en plantas de frijol común (Phaseolus vulgaris L.) variedad Quivicán. Terra Latinoam. 38(1):37-51. Doi: doi.org/10.28940/terra. v38i1.584. [ Links ]
Mushinskiy, A. A.; Aminovа, E. V. y Korotkova, A. M. 2018. Evaluación de la tolerancia de los tubérculos Solanum tuberosum a las nanopartículas de sílice. Environ. Sci. Pollut. Res. 25(34):34559-34569. https://doi.org/10.1007/s11356-018-3268-4. [ Links ]
Ruiz, E. F.; Villalpando, G. R. L.; Murillo, B. A.; Beltrán, M. F. A. y Hernández, M. L. G. 2014. Respuesta diferencial a la salinidad de genotipos de tomate (Lycopersicon esculentum Mill.) en primeras etapas fenológicas. Terra Latinoam. 32(4):311-323. https://www.scielo.org.mx/pdf/tl/v32n4/2395-8030-tl-32-04-00311.pdf. [ Links ]
Sun, Y.; Xu, J.; Miao, X.; Lin, X.; Liu, W. and Ren, H. 2021. Efects of exogenous silicon on maize seed germination and seedling growth. Scientifc Reports. 11(1):1-13. Doi: https://doi.org/10.1038/s41598-020-79723-y. [ Links ]
Torabi, F.; Majd, A. and Enteshari, S. 2012. Effect of exogenous silicon on germination and seedling establishment in Borago officinalis L. J. Medicinal Plants Res. 6(10):1896-1901. https://academicjournals.org/journal/JMPR/article-full-text-pdf/4A0962632431. [ Links ]
Turhan, A. N.; Kuşçu, H. D. and Şeniz, K. V. 2011. Effects of different salt concentrations (NaCl) on germination of some spinach cultivars. J. Agricultural Faculty of Uludag University. 25(1):65-77. https://dergipark.org.tr/tr/download/article-file/154131. [ Links ]
Vítámvás, P. V.; Kosová, K. I. and Prášil, T. I. 2007. Proteome analysis in plant stress research: a review. Czech J. Genet. Plant Breed. 43(1):1-6. Doi: https://doi.org/10.17221/1903-CJGPB. [ Links ]
Wang, S.; Liu, P.; Chen, D.; Yin, L.; Li, H. y Deng, X. 2015. Silicon enhanced salt tolerance by improving the root water uptake and decreasing the ion toxicity in cucumber. Front. Plant Sci. 6(1):1-10. https://doi.org/10.3389/fpls.2015.00759. [ Links ]
Received: November 01, 2022; Accepted: January 01, 2023