Servicios Personalizados
Revista
Articulo
Indicadores
-
Citado por SciELO
-
Accesos
Links relacionados
-
Similares en SciELO
Compartir
Revista mexicana de ciencias agrícolas
versión impresa ISSN 2007-0934
Rev. Mex. Cienc. Agríc vol.12 no.8 Texcoco nov./dic. 2021 Epub 02-Mayo-2022
https://doi.org/10.29312/remexca.v12i8.2742
Articles
Polyphenols from different plant sources and their in vitro effect against chickpea pathogens
1Universidad Autónoma Agraria Antonio Narro. Calzada Antonio Narro núm. 1923, Col. Buenavista Saltillo, Coahuila. CP. 25315. Tel. 844 4110327.
2Campo Experimental Norman E. Borlaug-INIFAP. Calle Dr. Norman E. Borlaug km 12, Cd. Obregón, Sonora. CP. 85000. (ivonn1590@hotmail.com).
3Departamento de Facultad de Ciencias Químicas-Universidad Autónoma de Coahuila. Ing. Cárdenas Valdez s/n, Col. República, Saltillo, Coahuila. CP. 25280. Tel. 844 4161238. (b-clic@hotmail.com; saira.mtz.aleman@hotmail.com; alberto-ascaciovaldes@uadc.edu.mx; rrh961@hotmail.com).
The production of chickpea (Cicer arietinum L.) is constantly compromised by a complex of pathogens that cause root wilt and rot (RWR). Some of the strategies used for the management of this disease are the use of resistant varieties, crop rotation, solarization, removal of regrowths and use of seeds free of pathogens or treated with fungicides, although the results have been limited or not very satisfactory, in recent years, biological control and organic products have become more important. In the present work, polyphenols were obtained from ethanolic extracts by the ultrasound-microwave-assisted technique of plant species: thunder (L. lucidum) leaf, sorghum (S. bicolor) grains and moringa (M. oleifera) leaves. The corresponding qualitative analysis was carried out using HPLC masses and the antifungal effect of each group of polyphenol extracts on three phytopathogenic fungi that make up the complex of root wilt and rot was determined by means of the technique of plate dilution and poisoned medium. The percentage of inhibition and the inhibitory concentration (IC50) were determined. The results indicate that polyphenols have high biological effectiveness on the fungus Macrophomina and Fusarium solani, the activity for Fusarium oxysporum f. sp. ciceris depended on the polyphenols of each plant species; polyphenols of L. lucidum with a concentration of 491.99 ppm. Additionally, it was found that all the groups of polyphenols had in their chemical composition some compounds of recognized microbial activity such as: flavones, anthocyanins, catechins and alkylphenols, among others.
Keywords: Fusarium; Macrophomina; polyphenols
La producción del garbanzo (Cicer arietinum L.) constantemente está comprometida por un complejo de patógenos que ocasionan la marchitez y pudrición de raíz (MPR). Algunas de las estrategias empleadas para el manejo de esta enfermedad son el uso de variedades resistentes, rotación de cultivos, solarización, retiro de socas y uso de semillas libre de patógenos o tratadas con fungicidas, aunque los resultados han sido limitados o poco satisfactorios, en los últimos años, el control biológico y los productos orgánicos ha cobrado mayor importancia. En el presente trabajo se obtuvieron polifenoles de extractos etanólicos mediante la técnica asistida de ultrasonido- microondas de las especies vegetales: hoja de trueno (L. lucidum), granos de sorgo (S. bicolor) y hojas de moringa (M. oleífera). Se les realizó el correspondiente análisis cualitativo mediante HPLC masas y se determinó el efecto antifúngico de cada grupo de polifenoles extracto sobre tres hongos fitopatógenos que conforman el complejo de la marchitez y pudrición de raíz, por medio de la técnica de dilución en placa y medio envenenado. Se determinó el porcentaje de inhibición y la concentración inhibitoria (CI50). Los resultados indican que los polifenoles tienen elevada efectividad biológica sobre el hongo Macrophomina y Fusarium solani, la actividad para Fusarium oxysporum f. sp. ciceris dependió de los polifenoles de cada especie vegetal; polifenoles de L. lucidum con una concentración de 491.99 ppm. Adicionalmente se comprobó que todos los grupos de polifenoles tenían en su composición química algunos compuestos de reconocida actividad microbiana como lo son: flavonas, antocianinas, catequinas y alquifenoles, entre otros.
Palabras clave: Fusarium; Macrophomina; polifenoles
Introduction
Chickpea (Cicer arietinum L.) crop ranks second worldwide among dry-grain legumes, only after the bean (Phaseolus vulgaris L.) (Morales and Durón, 2004). In Mexico, in the agricultural year 2016, the area destined to the production of this crop was 66 096 ha sown, with a production of 116 076 t; Sinaloa and Sonora were the main producing states, contributing 45 and 30% of the national production, respectively (SIAP, 2017). Among the diseases that limit chickpea production is Fusarium wilt, it is caused by Fusarium oxysporum f. sp. ciceris (FOC). Production losses from 10 to 15% are reported in Spain and in other countries between10 and 90% (Landa et al., 2006; Sharma and Muehlbauer, 2007).
However, Fusarium wilt can cause 100% losses when the crop is exposed to adverse conditions such as heat or water stress during the reproductive phase and capsule development (Landa et al., 2006). For a long time, the ‘rabies’ caused by Didymella rabiei (Kovatsch.) was considered the most important chickpea disease in Spain (Arx Navas et al., 1998). However, other studies indicate that it occurs occasionally, while ‘drying’ or ‘fusariosis’ has increased. It is suggested that fusariosis could include a pathogen complex similar to root wilt and rot (RWR), which severely reduces chickpea seed production, several fungi have been reported as the agents of the chickpea RWR complex, among which Fusarium, Rhizoctonia, Macrophomina and Sclerotium are mentioned (Padilla et al., 2008).
Some of the strategies used for the management of this disease are the use of resistant varieties, crop rotation, solarization, the removal of regrowths and the use of seeds free of pathogens or treated with fungicides, although the results have been limited or not very satisfactory (Paredes et al., 2009). Chemical control is the most widely used method in the control of diseases caused by Fusarium species; however, in recent years, biological control has become more important (Basco et al., 2017). Various investigations focus on studies on the formulation of natural products with some type of biological activity on phytopathogenic fungi with an increasingly and faster efficacy and action, which is generating that these practices are more effective in a wide range of environmental conditions, pest species and crop systems (Gakuya et al., 2013).
The importance of plant species and their derivatives (extracts, essential oils, decoctions, secondary metabolites) in crop protection under the concept of integrated pest and disease management (IPDM) is recognized (Sparks et al., 2017). Therefore, the objective of this work was to evaluate in vitro the biological effectiveness of polyphenols of thunder, moringa and sorghum obtained by ethanolic extracts against fungi associated with chickpea wilt and rot.
Materials and methods
Obtaining of plant material
Fresh thunder leaves (Ligustrum lucidum) were collected at the Antonio Narro Autonomous Agrarian University (3532º north latitude, 101.0332º west longitude, 1 786 masl), moringa leaves (Moringa oleifera) were obtained in Ciudad Obregón, Sonora (27° 22’ 04” north latitude, 109° 55’ 28” west longitude, 37 masl), sorghum (Sorghum bicolor) grains were obtained from the BRS-72 variety (with high tannin content) in the City of Tecomán, Colima (18° 54’ 51” north latitude, 103° 52’ 30” west longitude, 33 masl). The plant material was transferred to the laboratories of the Food Department of the Faculty of Chemical Sciences, where leaves and grains were selected based on size and without the presence of damage, to be subjected to dehydration for a period of two weeks at ambient temperature, then pulverized and sieved (RO-TAP; TYLER) using a 150 μ sieve.
Ultrasound-microwave-assisted extraction
The pulverized plant material was placed in the reactor with the quantities obtained from the ratios shown in the (Table 1) and this reactor was introduced into the ultrasound and microwave equipment (Nanjing ATPIO Instrumens Manufacture Co., Ltd Company, China) under the following conditions: Ultrasonic (VS): Power Radio 20, Ultrasonic on Relay 10, Ultrasonic off Relay 3, Amplitude off Relay 25 and Set Time 20. Microwave (MV): Power Radio 800, Display power 0, Set Temp 70 °C and Holding Time 5. After the ultrasound and microwave process, the samples were stored in a deep freezer at a temperature of -70 °C.
Amberlite column chromatography
Column chromatography was performed with the amberlite XAD-16N chromatographic filler. This includes activation of amberlite with methanol, column packing, filtration of extracts, drying of extracts and unpacking of the chromatography column (De Asmundis et al., 2011).
Recovery of dry extract
Once the ethanolic fraction was obtained, it was distributed in glass containers and dried in an oven at 60 °C, without exposure to light for 24 to 48 h. Finally, the dry extract in powder form was collected in an amber jar at ambient temperature for later analysis.
Characterization of phytochemicals present in plant extracts by reverse-phase liquid chromatography (HPLC masses)
The analysis by reverse-phase high-performance liquid chromatography was performed following the methodology of Ascacio et al. (2016), which consists of using a Varian HPLC system that includes an automatic injector (Varian ProStar 410, USA), a ternary pump (Varian ProStar 2310, USA) and a PDA decanter (Varian ProStar 330, USA). A liquid-chromatography ion-trap mass spectrometer (Varian 500-MS IT Mass Spectrometer, USA) equipped with an electrospray ion source was also used.
Samples (5 μl) were injected into a Denali C18 column (150 mm x 2.1 mm, 3 μm, Grace, USA). The temperature of the furnace was maintained at 30 °C. The eluents were formic acid (0.2%, V/V; solvent A) and acetonitrile (solvent B). The following gradient was applied: initial, 3% B; 0-5 min, 9% B linear; 5-15 min, 16% B linear; 15-45 min, 50% B linear. After the column was washed and reconditioned, the flow rate was maintained at 0.2 ml min-1 and the elution was controlled at 245, 280, 320 and 550 nm. All effluent (0.2 ml min-1) was injected into the mass spectrometer source, without dividing.
All MS experiments were conducted in negative mode [M-H]-1. Nitrogen was used as a nebulizer gas and helium as a buffer gas. The parameters of the ion source were: spray voltage 5 kV and capillary voltage and temperature were 90 V and 350 °C, respectively. Data was collected and processed using the MS Workstation Software (V 6.9). Samples were first analyzed in full scan mode acquired in the range m/z 50-2000.
Obtaining and identification of fungi
The strains used in the bioassays were isolated from chickpea plants with symptoms of wilting and yellowing, located in the Yaqui Valley at latitude 27° 29.185’, west longitude -109° 56.45’. Small pieces of plant roots with symptoms of the disease were disinfected with a 3% chlorine solution and rinsed with sterile distilled water. They were placed in Petri dishes with potato-dextrose-agar (PDA) culture medium and incubated for three days at 28 °C. Finally, the isolation was carried out by means of a hypha tip.
Molecular identification by PCR and random amplification of polymorphic DNA (RAPD)
For the extraction of DNA from each of the isolates of the fungi, pure mycelium cultures grown in 50 mL of dextrose potato broth, incubated at 25 °C for eight days, were used. At the end of this time, the cultures were centrifuged at 10 000 rpm for 10 min at ambient temperature, the supernatant was discarded, and the cell pellet was washed with PBS. The lysis of the sample was performed with 3 freeze-heating cycles (-80 °C, 75 °C) for 15 min, maceration and incubation for 15 min in TES buffer (Tris-HCl 50 Mm pH 7.5, EDTA 20 Mm, SDS 1%).
The total DNA of the mycelium was obtained by purification with the phenol-chloroform-isoamyl alcohol (25:24:1) method and precipitated with ethanol. The concentration and quality of the genetic material was determined by spectrophotometry (EPOCH; BioTek). For the identification of M. phaseolina and F. solani, specific oligonucleotides were used, which amplify for a conserved region adjacent to the 5.8 S gene and the TEF-1α gene, respectively (Babu, 2007; Arif, 2012).
Amplification was performed by PCR with the Taq DNA polymerase enzyme (qARTA Bio) and 100 ng μl-1 of genetic material, using the amplification conditions described by these authors: 25 denaturation cycles at 95 °C for 30 s, alignment at 56 °C for 1 min, extension at 72 °C for 2 min, and a final extension step at 72 °C for 10 min for M. phaseolina and 40 denaturation cycles at 94 °C for 1 min, alignment at 58 °C for 1 min, extension at 72 °C for 2 min for F. solani.
In addition, the identification of pathogenic races of Fusarium oxysporum f. sp. ciceris was performed using the random amplification of polymorphic DNA (RAPD) technique using the markers OPF and OPI, where the races are distinguished by the absence or presence of electrophoretic patterns with the same mobility, the amplification conditions were as follows, denaturation at 94 °C for 4 min, followed by 30 cycles of 94 °C for 1 min, alignment at 40 °C for 1 min, extension at 72 °C for 3 min and final extension at 72 °C for 7 min (Jiménez-Gasco, 2001). The amplified products were separated by 1% agarose gel electrophoresis (UltraPure Agarose Invitrogen), in TAE buffer. The gels were stained with nucleic acid gel stain (Invitrogen). The corresponding bands of the amplified products were observed with UV light. Images were captured with a digital camera (UVP; GDS-8000).
Antifungal activity of plant extracts against M. phaseolina by the poisoned medium method
Antifungal activity was determined by the poisoned medium method proposed by Jasso de Rodríguez et al. (2011), the treatments of the polyphenols obtained from: Ligustrum lucidum, Moringa oleifera and Sorghum bicolor were in concentrations of 50 mg L-1, 100 mg L-1, 200 mg L-1, 300 mg L-1, 400 mg L-1, 500 mg L-1 and 600 mg L-1: with four repetitions per treatment. The concentration and volume for each of the polyphenols was first determined and added to a flask with the required amount of sterile PDA. Subsequently, discs of 0.4 cm in diameter with active mycelium of the fungus of seven days of growth were placed, finally they were incubated at 28 ±2 °C until the absolute control filled the box completely. The percentage of inhibition was determined by the following formula: percentage of inhibition= (DC-DT/DC)*100. Where DC is the diameter of the control treatment, and DT is the diameter of the different concentrations.
Antifungal activity of plant extracts against F. oxysporum f. sp. ciceris and F. solani by the plate microdilution method
The plate microdilution method adapted from the techniques proposed by Masoko et al. (2005) and Gabrielson et al. (2002) was used. For this, 96-well polystyrene plates were used. The procedure started by placing 100 μl of sabouraud liquid medium in each well of the plate, then each group of polyphenols was prepared at a concentration of 2 000 mg L-1 using ethanol as a solvent, then 100 μl of the polyphenols prepared at 2 000 mg L-1 were placed in column four, mixed and 100 μl were retaken and transferred to the next column and so on, making 50% serial dilutions up to column 12, resulting in concentrations of 1 000 mg L-1, 500 mg L-1, 250 mg L-1, 125 mg L-1, 62.5 mg L-1, 31.2 mg L-1, 15.6 mg L-1, 7.8 mg L-1 and 3.9 mg L-1, the next step was to add 40 μl of 2,3,5- triphenyltetrazolium chloride as a growth developer to all wells; finally from column two, a solution of spores of F. oxysporum and F. solani at a concentration of 1x108 was placed.
Each plate was considered a repetition, and three repetitions were performed per treatment, incubated at 28 °C for 48 h and finally an absorbance reading was performed at 490 nm in spectrophotometer. Growth of the fungus was considered positive in the treatments where the well presented a pink color and negative in those that did not present any color, in addition to their respective absorbance values. The percentage of inhibition was calculated by adapting the formula proposed by Moreno-Limón et al. (2011), considering that the percentage of inhibition is the inverse of the percentage of growth. Growth percentage= (A-B) /C (100). Where: A= treatment absorbance, B= negative control absorbance, C= positive control absorbance. Inhibition percentage= 100 - growth percentage.
Statistical analysis
The experiments were established under a completely randomized block design with three repetitions. A Probit analysis was performed to determine the inhibitory concentration (IC50) of each polyphenol group, then an analysis of variance was performed with inhibitory concentrations (IC50) and the means of the treatments were compared using the Tukey multiple range test (α< 0.05).
Results and discussion
Phytochemicals present in plant extracts, characterized by reverse-phase liquid chromatography (HPLC)
Among the results obtained by HPLC, the presence of compounds from the family of flavones, hydroxycinnamic acid and anthocyanins can be observed more frequently (Table 2).
Table 2 Groups of polyphenols detected in extracts from three plant sources using high-performance liquid chromatography technology coupled with mass spectrometry (HPLC-MS).
Ethanolic extract | Mass | Retention time | Compound | Family |
Sorghum bicolor 1:8 70% ethanol | 341 | 2.856 | 3,4-DHPEA-EA | Tyrosol |
340.9 | 3.652 | Caffey glucose | Hydroxycinnamic Acid | |
304.7 | 9.431 | (+)-Gallocatechin | Catechin | |
304.8 | 23.776 | (-)-Epigallocatechin | Catechin | |
252.9 | 26.878 | 7,4’-Dihydroxyflavone | Flavones | |
268.8 | 44.509 | 7,3’,4’-Trihydroxyflavone | Flavones | |
564.1 | 54.302 | Pelargonidin 3-O-sambubioside | Anthocyanins | |
540.1 | 55.293 | Oleuropein (possibility) | Tyrosol | |
566.1 | 55.731 | Phloretin 2’-O-xylosyl-glucoside | Dihydrochalcones | |
325 | 56.896 | p-Coumaroyl glucose | Hydroxycinnamic Acid | |
Moringa oleifera 1:16 70% ethanol | 304.2 | 18.67 | 1-caffeoylquinic acid | Hydroxycinnamic Acids |
304.1 | 19.22 | 3-caffeoylquinic acid | Hydroxycinnamic Acids | |
341 | 2.28 | p-Coumaroyl tartaric acid | Hydroxycinnamic Acids | |
564.2 | 25.48 | Quercetin 3-O-galactoside | Hydroxycinnamic Acids | |
310 | 32.82 | Quercetin 3-O-glucoside | Flavonol | |
310 | 32.82 | Quercetin 4’-O-glucoide | Flavonol | |
755 | 35.29 | Peonidin 3-O-(6”-acetyl-galactoside) | Flavonol | |
755 | 36.5 | Peonidin 3-O-(6”-acetyl-glucoide) | Anthocyanins | |
252.8 | 28.43 | Delphinidin 3-O-galactoside | Anthocyanins | |
540.1 | 51.35 | Bisdemethoxycurcumin | Curcuminoids | |
Ligustrum lucidum 1:16 70% ethanol | 592.8 | 30.55 | Apigenin 6,8-di-C-glucoside | Flavones |
310.9 | 32.95 | Caffeoyl tartaric acid | Hydroxycinnamic Acids | |
755 | 34.67 | Quercetin 3-O-rhamnosyl-rhamnosyl-glucoside | Flavones | |
346.9 | 39.28 | 5-Heptadecylresorcinol | Alkylphenols |
Identification and molecular characterization of phytopathogenic fungal isolates
The strain of Macrophomina developed colonies with the following characteristics: dense mycelial growth, hairy in appearance, at first dark gray and subsequently it turned black. The colony developed microsclerotia, these characteristics were similar to those of M. phaseolina (Santos-Mier et al., 2015). For the fungal isolate analyzed by PCR, amplification of fragments of a size of 350 bp was achieved, this confirms that the strain belongs to the species M. phaseolina, since the indicators MpKF1 and MpKR1 are specific for this species of fungus.
According to Babu et al. (2007), DNA sequences obtained with the specific primers MpKFI and MpKRI can be used to quickly, selectively and specifically identify the fungus M. phaseolina. Similarly, Zhang et al. (2011) and Sánchez et al. (2013) used the specific primers MpKFI and MpKRI for the identification and detection of M. phaseolina isolates in strawberry and mung bean (Vigna radiata L.), respectively (Figure 1a). For the identification and discrimination of F. solani, the TEFs4 primer set was used, with which the TEF-1α gene was amplified, showing a product of 658 bp (Figure 1b), as reported by Arif et al. (2012).
Finally, for the identification of pathogenic races 0, 1B/C, 5 and 6 of Fusarium oxysporum f. sp. Ciceris by the RAPD technique, seven specific oligonucleotides called OPF and OPI were used (Jiménez-Gasco, 2001). All isolated samples were examined, and the banding pattern was evaluated. Only in one sample an amplification product at 900 bp with the primer OPF-10 was observed (Figure 1c), this product corresponds to race 5 according to what was previously reported by Del Mar Jiménez et al. (2001).
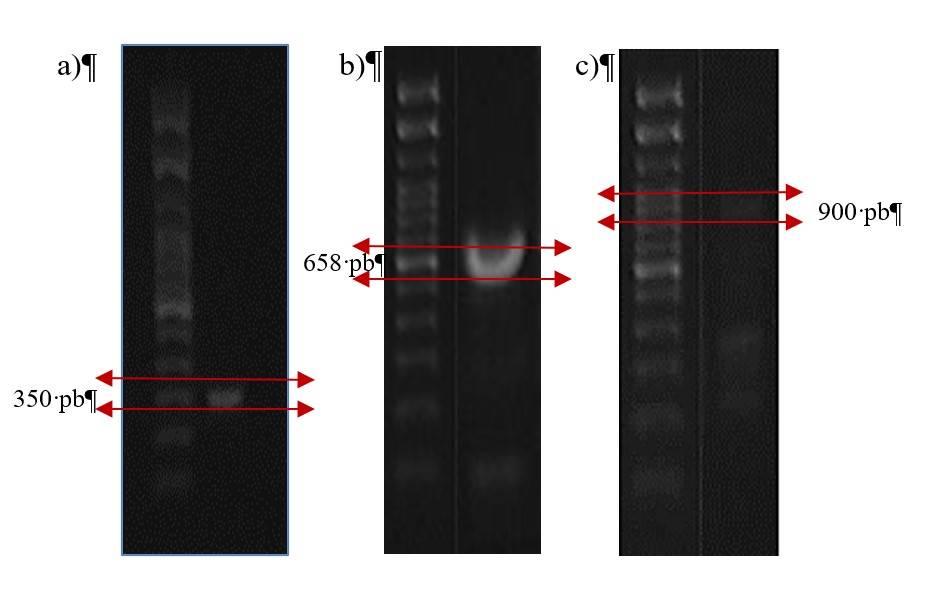
Figure 1 Identification of pathogens: a) M. phaseolina (350 bp); b) F. solani (658 bp); and c) F. oxysporum f. sp. ciceris (900 bp). Amplification products separated in a 1% agarose gel and stained with Nucleic acid gel stain (Invitrogen).
The results of other studies have shown that, in the place where the isolates were carried out, different races have been identified in different sampling areas (Arvayo-Ortiz et al., 2011). Therefore, the results obtained by our work team are consistent with these findings.
Antifungal activity of plant extracts against M. phaseolina by the poisoned medium method
The effect of polyphenols from different plant sources on the growth of the fungus M. phaseolina is shown in Figure 2. As can be seen, the polyphenols of L. lucidum and S. bicolor showed total inhibition on the mycelial growth of the fungus from 300 ppm, while the polyphenols of M. oleifera at the same concentration managed to inhibit 94.5% of the mycelial growth. IC50 ranged from 53.65 ppm M. oleifera to 78.09 ppm S. bicolor.

Figure 2 Percentage of inhibition of polyphenols obtained by ethanolic extracts from plant sources on M. phaseolina.
All polyphenols showed inhibition of fungal growth, with significant differences from control treatment. The percentages of inhibition obtained in this work were higher than those reported by Abreu et al. (2015), who, in their research, managed to obtain an inhibition of 60% using ethanolic extracts from paradise (Melia azedarach L), moringa (Moringa oleifera) and neem (Azadirachta indica A. Juss) against Macrophomina phaseolina, while in the present work, an inhibition of 100% was obtained using polyphenols separated by column chromatography.
Alkaloids have an important role in the defensive strategies of plants against pathogens, such as the repair of damage by the antioxidant system (Matsuura et al., 2017) and the establishment of defensive barriers of a biochemical nature (Zacarés, 2008). In addition to being sometimes induced through the jasmonate signaling pathway (Matsuura et al., 2017), which would explain the positive effect of treatments on the control of Macrophomina.
The obtaining of positive results of this work is associated with the technique of obtaining polyphenols because it is a technique in which good yields are shown in short periods and at low temperatures, showing a hydration process that favors the extraction of certain substances, since ultrasound hydrates the lamella (present in the membranes of plant cells) and once the lamella is disintegrated, plant cells are exposed to the solvent extraction process (Toma et al., 2001).
Antifungal activity of plant extracts against F. oxysporum f. sp. ciceris by the plate microdilution method
The antifungal activity of the selected polyphenols of Ligustrum lucidum (leaves), Moringa oleifera (leaves) and Sorghum bicolor (grains) were tested against Fusarium oxysporum f. sp. ciceris (Figure 3). The results showed that the three groups of polyphenols used inhibit the growth of the pathogen between 60 and 85% at 1 000 ppm. Similarly, the three groups of polyphenols at low concentrations did not present inhibition of the growth of the pathogen, it should be noted that the polyphenols of Ligustrum lucidum were those that showed the highest percentage of inhibition with 83.63%, followed and with a minimum difference by the group of polyphenols of Moringa oleifera with 82.86%, while Sorghum bicolor only inhibited 68.3%.
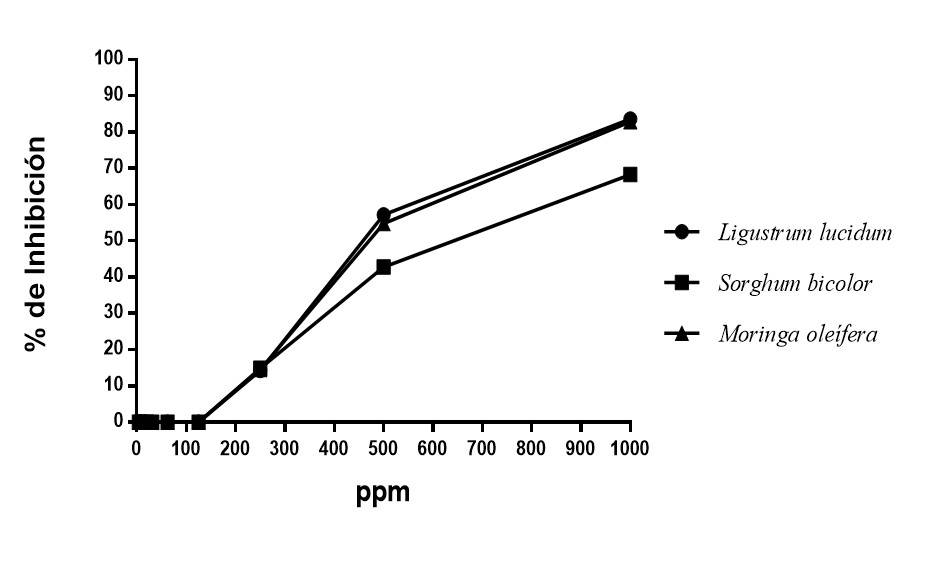
Figure 3 Percentage of inhibition of polyphenols from different plant sources against Fusarium oxysporum f. sp. Ciceris.
The percentages of fungal inhibition obtained in the present study using groups of polyphenols were higher than those reported by Dwivedi and Sangeeta (2015), according to their results, the extract of Moringa oleifera, Tinospora cardiofolia and Cymbopogon citratus against Fusarium oxysporum f. sp. ciceris reach an inhibition of 60.29%, in the same way, the results reported by Chandra and Singh (2005) with different plant extracts with which only 65% of inhibition of Fusarium oxysporum f. sp. Ciceris was reached. The analysis of variance showed significant differences between the IC50 of the three groups of polyphenols, being those obtained from Ligustrum lucidum those that promoted the lowest IC50 and statistically the best of the three extracts with 492.02 ppm (Table 3).
Table 3 50% inhibitory concentration (IC50) of polyphenols obtained from various plant sources for the inhibition of Fusarium oxysporum f. sp. Ciceris.
Plant source | IC50 | Df | F | p-value |
Sorghum bicolor | 628.82 a | 3 | 82629272.98 | <0.0001 |
Moringa oleifera | 502.22 b | |||
Ligustrum lucidum | 491.99 c |
CV= 0.01. Values with the same letter are statistically equal (Tukey 0.05). LSD= 0.13829.
Antifungal activity of polyphenols of different plants against F. solani by the plate microdilution method
The effect of polyphenol groups on Fusarium solani was determined by the percentage of inhibition. The analysis of variance of the inhibitory concentration shows significant differences between the treatments, being the extract of Ligustrum lucidum the one that showed the lowest IC50 (Table 4). The polyphenols of ethanolic extracts of Ligustrum lucidum, Moringa oleifera and Sorghum bicolor inhibited 100% of the growth of Fusarium solani at the concentration of 1 000 ppm, the rest of the concentrations continued to show inhibition, being the polyphenols of Ligustrum lucidum the most relevant.
Table 4 50% inhibitory concentration (IC50) of polyphenols from different plant sources for the inhibition of Fusarium solani.
Plant source | IC50 | Df | F | p-value |
Sorghum bicolor | 94.97 a | 3 | 1574972.80 | <0.0001 |
Moringa oleifera | 78.03 b | |||
Ligustrum lucidum | 54.22 c |
CV= 0.08. Values with the same letter are statistically equal (Tukey 0.05). LSD= 0.12876
The above is relevant if it is considered that there are chemicals that are applied one or more times a week and do not achieve these results, even under laboratory conditions they do not manage to inhibit 100% the growth of the mycelium, as reported by Yossen and Conles (2016), who, in their work with commercial molecules, reach an inhibition between 60 and 97%. Similar results to ours on Fusarium spp. (Figure 4) were reported by Duarte et al. (2013), who, with essential oils of Piper aduncum subsp. ossanum and Piper aurintum, totally inhibit the growth of this fungus. On the other hand, Zaker (2014) concluded that the ethanolic extract of Artemisia annua leaves can inhibit the growth of F. solani.
Conclusions
The polyphenols of thunder (Ligustrum lucidum), moringa (Moringa oleifera) and sorghum (Sorgum bicolor) obtained by ultrasound-microwave-assisted extraction were effective in inhibiting 100% the growth of M. phaseolina and F. solani, while for the inhibition of Fusarium oxysporum f. sp. Ciceris, the polyphenols of Ligustrum lucidum were the most effective and the lowest inhibitory concentration (IC50) 491.99 ppm. Similarly, it is concluded that the polyphenols of Ligustrum lucidum manage to inhibit the growth of the phytopathogens evaluated, at lower concentrations than the other sources of extraction. This is the first report of Ligustrum lucidum used for plant disease control.
Acknowledgements
We thank the National Council for Science and Technology (CONACYT, for its acronym in Spanish) and the Antonio Narro Autonomous Agrarian University (UAAAN, for its acronym in Spanish) for the financial support for the realization of this work.
REFERENCES
Arif, M.; Chawla, S.; Zaidi, M. W.; Rayar, J. K.; Variar, M. y Singh. 2012. Desarrollo de cebadores específicos para los géneros Fusarium y F. solani utilizando la subunidad de ADNr y el gen del factor de elongación de la transcripción (TEF-1α). Rev. Afric. Biotecn. 11(2):444-447. https://www.researchgate.net/publication/230555127. [ Links ]
Arvayo, O. R. M.; Esqueda, M.; Acedo F. E.; Sánchez, A. and Gutiérrez, A. 2011. Morphological variability and races of Fusarium oxysporum f. sp. ciceris associated with chickpea (Cicer arietinum) crops. Am. J. Agric. Biol. Sci. 6(1):114-121. https://www.researchgate.net/publication/280020049 . [ Links ]
Ascacio, V. J. A.; Aguilera, C. A. F.; Buenrostro, J. J.; Prado, B. A.; Rodríguez, H. R. and Aguilar, C. N. 2016. The complete biodegradation pathway of ellagitannins by Aspergillus niger in solid‐state fermentation. J. Basic Microbiol. 56(4):329-336. doi: 10.1002/jobm.201500557 [ Links ]
Babu, B. K.; Saxena, A. K.; Srivastava, A. K. and Arora, D. K. 2007. Identification and detection of Macrophomina phaseolina by using species-specific oligonucleotide primers and probe. Mycologia. 99(6):797-803. http://dx.doi.org/10.1080/15572536.2007.11832511. [ Links ]
Basco, M. J.; Bisen, K.; Keswani, C. and Singh, H. B. 2017. Biological management of Fusarium wilt of tomato using biofortified vermicompost. Mycosphere. 8(3):467-483. http://dx.doi.org/10.5943/mycosphere/8/3/8. [ Links ]
Chandra, S.; Choure, K.; Dubey, R. C. and Maheshwari, D. K. 2007. Rhizosphere competent Mesorhizobiumloti MP6 induces root hair curling, inhibits Sclerotinia sclerotiorum and enhances growth of Indian mustard (Brassica campestris). Brazilian J. Microbiol. 38(2):124-130. http://dx.doi.org/10.1590/S1517-83822007000100026. [ Links ]
De-Asmundis, C.; Romero, C. H.; Acevedo, H. A.; Pellerano, R. G. y Vázquez, F. A. 2011. Funcionalización de una resina de intercambio ionico para la preconcentracion de HG (II). Avances en Ciencias e Ingeniería. 2(1):63-70. http://www.redalyc.org/articulo.oa?id= 323627681006. [ Links ]
Duarte, Y.; Pino, O. y Martínez, B. 2013. Efecto de cuatro aceites esenciales sobre Fusarium spp. Rev. Protec. Veget. 28(3):232-235. http://scielo.sld:cu/scielo.php?script=sci-arttext&pid= S=1010-27522013000300013. [ Links ]
Dwivedi, S. K. 2015. Eficacy of some medical plant extract against Fusarium oxysporum f. sp. ciceri causing chickpen wilt. Asian J. Crop Sci. 7(2):138-146. doi:10.3923/ajcs.2015. 138.146. [ Links ]
Gabrielson, J.; Hart, M.; Jarelöv, A.; Kühn, I.; McKenzie, D. and Möllby, R. 2002. Evaluation of redox indicators and the use of digital scanners and spectrophotometer for quantification of microbial growth in microplates. J. Microbiol. Methods. 50(1):63-73. https://doi.org/10.1016/S0167-7012(02)00011-8. [ Links ]
Gakuya, D. W.; Itoga, S. M.; Mbaria, J. M.; Muthee, J. K. and Musau, J. K. 2013. Ethnobotanical survey of biopesticides and other medicinal plants traditionally used in meru central district of Kenya. J. Ethnopharmacol. 145(2):547-553. Doi: 10.1016/j.jep.2012.11.028. [ Links ]
Jasso-Rodríguez, D.; García, R. R.; Castillo, H. F.; González, C. A.; Galindo, A. S.; Quintanilla, J. V. and Zuccolotto, L. M. 2011. In vitro antifungal activity of extracts of Mexican Chihuahuan desert plants against postharvest fruit fungi. Industrial Crops and Products. 34(1):960-966. https://doi.org/10.1016/j.indcrop.2011.03.001. [ Links ]
Jiménez, G. M. M.; Pérez, A. E. and Jiménez, D. R. M. 2001. Identification of pathogenic races 0, 1B/C, 5, and 6 of Fusarium oxysporum f. sp. ciceris with random amplified polymorphic DNA (RAPD). Eur. J. Plant Pathol. 107(2):237‐248. doi: 10.1023/a: 1011294204630. [ Links ]
Landa, B. B.; Navas, C. J. A.; Jiménez, G. M. M.; Katan, J.; Retig, B. and Jiménez D. R. M. 2006. Temperature response of chickpea cultivars to races of Fusarium oxysporum f. sp. ciceris, causal agent of Fusarium wilt. Plant Dis. 90(3):365‐374. http://dx.doi.org/10.1094/PD-90-0365. [ Links ]
Masoko, P.; Picard, J. and Eloff, J. N. 2005. Antifungal activities of six South African Terminalia species (Combretaceae). J. Ethnopharmacol . 99(2):301-308. http://dx.doi.org/10.1016/ j.jep.2005.01.061. [ Links ]
Matsuura, H. N. and Fett, N. A. G. 2017. Plant alkaloids: main features, toxicity, and mechanisms of action. Plant toxins Gopalakrishnakone, P.; Carlini, C. R. and Igabue, B. R. (Ed.). 243-261p. doi 10.1007/978-94-007-6728-7-2-1. [ Links ]
Morales, G. J. A. y Durón, N. L. J. 2004. Aspectos generales. Morales, G. J. A.; Durón, N. L. J.; Martínez, D. G.; Núñez, M. D. J. H. y Fu, C. A. A. (Ed.). El cultivo de garbanzo blanco en Sonora. Libro Técnico Núm. 6. INIFAP-Campo Experimental Costa de Hermosillo, Sonora, México. 11-24 pp. [ Links ]
Moreno, L. S.; González, S. L. N.; Salcedo, M. S. M.; Cárdenas, Á. M. L. y Perales, R. A. 2011. Efecto antifúngico de extractos de gobernadora (Larrea tridentata L.) sobre la inhibición in vitro de Aspergillus flavus y Penicillium sp. Polibotánica. 32:193-205. http://www.redalyc.org/articulo.oa?id=62119933012. [ Links ]
Navas, C. J. A.; Hau, B. and Jiménez‐Díaz, R. M. 1998. Effect of sowing date, host cultivar, and race of Fusarium oxysporum f. sp. ciceris on development of Fusarium wilt of chickpea. Phytopathology. 88(12):1338‐1346. [ Links ]
Paredes, E. J. E.; Carrillo, F. J. A.; García, E. R. S.; Allende, M. R.; Sañudo, B. J. A. y Valdez, T. J. B. 2009. Microorganismos antagonistas para el control del complejo de hongos causantes de la rabia del garbanzo (Cicer arietinum L.) en el Estado de Sinaloa, México., Rev. Mex. Fitopat. 27(1):27-35. [ Links ]
Sharma, K. D. and Muehlbauer, F. J. 2007. Fusarium wilt of chickpea: physiological specialization, genetics of resistence and resistence gene tagging. Euphytica. 157(1):1-14. doi: http://dx.doi.org/10.1007/s10681-007-9401-y. [ Links ]
Sparks, T. C.; Hahn, D. R. and Garizi, N.V. 2017. Natural products, their derivatives, mimics and synthetic equivalents: role in agrochemical discovery. Pest Manag. Sci. 73(4):700-715. Doi: 10.1002/ps.4458. [ Links ]
Sánchez, S. M.; Gambardella, J. L. and Enriquez, I. D. 2013. First report of crown rot of strawberry caused by Macrophomina phaseolina in Chile. Plant Dis. 97-996 p. http://dx.doi.org /10.1094/PDIS-12-12-1121-PDN. [ Links ]
SIAP. 2017. Sistema de Información Agroalimentaria y Pesquera. Avance de siembras y cosechas, resumen nacional por cultivo. https://www.gob.mx/siap/. [ Links ]
Toma, M.; Vinatoru, M.; Paniwnyk, L. and Mason, T. 2001. Investigation of the effects of ultrasound on vegetals tissues during solvent extraction. Ultrasonics Sonochemistry. 8(2):137-142. http://dx.doi.org/10.1016/S1350-4177 (00)00033-X. [ Links ]
Yossen, V. E. y Conles, M. Y. 2016. Eficacia de fungicidas in vitro para el control de Fusarium oxysporum y F. proliferatum, agentes causales de marchitamiento en el cultivo de orégano en la Argentina. Rev. Industrial y Agrícola de Tucumán. 91(1):19-25. https://riat.eeaoc. org.ar/ojs/ index.php /riat/ article/view/v91n1a03/33. [ Links ]
Zacarés, L. 2008. Nuevas aportaciones al metabolismo secundario del tomate, identificación y estudio de moléculas implicadas en la respuesta a la infección con Pseudomonas syringae pv. Tomato. Tesis Doctoral. Universidad Politécnica de Valencia. https://doi.org/10.4995/Thesis/10251/3021 . [ Links ]
Zaker, M. 2014. Antifungal evaluation of some plant extracts in controlling Fusarium solani, the causal agent of potato dry rot in vitro and in vivo. Inter. J. Agri. Biosci. 3(4):190-195. https://www.ijagbio.com/ [ Links ]
Zhang, J. Q.; Zhu, Z. D.; Duan, Z. D.; Wang, C. X. and Li, H. J. 2011. First report of charcoal rot caused by Macrophomina phaseolina on mungbean in china. Plant Dis. 95(7):95-87. http://dx.doi.org/10.1094/PDIS-01-11-0010. [ Links ]
Received: August 01, 2021; Accepted: October 01, 2021