Servicios Personalizados
Revista
Articulo
Indicadores
-
Citado por SciELO
-
Accesos
Links relacionados
-
Similares en SciELO
Compartir
Revista mexicana de ciencias agrícolas
versión impresa ISSN 2007-0934
Rev. Mex. Cienc. Agríc vol.12 no.5 Texcoco jun./ago. 2021 Epub 14-Mar-2022
https://doi.org/10.29312/remexca.v12i5.2764
Articles
Strawberry quality affected by the nitrate:ammonium ratio in the nutrient solution
1Crop Science Department-Federal University of Paraná, Brazil. R. dos Funcionários 1540, Curitiba, Brazil. ZC. 80035-050. (francine@ufpr.br).
5²Soils and Agricultural Engineering Department-Federal University of Paraná, Brazil. R. dos Funcionários 1540, Curitiba, Brazil. ZC. 80035-050. (vpauletti@ufpr.br).
3Basic Pathology Department-Federal University of Paraná, Brazil. Av. Cel. Francisco H. dos Santos 100, Curitiba, Brazil. ZC. 81531-980. (mazawa@ufpr.br).
Strawberry (Fragaria x ananassa Duch.) production by using the semi-hydroponic fertigation system, is an increasingly adopted technology. One of the main challenges of this system is the nutritional management of nitrate (NO3 -) and ammonium (NH4 +), which can affect fruits development and quality. This work aims to determine the NO3 -:NH4 + ratio of the nutrient solution for better quality of strawberry fruits grown in a semi-hydroponic system. ‘San Andreas’ strawberry runners were planted in an organic substrate composed by charred rice husk and pine bark. The NO3 -:NH4 + proportions evaluated in fertigation were 100:0; 75:25; 50:50; 25:75 and 0:100. Leaf nitrogen contents and aerial dry mass loss showed a proportional increase concomitantly NH4+ increase on the solution. Leaf area and chlorophyll content increased up to 29% NH4 + in the nutrient solution, while higher concentrations negatively affected these characteristics. Chemical fruits’ features were not affected by the NO3 -:NH4 + ratio, but the pulp firmness decreased as the NH4 + increased in the nutrient solution. Results obtained showed the best strawberry fruits development and quality were obtained by applying NO3 -:NH4 + in fertigation solution at the ratio of 71:29.
Keywords Fragaria x ananassa Duch; ammonium nutrition, ‘San Andreas’, soilless cultivation
La producción de fresa (Fragaria x ananassa Duch.) mediante el uso del sistema de fertirrigación semihidropónico es una tecnología cada vez más adoptada. Uno de los principales desafíos de este sistema es el manejo nutricional del nitrato (NO3 -) y el amonio (NH4 +), que pueden afectar el desarrollo y la calidad de los frutos. Esta investigación tuvo como objetivo determinar la mejor relación NO3 -:NH4 + para una mejor calidad de frutos de fresa cultivados en un sistema semihidropónico. Los corredores de fresa ‘San Andreas’ se plantaron en sustrato orgánico compuesto por cáscara de arroz carbonizada y corteza de pino. Las proporciones de NO3 -:NH4 + evaluadas en fertirrigación fueron 100: 0; 75:25; 50:50; 25:75 y 0:100. El contenido de nitrógeno de las hojas y la pérdida de masa seca aérea mostraron un aumento proporcional concomitantemente con un aumento de NH4 + en la solución. El área foliar y el contenido de clorofila aumentaron hasta 29% de NH4 + en la solución nutritiva, mientras que concentraciones más altas afectaron negativamente estas características. Las características químicas de las frutas no se vieron afectadas por la relación NO3 -:NH4 +, pero la firmeza de la pulpa disminuyó a medida que aumentaba el NH4 + en la solución nutritiva. Los resultados obtenidos mostraron que los frutos de fresa con mejor desarrollo y calidad se obtuvieron aplicando NO3 -:NH4 + en solución de fertirrigación en la proporción de 71:29.
Palabras clave Fragaria x ananassa Duch.; cultivo sin suelo; nutrición de amonio; ‘San Andreas’
Introduction
Strawberry cultivation in the semi-hydroponic system, popularly known as soilless cultivation, is a is a practice applied in several regions of the planet (Recamales et al., 2007; Neri et al., 2012; Rampazzo, 2016). Instead of soil, it uses a wide range of raw materials to produce the substrates, such as perlite, vermiculite, peat, pine bark, charred rice husk, and coconut fiber (Lieten et al., 2004; Jarosz and Konopinska, 2010; Marques, 2016). The substrats used in semi-hydroponic system usually have good physical and chemical characteristics and have good nutrient bioavailability for plant development (Abad et al., 2005; Ilha, 2013). They also, are free of pest and diseases, provide more rational water and nutrients use, and are more ergonomics for labors (Rampazzo, 2016).
One of the most critical points in strawberry semi-hydroponic system is the nitrogen management by fertigation (Othman et al., 2019). Nitrogen availability affects strawberries productivity and fruits quality (Nestby et al., 2004; Jarosz and Konopiska, 2010). Both nitrogen forms, nitrate and ammonium salts, are absorbed and metabolized by plants (Roosta et al., 2009), and play important metabolic functions, such as growth, development, production and affect fruits appearance, durability and flavor (Tabatabaei et al., 2008; Krüger et al., 2012; Samec et al., 2016).
Despite this, there is little information about nitrate and ammonium concentration in nutrient solutions for growing strawberries. In the existing ones, the variation is quite expressive, between 4 and 12 mmol L-1 for NO3 - and 0.2 and 2.5 mmol L-1 for NH4 + (Hennion and Veschambre, 1997; Paranjpe et al., 2003; Furlani and Fernandez, 2004). This research aimed to determine the best NO3 -:NH4 + ratio for strawberry production by using the semi-hydroponic fertigation system.
Material and methods
This trial was performed in Curitiba, Paraná State, Brazil (25° 24’ 38.5” S; 49° 14’57.5” W). Each ‘San Andreas’ strawberry runners, a neutral cultivar to the photoperiod, was planted in 8-liter black polypropylene pots on 09/07/18, filled with organic substrate (Table 1) composed by rice husk and pine bark.
Table 1 Characteristics of the organic substrate used for cultivation of ‘San Andreas’ strawberry runners in a semi-hydroponic system.
pH CaCl2 | DD (kg m-3) | EC (mS cm-1) | C (g dm-3) | TP | RAW | BS | AW | |
---|---|---|---|---|---|---|---|---|
(%) | ||||||||
5.65 | 266.78 | 399 | 151.3 | 84.4 | 7.96 | 1.59 | 9.55 |
DD= dry density; TP= total porosity; RAW= readily available water; BS= buffer solution; AW= available water
The experimental design applied was completely randomized with five repetitions per treatment, and each repetition with three pots. The treatments consisted of proportions of nitrate and ammonium (NO3 -:NH4 +) in the nutrient solution of 100:0; 75:25; 50:50; 25:75; and 0:100%, with 150 mg L-1 nitrogen concentration applied in all pots, based and modified by Furlani and Fernandes Júnior (2004) (Table 2).
Table 2 Macronutrients and chlorine concentration in the nutritional solution used, according to each nitrate: ammonium proportion.
NO3 -:NH4 + | NO3 - | NH4 + | SO4 2- | Cl- |
---|---|---|---|---|
(mg L-1) | ||||
100-0 | 150 | 0 | 48.9 | 131 |
75-25 | 112.5 | 37.5 | 90 | 249.8 |
50-50 | 75 | 75 | 112.5 | 423.1 |
25-75 | 37.5 | 112.5 | 153.6 | 544.8 |
0-100 | 0 | 150 | 194.6 | 666.1 |
Other nutrients were applied in the same concentrations in all pots: 170 mg L-1 of Ca, 200 mg L-1 of K, 40 mg L-1 of HPO4 -, 40 mg L-1 of Mg, 32 μmol L-1 of Fe, 10 μmol L-1 of Mn, 30 μmol L-1 of B, 3.1 μmol L-1 of Zn, 1.3 μmol L-1 of Cu, and 0.17 μmol L-1 of Mo. The following salts were used to formulate the nutrient solution: Ca(NO3)2, KNO3, KH2PO4, MgSO4, NH4H2PO4, CaCl2, KCl, (NH4)2SO4, H3BO3, CuSO45H2O, MnSO4H2O, ZnSO47H2O, Na2MoO42 H2O, Fe-EDDHA (6%).
Fertigation was applied in each pot once a day, to maintain the substrate water retention capacity at 60%, in such way approximately 500 mL were daily applied per pot. Electrical conductivity (EC) on the nutritional solution was maintained between 1 300 and 1 800 mS cm-1 and the pH of 6 ±0.2.
Leaf evaluations were performed at 60 and 120 days after planting (Dap), corresponding to the vegetative and productive stages, respectively (Nepar, 2019). The leaf area was obtained by measuring the length and width of the leaflets of the newly developed 4th leaf of the plant with a digital caliper and using the equation La= (π / 4) [(L + W) / 2]2 (Pires, 1999). Were La= leaf area, L= length and W= width. From the same leaflets, a tissue sample of approximately 0.17 g was collected to evaluate chlorophyll a, b and total leaves (Porra, 2002).
The material was macerated with 10 ml of 80% acetone and centrifuged at 12 000 rpm for 10 minutes and a spectrophotometer reading at 645 and 663 nm wavelengths was performed. The remainder of the 4th collected leaf was used for nutritional analysis (S, Ca, K, Mg, P, Fe and Zn) by wet digestion with nitric acid (HNO3) and hydrogen peroxide (H2O2), and reading were done with the inductively coupled plasma optical emission spectroscopy (ICP-OES) (Varian 720-ES™). Total N was analyzed by the Dumas combustion method in Elemental Analyzer (Carmo et al., 2000).
All senescent leaves were collected through the plants’ growth and dried at 65 ºC until reaching constant mass. At the end of trial, all remained leaves were also dried at 65 ºC until reaching constant mass. Dry weigh per plant were obtained by adding the senescence dry weight to the final leaves dry weight.
Physical and chemical fruits features were analyzed on ten fruits harvested per replicate, in three harvest dates (20/12/2017; 20/01/2018; 20/02/2018). Those with at least 3/4 reddish epidermis, minimum weight of 6 g and without injuries, diseases or deformations were chosen. First of all, pulp firmness was determined by using a texture analyzer (Brookfield CT3™), with 2 mm tip, 5 mm penetration and 5 mm s-1 penetration speed. Afterwards, strawberry juice was extracted, soluble solids content was measured on the by using a refractometer. Juice solution was diluted in water to 10% and titration was performed with 0.1 M sodium hydroxide solution (NaOH) under constant agitation, to reach the pH of 8.2.
The Bartlett test was used to verify the homogeneity of variances (p> 0.05) of the data, followed by Anova. In case of significant variation, regression analysis was used to verify the effects of the NO3 -:NH4 + ratios. For the statistical analysis, the software Assistat 7.7 (Silva, 2002) was used.
Results and discussion
The NO3 -:NH4 + proportions did not affect the most leaves’ nutrient content (S=1.19 g kg-1; Ca =10.64 g kg-1; K=18.13 g kg-1; Mg= 3.75 g kg-1; P= 2.89 g kg-1; Fe= 69.42 mg kg-1; Zn= 23.38 mg kg-1). These nutrients levels are the similar foliar level reported in strawberry leaves by other authors (CQFS RS/SC, 2004; Nepar, 2019). It was expected did affect the Ca, K and Mg levels under the highest NH4 + level in the nutritive solution, through competition between ions of the same valence, either by a protein channel or for binding to a transport protein in cell membrane (White, 2012), but it not happened in this research.
We also didn’t see a change in pH in the rhizosphere due to NH4 + absorption (Hawkesford et al., 2012), which has a direct effect on nutrient bioavailability (Waller and Wilson, 1984). The substrate pH changed very few during this trial (5.65 before planting- 5.27 in 0-100% of NO3 -:NH4 +), probably due to its organic composition. The substrate composition plays an important role since Choi et al. (2011) found a decrease in the substrate pH, from pH 7.0 with the use of nutrient solution without NH4 + to pH 5.8 with NH4 +, and also a decrease in the levels of K+, Ca2+ and Mg2+ in strawberry leaves using a mineral substrate.
Among all nutrients evaluated in the leaf, only the total nitrogen (N) was affected by the different proportions of NO3 -:NH4 + from the nutrient solutions. The content found was 20.64 g kg-1, when 100% of the nitrogen applied was in the form of NH4 +, representing an increase of approximately 14% of N in relation to the absence of NH4 + (Figure 1). A similar result was obtained by Tabatabaei et al. (2008), where the application of the maximum dose of 75% of NH4 + in the ‘Selva’ strawberry variety found an increase in the leaf content of N around 16.8%, and by Choi et al. (2011) with an increase of 14.2% of N when using 100% NH4 + in nutrient solution.

Figure 1 Content of total nitrogen in leaves of the ‘San Andreas’ strawberry cultivar according to NO3 -:NH4 + proportions in nutritive solution, in vegetative period (60 days after planting) and reproductive period (120 days after planting), in semi-hydroponic cultivation.
Most plants absorb the two nitrogen forms which are mediated by specific transport proteins (Roosta et al., 2009). Nitrogen forms can be metabolized in the same root cell where it was absorbed or translocated unchanged to the aerial part of the plant. NH4 + is assimilated into root cells and its reduced form is carried to the leaves, while most of NO3 - is carried to the leaves and then reduced to be assimilated to amino acids. The energy requirement for NH4 + assimilation is lower than for NO3 - assimilation, since the first does not need to be reduced for its incorporation into amino acids (Bloom et al., 1992; Hawkesford et al., 2012; Taiz and Zeiger, 2017). Possibly, the NH4 + assimilated in the roots and transported in the form of amino acids, ended up accumulating in the leaves and increasing the levels of N in these tissues (Majerowicz et al., 2000).
High concentrations of NH4 + in the nutrient solution caused necrosis in the leaves (Figure 2), consequently increasing the dry mass of the aerial part (Figure 3), indicating a toxic effect on the plant. Choi et al. (2011) also observed that in high concentrations of NH4 + in the nutrient solution, the young strawberry leaves had a matte green color, with withered and curled edges, while the older leaves were dry and tanned. The higher N content in the leaf (Figure 1) favored the production of NH4 + through photorespiration, considered the main metabolic pathway for the production of this compound in plants (Bittsánszky et al., 2015), leading to toxicity.
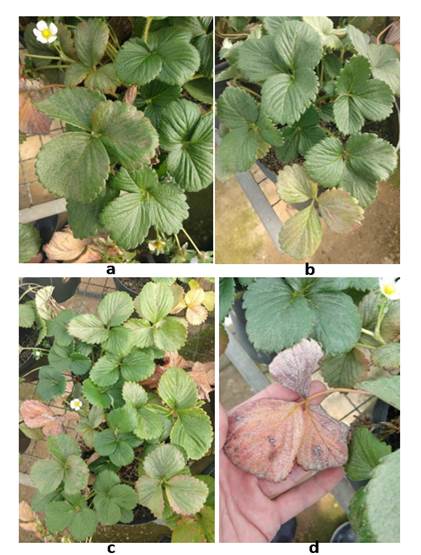
Figure 2 Symptoms related to NH4 + toxic effect in strawberry plants under 0:100% treatment of NO3 -:NH4 + in nutrient solution, where: a) symptom onset; b) aggravated symptom; c) aerial view of the plant; and d) leaf death.
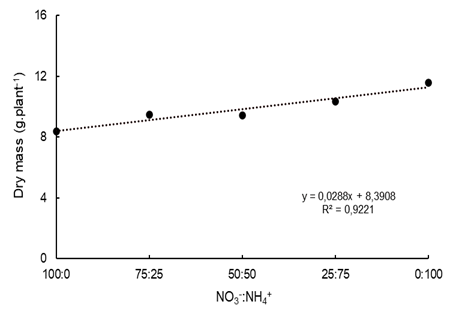
Figure 3 Dry mass of ‘San Andreas’ cultivar after the period of 120 days after planting the seedlings, according to NO3 -:NH4 + proportions in nutrient solution in semi-hydroponic cultivation.
Toxicity occurs when the rate of NH4 + assimilation into amino acids and amides becomes lower than the rate of absorption. In the leaves, the accumulation of NH4 + causes damage to the structures of the chloroplast, lowering the pH of the cells to intolerable levels. This fact dissipates transmembrane proton gradients necessary for electron transport in photosynthesis, which results in a decrease in the photosynthetic rate (Bittsánszky et al., 2015).
Chlorophyll levels varied similarly on 60 DAP and 120 DAP periods, under the proportions of NO3 -:NH4 + (Figure 4). The maximum chlorophyll contents were 0.25 mg g-1, in the proportions of 36.2% NH4 +. Tabatabaei et al. (2008) also observed chlorophyll content increase using the proportion of 50% NH4 + in nutrient solution. In our study, increasing NH4 + concentration above 36% decreased leaf chlorophyll content, probably due the NH4 + phytotoxicity discussed earlier. Maximum levels of chlorophyll were obtained with NO3 - and NH4 + in the fertigation solution, in which the proton generated by NH4 + assimilation can be used to reduce NO3 -, thus making it easier for plants to regulate intracellular pH when both forms of nitrogen are provided (Hawkesford et al., 2012).
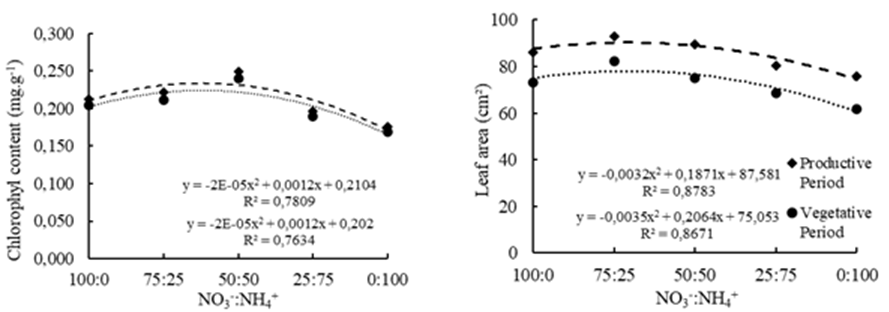
Figure 4 Chlorophyll content and leaf area on ‘San Andreas’ strawberry leaves according to the proportions of NO3 -:NH4 + in nutrient solution in vegetative period (60 days after planting) and reproductive period (120 days after planting) in semi-hydroponic cultivation.
The adequate combination of NO3 -:NH4 + has a stimulating effect on plants because reduce the spent of energy in nitrogen uptake process, done by root cells, and in assimilation into amino acids. Therefore, the cell with your metabolism regulated and with greater energy efficiency can assimilate the nitrogen into glutamine and glutamate, as well as incorporate other amino acids that play an important role in protein and molecule formation, such as chlorophyll (Taiz and Zeiger, 2017).
The ratio 71:29 to NO3 -:NH4 + was the one that provided the highest leaf area, both in 60 DAP and 120 DAP, reaching 93 cm² (Figure 4). These values approximate those obtained in the studies conducted by Tabatabaei et al. (2008) and Choi et al. (2011), in which the application of 25% of the nitrogen in the form of NH4 + resulted in larger leaf areas. A greater leaf expansion was possible, since the osmotic and energetic regulation provided by the two forms of N (NO3 - and NH4 +) benefited the cell elongation and plant growth (Cao and Li, 2003; Li et al., 2013).
Leaf area results (Figure 4) show the same tendency as chlorophyll contents (Figure 4), since chlorophyll is the basis for the formation of various components of the plant photosynthetic apparatus, providing greater leaf development. However, there was a lower leaf growth on plants supplemented with high NH4 + concentration (75% and 100%), probably due to higher demand of carbohydrates that are channeled for assimilation and detoxification of the large amount of this cation.
The pulp firmness was the only physical-chemical characteristic that guides the quality of the fruit affected by the proportions of NO3 -:NH4 + and decreased up to 25% with increasing NH4 + levels in the nutrient solution (Figure 5). Its similar to the results found by Sokri et al. (2015) in apples, which the highest concentration of NH4 + tested in the solution decreases the firmness of the pulp.
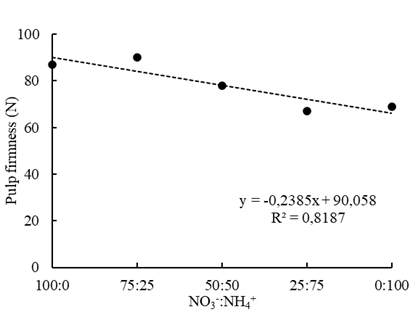
Figure 5 Pulp fruit firmness of ‘San Andreas’ strawberry cultivar according to NO3 -:NH4 + proportions in nutrient solution in semi-hydroponic cultivation.
The firmness of the pulp is closely linked to Ca since it is linked to polygalacturonic acids such as pectins. They form the Ca-pectates in the middle lamella, whose function is essential for the formation of cell walls and plant tissues. Pectate degradation is mediated by polygalacturonase, an enzyme strongly inhibited by high Ca concentrations (Wehr et al., 2004). Thus, when Ca deficiency occurs, polygalacturonase activity is increased (Konno et al., 1984). A typical symptom of Ca deficiency is the disintegration of cell walls and the breakdown of affected tissues, which causes loosening and loss of fruit firmness (Ho and White, 2005). The reduction in pulp firmness may be related to the lower Ca concentration in fruits (Alan, 1989). In this experiment, although leaf concentrations of Ca were not affected by NH4 +, it is likely that Ca concentrations in the fruit may have been influenced.
Fruits had mean values of total soluble solids (TSS) of 8.5, in °Brix, total titratable acidity (TTA) in % of citric acid of 0.97; TSS/TTA (ratio) of 8.9 and pH of 3.5. According to Kader (1999) for the strawberry flavor to be acceptable, the minimum amount of TSS recommended is around 7 °Brix. The maximum values of acidity recommended are between 0.8%, and the SS/TA ratio of at least 8.75. It was expected that SST levels would decrease and TTA levels would increase with higher concentrations of NH4 + in the nutrient solution.
This is probably because, according to Sokri et al. (2015) and Zhang et al. (2019), the application of NH4 + decreases the influx of K by the plant, due to the antagonism of these cations. Cation concentration is reduced with increasing ammonium concentration, which can lead to an increase in the acidity of the fruits. On the other hand, in soybean seeds and apple fruit (Malus domestica Borkh. cv. Fuji) fertilization with K increased TSS (Tu et al., 2017; Zhang et al., 2017). Although leaf tissues show symptoms of NH4 + toxicity, in this trial, leaf K contents did not vary with the increase in NH4 +, maintaining the chemical characteristics of the fruits.
Conclusions
In this work, the damage caused by the excess of NH4 + in the nutrient solution was mitigated by the use of an organic substrate in strawberry cultivation. Given the above, the exact mechanisms of the toxicity caused by NH4 + are not fully understandable. Future studies are recommended to better elucidate how NH4 + affected the firmness of strawberry pulp. The best NO3 -:NH4 + ratio for strawberry development and quality by using the semi-hydroponic fertigation system is 71:29.
Literatura citada
Abad, M.; Fornes, F.; Carrión, C.; Noguera, V.; Noguera, P.; Maquieira, A. and Puchades, R. 2005. Physical properties of various coconut coir dusts compared to peat. HortScience. 40(7):2138-2144. Doi: https://doi.org/10.21273/hortsci.40.7.2138. [ Links ]
Alan, R. 1989. The effect of nitrogen nutrition on growth, chemical composition and response of cucumber Cucumis sativius L. to nitrogen forms in solution culture. J. Hortic. Sci. 64(4):467-474. Doi: https://doi.org/10.1080/14620316.1989.11515979. [ Links ]
Bittsánszky, A.; Pilinszkya, K.; Gyulaib, G. and Komives, T. 2015. Overcoming ammonium toxicity. Plant Sci. 231(2):184-190. Doi: 10.1016/j.plantsci.2014.12.005. [ Links ]
Bloom, A. J.; Sukrapanna, S. S. and Warner, R. L. 1992. Root respiration associated with ammonium and nitrate absorption and assimilation by barley. Plant Physiol. 99(4):1294-1301. Doi: https://doi.org/10.1104/pp.99.4.1294. [ Links ]
Cao, C. L. and Li, S. X. 2003. Effects of N form on some physiological characteristics and yield of wheat during the vegetative reproductive growth stages. Acta Agr. Singapore. 29(2):258-262. [ Links ]
Carmo, C. A. F. S.; Araujo, W. S.; Bernardi, A. C. C. y Saldanha, M. F. C. 2000. Metodos de análise de tecidos vegetais utilizados na embrapa solos. Río de Janeiro. Embrapa Solos. 41 p. [ Links ]
CQFS, RS/ SC. 2004. Comissão de química e fertilidade do solo. Manual de adubação e de calagem para os estados do Rio Grande do Sul e de Santa Catarina. 10. (Ed.). Porto Alegre, Sociedade Brasileira De Ciência do Solo. 400 p. [ Links ]
Choi, J. M.; Latigui, A. and Lee, C. W. 2011. Growth and nutrient uptake responses of ‘seolhyang’ strawberry to various ratios of ammonium to nitrate nitrogen in nutrient solution culture using inert media. Afr. J. Biotechnol. 10(59):12567-12574. Doi:10.5897/ajb11.1104. [ Links ]
Furlani, P. R. and Fernandez, J. F. 2004. Cultivo hidropônico de morango em ambiente protegido. In: simpósio nacional do morango and encontro de pequenas frutas e frutas nativas do mercosul pelotas. Anais pelotas: corrêa antunez, le (Ed.). Embrapa. 102-115 p. [ Links ]
Hennion, B. and Veschambre, D. 1997. La fraise: maîtrise de la production. Paris. CTFIL. 299 p. [ Links ]
Ho, L. and White, P. J. A. 2005. Cellular hypothesis for the induction of blossom end rot in tomato fruit. Ann. Bot. 95(4):571-581. Doi: https://doi.org/10.1093/aob/mci065. [ Links ]
Ilha, L. H. 2013. Produção de morango semi-hidropônico. In: seminário brasileiro sobre pequenas frutas. Embrapa Uva e Vinho. 41 p. [ Links ]
Jarosz, Z. and Konopinska, J. 2010. Effect of substrate type and nitrogen fertilization upon yielding and chemical composition of “elsanta” strawberry cultivar grown in unheated foil tunnel. Acta Scientiarum Polonorum, Hortorum Cultus. 9(1):87-96. [ Links ]
Kader, A. A. 1999. Fruit maturity, ripening, and quality relationships. Acta Hortic. 485(1):203-208. Doi:10.17660/ACTAHORTIC.1999.485.27. [ Links ]
Konno, H.; Yamaya, T. M.; Yamasaki, Y. and Matsumoto, H. 1984. Pectic polysaccharide break-down of cell walls in cucumber roots grown with calcium starvation. Plant Physiol . 76(3):633-637. Doi: https://doi.org/10.1104/pp.76.3.633. [ Links ]
Krüger, E.; Josuttis, M.; Nestby, R.; Toldam-andersen, T. B.; Carlen, C. and Mezzetti, B. 2012. Influence of growing conditions at different latitudes of europe on strawberry growth performance, yield and quality. J. Berry Res. 2(3):143-157. Doi: 10.3233/JBR-2012-036. [ Links ]
Li, S. X.; Zhao-Hui, W. and Stewart, B. A. 2003. Responses of crop plants to ammonium and nitrate. N. Adv. Agron. 118(1):205-397. Doi: https://doi.org/10.1016/B978-0-12-405942-9.00005-0. [ Links ]
Lieten, P.; Longuesserreet, J.; Baruzzi, G. and Lopez-Medina, J. 2004. Recent situation of strawberry substrate culture in Europe. Acta Hortic. 649(1):193-196. Doi: 10.17660/ActaHortic.2004.649.35. [ Links ]
Marques, G. N. 2016. Sistemas abertos e fechados de cultivo. In.: Rampazzo, E. F. Shimizu, H. K.; Peil, R. M. N.; Fermino, M. H.; Furlani, P. R.; Vidal, H. R. y Zawadneak, M. A. C. and Eneida, M. D. Cultivo de morangueiro em substrato. Curitiba: SENAR-PR. 112 p. [ Links ]
Majerowicz, N.; Kerbauy, G. B.; Nievola, C. C. and Suzuki, R. M. 2000. Growth and nitrogen metabolism of Catasetum fimbriatum (Orchidaceae) grown with different nitrogen sources. Environ. Exp. Bot. 44(3):195-206. Doi: https://doi.org/10.1016/S0098-8472(00)00066-6. [ Links ]
Hawkesford, M.; Horst, W.; Kichey, T.; Lambers, H.; Schjoerring, J.; Moller, I. S. and White, P. 2012. Functions of macronutrients. In: Marschner, P. Marschner’s mineral nutrition of higher plants, third (Ed.). UK. Academic press, London. 135-151 p. [ Links ]
Neri, D.; Baruzzi, G.; Massetani, F. and Faedi, W. 2012. Strawberry production in forced and protected culture in Europe as a response to climate change. Canada. Can. J. Plant Sci. 92(6):1021-1036. Doi: 10.4141/cjps2011-276. [ Links ]
Nestby, R.; Lieten, F.; Pivot, D.; Raynal-Lacroix, C. and Tagliavini, M. 2004. Influence of mineral nutrients on strawberry fruit quality and their accumulation in plant organs: a review. Int. J. Fruit Sci. 5(1):141-158. Doi: https://doi.org/10.1300/J492v05n01-13. [ Links ]
Paranjpe A.; CantliffeI, D. J.; Lamb, E. M.; Stoffella, P. J. and Powell, C. 2003. Winter strawberry production in greenhouses using soilless substrates: an alternative to methyl bromide soil fumigation. Hortic. Sci. Proceedings Florida State. 116(1):98-105. [ Links ]
Nepar. 2019. Núcleo Estadual de sociedade brasileira de ciência do solo. Manual de adubação e calagem para o estado do paraná. Nepar. 2ª (Ed.). 289 p. [ Links ]
Othman, Y.; Bataineh, K.; Al-Ajlouni, M.; Alsmairat, N.; Ayad, J.; Shiyab, S.; Qarallah, B. and Hilaire, R. 2019. Soilless culture: management of growing substrate, water, nutrient, salinity, microorganism and product quality. Fresenius Environmental Bulletin. 28(4):3249-3260. [ Links ]
Pires, R. C. M.; Folegatti, M. V. and Passos, F. A. 1999. Estimativa da área foliar de morangueiro. Horticultura Brasileira. 17(2):86-90. [ Links ]
Porra, R. J. 2002. The chequered history of the development and use of simultaneous equations for the accurate determination of chlorophylls a and b. Photosynth. Res. 73(1-3):149-156. [ Links ]
Rampazzo, E. F. 2016. Instalações para cultivo de morangueiro fora do solo. In: Shimizu, H. K .; Marques, G. N.; Peil, R. M. N. ; Fermino, M. H. ; Furlani, P. R. ; Vidal, H. R. and Zawadneak, M. A. C . and Eneida, M. D . Cultivo de morangueiro em substrato. Curitiba: SENAR -PR. 112 p. [ Links ]
Recamales, Á. F.; López Medina, J. and Hernanz, D. 2007. Physicochemical characteristics and mineral content of strawberries grown in soil and soilless system. J. Food Quality. 30(5):837-853. Doi: 10.1111/j.1745-4557.2007.00154.x. [ Links ]
Roosta, H. R.; Sajjadinia, A.; Rahimi, A. and Schjoerring, J. K. 2009. Responses of cucumber plant to NH4 + and NO3 − nutrition: The relative addition rate technique vs cultivation at constant nitrogen concentration. Sci. Hortic. 121(4):397-403. Doi: 10.1016/j.scienta.2009.03.004. [ Links ]
Samec, D.; Maretić, M.; Lugarić, I.; Mešić, A.; Salopek-sondi, B. and Duralija, B. 2016. Assessment of the differences in the physical, chemical and phytochemical properties of four strawberry cultivars using principal component analysis. Food Chem. 194(1):828-834. Doi:10.1016/j.foodchem.2015.08.095. [ Links ]
Silva, F. A. S. and Azevedo, C. A. V. 2002. The Assistat Software Version 7.7 and its use in the analysis of experimental data. Afr. J. Agr. Res. 11(39):3733-3740. [ Links ]
Sokri, S. M.; Babalar, M.; Barker, A. V.; Lesani, H. and Asgari, M. A. 2015. Fruit quality and nitrogen, potassium, and calcium content of apple as influenced by nitrate: Ammonium ratios in tree nutrition. J. Plant Nutr. 38(10):1619-1627. [ Links ]
Tabatabaei, S. J.; Yusefi, M. and Hajiloo, J. 2008. Effects of shading and NO3: NH4 ratio on the yield, quality and N metabolism in strawberry. Sci. Hortic . 116(3):264-272. Doi: 10.1016/j.scienta.2007.12.008. [ Links ]
Taiz, L.; Zeiger, E.; Møller, I. M. y Murphy, A. 2017. Fisiologia vegetal e desenvolvimento vegetal. 6 (Ed.). Porto Alegre. 719 p. [ Links ]
Tu, B.; Liu, C.; Tian, B.; Zhang, Q.; Liu, X. and Herbert, S. J. 2017. Reduced abscisic acid content is responsible for enhanced sucrose accumulation by potassium nutrition in vegetable soybean seeds. J. Plant Res. 130(3):551-558. DOI: 10.1007/s10265-017-0912-x. [ Links ]
Waller, P. L. and Wilson, G. C. S. 1984. Evaluation of growing media for consumer use. Acta Hortic . 150(1):51-58. Doi: 10.17660/ActaHortic.1984.150.5. [ Links ]
Wehr, J. B.; Menzies, N. W. and Blamey, F. P. C. 2004. Inhibition of cell-wall autolysis and pectin degradation by cations. Plant Physiol . Biochem. 42(6):485-492. Doi: https://doi.org/ 10.1016/j.plaphy.2004.05.006. [ Links ]
White, P. J. 2012. Ion uptake mechanisms of individual cells and roots: short-distance transport. In: marschner, P. Marschner’s mineral nutrition of higher plants. Third (Ed.). 672 p. [ Links ]
Zhang, W.; Zhang, N. S.; Zhao, J. J.; Guo, Y. P.; Zhao, Z. Y. and Mei, L. X. 2017. Potassium fertilization improves apple fruit (Malus domestica borkh. Cv. Fuji) development by regulating trehalose metabolism. J. Hortic. Sci . Biotechnol. 92(5):1-11. Doi: https://doi.org/10.1080/14620316.2017.1304165. [ Links ]
Zhang, J.; Lv, J.; Dawuda, M. M.; Xie, J.; Yu, J.; LI, J.; Zhang, X.; Tang, C.; Wang, C. and Gan, Y. 2019. Appropriate ammonium-nitrate ratio improves nutrient accumulation and fruit quality in pepper (Capsicum annuum L.). Agronomy. 9(11):683. Doi: https://doi.org/ 10.3390/agronomy9110683. [ Links ]
Received: March 2021; Accepted: June 2021