Servicios Personalizados
Revista
Articulo
Indicadores
-
Citado por SciELO
-
Accesos
Links relacionados
-
Similares en SciELO
Compartir
Revista mexicana de ciencias agrícolas
versión impresa ISSN 2007-0934
Rev. Mex. Cienc. Agríc vol.8 no.6 Texcoco ago./sep. 2017
Articles
Phaseolus vulgaris response to salinity generated by NaCl, Na2SO4 and NaHCO3
1Unidad Académica de Agricultura-Universidad Autónoma de Nayarit. Carretera Tepic-Compostela km 9, Xalisco, Nayarit, México. CP. 63780. Tel. 01 (311) 2110128.
2Programa de Hidrociencias-Colegio de Postgraduados, Estado de México, México.
3Instituto de Ecología-Universidad del Mar (UMAR). Puerto Ángel, Oaxaca, México.
4Departamento de Producción Agrícola-Centro Universitario de la Costa Sur (CU Costa Sur), Universidad de Guadalajara (U de G). Autlán de Navarro, Jalisco, México.
It was evaluated in the germination and seedling stages the response to salinity induced with NaCl, Na2SO4 and NaHCO3 of three bean varieties which are the most produced in Nayarit, México. In a greenhouse, a completely random experimental design A×B×C was established with 63 treatments and five replicates. Factor A has seven levels of salt concentration (of 0.000-5760 g L-1), B with three bean varieties (Black, Pinto, Azufrado) and C three types of salt (NaCl, Na2SO4, NaHCO3). Germination, length, fresh and dry biomass of the stem and root were measured. For all types and concentrations of salt, compared to the threshold, germination significantly decreased on average 31% to 4.032 g L-1 and 49% to 5.760 g L-1, where Azufrado bean was the most resistant and NaHCO3 salt the one that decreased the most the percentage of germination. Stem length was significantly decreased on average 3.942 cm to 5.76 g L-1, Pinto bean obtained increased fresh and dry shoot biomass and at a concentration of 2.304 g L-1 of NaHCO3 none of the varieties survived; root length significantly decreased 10.532 cm to 5760 g L-1, Pinto beans produced more fresh and dry root biomass and NaHCO3 was the more affecting salt (Tukey, p≤ 0.05). As the salt concentration increased, the germination percentage, length, fresh and dry biomass of the stem and root decreased; the damage caused by salts was: NaHCO3>NaCl> Na2SO4.
Keywords: biomass; germination; seedling
Se evaluó en la etapa de germinación y plántula la respuesta a la salinidad inducida con NaCl, Na2SO4 y NaHCO3 de tres variedades de frijol que son las de mayor producción en Nayarit, México. En invernadero, se estableció un diseño experimental A×B×C completamente al azar con 63 tratamientos y cinco repeticiones. El factor A tuvo siete niveles de concentración de sal (de 0.000 a 5.760 g L-1), el B tres variedades de frijol (Negro, Pinto, Azufrado) y el C tres tipos de sal (NaCl, Na2SO4 , NaHCO3). Se midió la germinación, longitud, biomasa fresca y seca del vástago y raíz. Para todas las concentraciones y tipos de sal, con respecto al umbral, la germinación disminuyó significativamente en promedio 31% a 4.032 g L-1 y 49% a 5.760 g L-1, donde el frijol Azufrado fue el más resistente y la sal NaHCO3 la que más disminuyó el porcentaje de germinación. La longitud de vástago disminuyó significativamente en promedio 3.942 cm a 5.76 g L-1, el frijol Pinto obtuvo mayor biomasa fresca y seca del vástago, y a una concentración de 2.304 g L-1 de NaHCO3 ninguna variedad sobrevivió; la longitud de raíz disminuyó significativamente 10.532 cm a 5.760 g L-1, el frijol Pinto produjo mayor biomasa fresca y seca de raíz y el NaHCO3 fue la sal que más afectó (Tukey, p≤ 0.05). Conforme se incrementó la concentración de sales el porcentaje de germinación, longitud, biomasa fresca y seca del vástago y la raíz disminuyeron; el daño de las sales fue NaHCO3>NaCl>Na2SO4.
Palabras clave: biomasa; germinación; plántula
Introduction
Soils have different concentrations and types of salt, derived from the processes of weathering and deposit by surface currents towards the lower parts, as well as anthropogenic activities in which the inadequate management of irrigation wáter stands out (Kovda, 1961; Ayers y Westcot, 1987). The most important salts present in soil and water are the salts of carbonic acid, sulfuric acid and hydrochloric acid (Kovda et al., 1973), the most common in agricultural areas soils are sodium chloride (NaCl), sodium sulfate (Na2SO4) and to a lesser extent sodium bicarbonate (NaHCO3) (Richards, 1990; Reginato et al., 2014).
The NaCl and Na2SO4 salts due to its high solubility remain in the soil solution in conditions of residual moisture (Sánchez-Bernal et al., 2012). Regarding NaHCO3, this is too toxic to crops, however, most will form CaCO3 and CaHCO3 precipitates (Sánchez-Bernal et al., 2008; Sánchez-Bernal et al., 2012). Therefore, this salt will affect bean varieties the most. Therefore, NaHCO3 was studied with particular attention, since much of the agricultural areas in México are irrigated with surface water of residual origin. The residual water is rich in NaHCO3 (Velázquez-Machuca et al., 2002).
México ranks fifth in production of beans (Phaseolus vulgaris L.) worldwide (Reyes-Rivas et al., 2008). Beans production in México occur in the spring-summer and autumn-winter agricultural cycles. The autumn-winter cycle is performed under conditions of residual moisture, conditions that increase salinity (Miyamoto and Pingitore, 1992).
Beans are sensitive to salt conditions and yield decreases from an electrical conductivity of 2 dS m-1 (Subbarao and Johansen, 1994). Mass and Hoffman (1977) showed that an electrical conductivity of up to 1 dS m-1 gives 100% grain yield while 6.3 dS m-1 decreases it to 0%.
Strogonov (1964) and Mass (1990), mentioned as the most common effect of salinity, the general retardation of plant growth; when the salts concentration increases above the limit level, the speed of growth and the size of most species decrease progressively. High salt concentrations affect the stage and germination rate, stem length, branching and leaf size (Gutiérrez-Rodríguez et al., 2005; Gama et al., 2007). The involvement degree is different among varieties and type of salt (Rogers et al., 1998; Sánchez-Bernal et al., 2008).
In agricultural soils with salinity problems it is necessary to know the tolerance of the crops to the concentrations and types of salt in the solution of the soil. Based on these data the producer can make an adequate management of the soil and the water according to the tolerance limits of the culture to the salt and with this achieve a more profitable production. Whereby the objective was to evaluate the response to salinity induced with NaCl, Na2SO4 and NaHCO3 in Black, Pinto and Azufrado common bean varieties in the germination and seedling stage.
Material and methods
Black Jamapa, Pinto and Azufrado beans were used, they were harvested in the irrigation district number 043 located in the coastal zone of Nayarit, México. The experiment was established in a greenhouse at the Unidad Académica de Agricultura de la Universidad Autónoma de Nayarit, located between 21° 25’ 40.88” north latitude and 104° 53’ 29.54” west longitude, at an average monthly temperature of 28 °C at a height of 974 masl.
In 500 mL black pots with agrolite as substrate, 10 bean seeds were planted at one centimeter depth. The seeds were pre-sterilized with 5% sodium hypochlorite solution and rinsed with distilled water. Presowing irrigation was applied to water retention capacity of the substrate with distilled water as control (T0= 0 g L-1) and saline solutions (T1= 0.64; T2= 1.472; T3= 2.304; T4= 3.2; T5= 4.032 and T6= 5.76 g L-1). Once the solution was poured, the pots were weighed daily to know the evapotranspirated water. The evapotranspiration volume was replenished with distilled water to maintain the salt concentration constant over time. After germinating, five seedlings were left per pot, continuing the irrigation with distilled water.
Treatments
The concentration of the salt solutions of NaCl, Na2SO4 and NaHCO3 was calculated based on the theoretical electrical conductivities (CE) and decreasing yield of 0% to 150%, the equation used was proposed by Mass and Hoffman ( 1977): Y= 100 - b (CEs - Su): where: Y= is the potential yield (%); CEs= is the electrical conductivity of the solution (dS m-1); Su= is the threshold salinity (dS m-1); b = is the decrease in yield per unit increase of salinity.
The treatments investigated were control 0% (T0), 0% threshold (T1), 25% (T2), 50% (T3), 75% (T4), 100% (T5) and 150% (T6) theoretical CE were: T0= 0 dS m-1; T1= 1 dS m-1; T2= 2.3 dS m-1; T3= 3.6 dS m-1; T4= 5 dS m-1; T5= 6.3 dS m-1 and T6= 9 dS m-1.
Preparation of solutions
The amount of salt in the treatments was calculated by applying the equations proposed by the Salinity Laboratory of the United States of America (Richards, 1990).
Where: mg L-1= is the salts concentration in the solution; CE= is the electrical conductivity of the solution (dS m-1 at 25 °C).
For calculation purposes it was considered that 0.64 g L-1 salt generates a theoretical electrical conductivity of 1 dS m-1.
Experimental design
A factorial design A×B×C was used, completely random with five replicates. Where A= salt concentration, B = bean variety and C = salt types. The first factor had seven levels (T0= 0 g L-1; T1= 0.64 g; T2= 1.472 g; T3= 2.304 g; T4= 3.2 g; T5= 4.032 g and T6= 5.76 g), the second had three (Black, Pinto and Azufrado bean) and the third had three (NaCl, Na2SO4 and NaHCO3). As experimental unit it was considered a pot with 10 seeds germinated for the first stage and five seedlings for the second.
Studied variables
In the germination stage it was evaluated with the appearance of the hypocotyl with the cotyledons on the agrolite and in the seedling stage when there was effect of the salts concentration in the first trifoliate leaf. In order to obtain the germination percentage the germinated seeds were quantified compared to the total. In the seedling stage, at the end of the experiment, at 22 days, the length in cm of the seedling and the root were measured. Fresh and dry biomass of the stem and root were evaluated with fresh and dry weight in g, using an analytical balance and stove at 60 °C.
Statistic analysis
Analysis of variance was applied using the SAS program (SAS Institute, 1999). Differences between means were compared by Tukey (p≤ 0.05) and regression analysis were performed.
Results and discussion
Germination
In the interaction bean factor by salt concentration it was found that at a concentration of 2.304 g the Black beans decreased in higher value its germination. In the salt type interaction by bean type Azufrado and Pinto beans obtained the greatest germination with Na2SO4, but with NaHCO3 Azufrado and Pinto decreased to near-Black bean values.
Generally the germination percentage decreased as salt concentration increased (Table 1), the theoretical CE of 3.6 dS m-1 with 2.304 g of the salt decreased 7%, the concentration of 4.032 g 31%and with 5.76 g 49% compared to the threshold, for all concentrations and types of salt. The most resistant bean variety in the germination stage at all concentrations and salt types was Azufrado, followed by Pinto and Negro (Table 2). The type of salt affected particularly each variety, the NaCl affected more the Pinto bean, the Na2SO4 affected Black beans and NaHCO3 the Pinto beans (Figure 1). The type of salt, at all concentrations, that decreased the most the percentage of germination of all beans varieties was NaHCO3 (33%), followed by NaCl (18%) and Na2SO4 (11%) (Table 3).
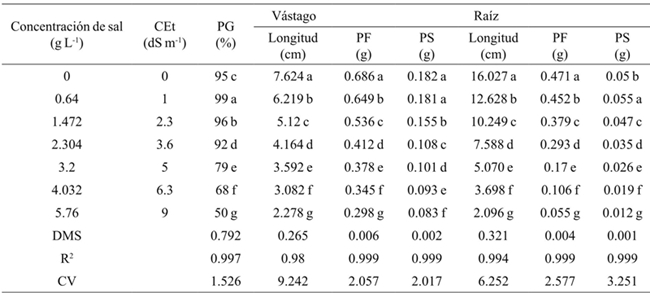
Promedios en una columna con la misma letra son estadísticamente iguales (Tukey, 0.05). CEt= conductividad eléctrica teórica; PG= porcentaje de germinación; PF= peso fresco; PS= peso seco; DMS= diferencia mínima significativa; CV= coeficiente de variación. Anova general, sin discriminar por tipo de sal ni variedad
Table 1 Effect of salt concentrations on germination, length, fresh and dry biomass of stem and root in beans.

Promedios en una columna con la misma letra son estadísticamente iguales (Tukey, 0.05). PG= Porcentaje de germinación; PF= peso fresco; PS= peso seco; DMS= diferencia mínima significativa; CV= coeficiente de variación. Los valores corresponden a la respuesta de cada variedad al factor salinidad sin discriminar concentración y tipo de sal.
Table 2 Effect of varieties on germination, length, fresh and dry biomass of stem and root in beans.

Figure 1 Germination of bean varieties under induced saline conditions with NaCl, Na2SO4 and NaHCO3.
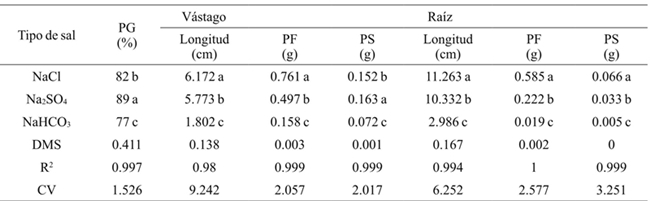
Promedios en una columna con la misma letra son estadísticamente iguales (Tukey, 0.05). PG= porcentaje de germinación; PF= peso fresco; PS= peso seco; DMS= diferencia mínima significativa; CV= coeficiente de variación. Los valores corresponden a medias generales para todas las variedades y todas las concentraciones usadas para cada tipo de sal.
Table 3 Effect of salt types on germination, length, fresh and dry biomass of stem and root in beans.
Aerial part
In the interaction bean factor by salt concentration it was found that the length, fresh and dry biomass of the stem of the concentration of 0.64 to 2.304, the Azufrado bean was the most affected with lower value for these variables. In the type of salt by bean type interaction, length, fresh and dry stem biomass, Azufrado showed lower values with NaCl and Na2SO4 but with NaHCO3 the three varieties drastically decreased the values of these variables and were close to each other.
Table 1 shows that as the salt concentration increased, the length and fresh and dry biomass of the stem decreased. At a concentration of 4.032 g L-1 stem length decreased 3.138 cm on average and 5.760 g 3.942 cm, with respect to the threshold, for all concentrations and types of salt. The variety that reached the highest fresh and dry biomass for all concentrations and salt types was Pinto beans (Table 2). In the seedling stage the salt that most affected the length, fresh and dry biomass of stem and root of bean varieties was NaHCO3 (Table 3), at a concentration of 2.304 g of NaHCO3 any variety survived (Figure 2).
Underground part
In the interaction bean factor by salt concentration it was found that the length, fresh and dry biomass of root of the concentration 0.64 to 2.304 the Azufrado bean was the most affected with lower value for these variables. In the salt type by bean type interaction, length, fresh and dry root biomass of Azufrado bean showed lower values with NaCl and Na2SO4 but with NaHCO3 the three varieties drastically decreased those values and were close to each other.
The length, fresh and dry biomass of the root decreased as the salinity increased. The root length in relation to the threshold had a difference in average of 8.93 cm at a concentration of 4.032 g L-1 and 10.532 at 5.76 g (Table 1). Pinto beans obtained higher fresh and dry biomass for all concentrations and salt types, followed by Azufrado and Black (Table 2). Figure 3 shows that NaHCO3 at a concentration of 2.304 g did not allowed root development. NaCl affects less the length of the root than Na2SO4.
Discussion
Germination
Mass and Hofman (1977) set, based on the percentage of relative yield, as bean tolerance limit (threshold) in terms of electrical conductivity 1 dS m-1 (0.64 g L-1), the data from this research are consistent, however the control (distilled water) had a germination 4% lower than that of the treatment with 0.64 g of salt. Germination in saline conditions where no distilled water is used, greenhouse conditions and inert substrate do not reflect this difference. On this result it is inferred that the concentration of ions in the solutions, up to a certain upper and lower limit, plays an important role in the activation of seed metabolism and function as scarifiers to enhance germination.
Tolerance to salinity in the germination stage is important because it determines rapid implantation of the crop and competitive advantage in the growth and production. The tolerance to salinity between bean varieties was different and in general the increase in salinity decreased the percentage of germination after the tolerance limit. Research in saline germination demonstrate this trend, Yokoishi and Tanimoto (1994) with Suaeda japónica Makino and Chenopodium quinoa Willd Madueño et al. (2006) with Rhynchosia minima L., Konak et al. (1999) and Bagci et al. (2007) with varieties and genotypes of Triticum aestivum L.
To germinate the seed requires absorbing enough water to activate its metabolism. The reduction in the germination percentage occurs by osmotic stress generated by the salts and the toxicity of specific ions. A lower osmotic potential in the germination medium due to the presence of high concentrations of salts promotes this osmotic stress. The higher the salt concentration the lower the osmotic potential, the water potential will decrease and the water will be less available for imbibition of the seed.
Osmotic stress with certain salts like NaHCO3 causes toxicity, so that lower germination is even greater. Our germination results with NaCl and Na2SO4 match those of Huang and Redmann (1995) whom in species of barley and canola, found less affection by sulfate salts compared to NaCl.
Aerial part
If the salt concentration of the growth medium exceeds the tolerance of the plant, the height, fresh and dry stem biomass and the water content in the plant would decrease (Cachorro et al., 1993; Hosseini et al., 2002; Bai et al., 2008; Keshavarzi, 2012; Habtamu et al., 2014). Some plants in order to tolerate high salinity levels in the growth medium perform an osmotic adjustment, which allows them to generate potentials smaller than the medium and to be able to absorb water and nutrients. Osmotic adjustment allows to maintain or increase cell turgor and plant growth (Bai et al., 2008; Bahena-Betancourt et al., 2008; Pratap and Sharma, 2010).
The seedlings in order to decrease its osmotic potential absorb the ions in solution and accumulate them in the tissue, thus counteracting the toxic potential generated by the salts. Some absorbed ions have toxic effects when they exceed the specific tolerance limits of each plant. Na+, Cl- and SO42-contribute to osmotic adjustment (Ottow et al., 2005). Bhivare and Nimbalkar (1984) found that NaCl and Na2SO4 salts led to the succulence of leaves, however NaCl reduces the height and dry matter of the plant, and improves cationic balance compared with Na2SO4. Cachorro et al. (1993) concluded that Phaseolus vulgaris is sensitive to NaCl salinity and growth inhibition was due to toxic effects of CI- ion. Bicarbonate at low concentrations had a very toxic effect in Picea glauca where aereal biomass diminished with sodium combined with carbonate at low concentrations (0.5 dS m-1) (Maynard et al., 1997).
The greatest effect of NaHCO3 is attributable to the osmotic effect and the generation of an alkaline pH that affects the enzymes activity and metabolic processes in cells (Yildirim et al., 2006; Pratap y Sharma, 2010).
In this paper, at the end of germination it was observed that the hypocotyl and radicle at 2.304 g of NaHCO3 were heavily damaged, those that survived concentrations below 2.304 g of NaHCO3 had a reduction in the stem and root length in the seedling stage. The decrease of fresh and dry biomass of the bean stem is attributed to the saline stress that could cause a reduction in the water absorption.
The plant in order to perform the osmotic adjustment under salinity conditions activates adaptation mechanisms to generate organic compounds. In addition to the accumulation of Na+ and Cl- in the tissues, the plant also produces proline in response to osmotic stress (Misra and Gupta, 2005; Slama et al, 2007; Bai et al., 2008). Bahena-Betancourt et al. (2008) mention that total carbohydrates are also generated in leaves and stems, in order to maintain the turgor in the early stages of the saline stress.
Underground part
Salinity caused by NaCl reduced the length, fresh and dry biomass of the root, which was more noticeable as the salinity of the medium increased and it was different among varieties. It was observed that from 2.3 g is Na2SO4 more inhibitory than NaCl regarding the roots length. This trend was observed in forages species (Shen et al., 2003), Glycine species (Kao et al., 2006), in Amaranthus spp. (Omamt et al., 2006), in Phaseolus vulgaris L. (Gama et al., 2007; Bahena-Betancourt et al., 2008; Radi et al., 2013; Habtamu et al., 2014) and Medicago sativa L. (Farissi et al., 2013).
Studies on germination stage of Lactuca sativa L. found that the length of the plumule and radicle decreased when increasing the salinity level (Keshavarzi, 2012). In this research, it was found that from 1.472 g of NaHCO3 the embryo development stopped and at higher levels they died. Reginato et al. (2014) in Prosopis strombulifera with NaCl and Na2SO4 found that SO42- had a deleterious effect on root growth than Cl-. In Figure 3 is shown a drastic inhibitory effect of root growth with NaHCO3 at concentrations of 2.034 g L-1.
Bai et al. (2008) found that the water deficit of the shoots was greater than that of the roots and had a positive correlation with the salt concentration. Under salt stress water transport was inhibited from the root to the seedling and this kept the Na+ in the roots. Hirpara et al. (2005) with Butea monosperma Taub and NaCl found that root growth was much greater than the stem, and that the water content of all tissues of primary roots and lateral roots was reduced, resulting in lack of internal water and inhibition of succulence. In this research the fresh biomass of the root with respect to the control was smaller and it is attributed to the water stress that could cause a decrease of the water content in the root.
Conclusions
The germination percentage, length and biomass of the stem and the bean root, was reduced with the increase of the concentration of the three salt types.
On germination the Azufrado bean was less affected by the salts. NaHCO3 was the one that most decreased the germination percentage of all beans varieties.
In seedlings, the Azufrado bean was the most affected and the Negro obtained the largest stem length. At 2.304 g L-1 (3.6 dS m-1 theoretical) of NaHCO3 any variety survived.
Salts damage was as follows: NaHCO3>NaCl> Na2SO4.
Literatura citada
Ayers, R. S. y Westcot, D. W. 1987. La calidad del agua y su uso en la agricultura. Estudio FAO Riego y Drenaje 29. Rev. 1. FAO,Roma, Italia, 81 p. [ Links ]
Bagci, S. A.; Ekiz, H. and Yilmaz. A. 2007. Salt tolerance of sixteenwheat genotypes during seedling growth. Turk J Agric For 31: 363-372. [ Links ]
Bahena, B. L.; Macías, R. L.; López, G. R. and Bayuelo, J. J. S. 2008. Crecimiento y respuestas fisiológicas de Phaseolus spp. En condiciones de salinidad. Rev. Fitotec. Mex. 31:213-223. [ Links ]
Bai, W. B.; Li, P. F.; Li, B. G.; Fujiyama, H. and Fan, F. C. 2008.Some physiological responses of Chinese iris to salt stress. Pedosphere. 18:454-463. [ Links ]
Bhivare, V. B. and Nimbalkar, J. D. 1984. Salt stress effects on growth and mineral nutrition of French beans. Plant and Soil. 80:91- 98. [ Links ]
Cachorro, P.; Ortiz, A. and Cerdá, A. 1993. Growth, water relations and solute composition of Phaseolus vulgaris L. under saline conditions. Plant Sci. 95:23-29. [ Links ]
Farissi, M.; Ghoulam, C. and Bouizgaren, A. 2013. Changes in wáter deficit saturation and photosynthetic pigments of alfalfa populations under salinity and assessment of proline role in salt tolerance. Agric. Sci. Res. J. 3:29-35. [ Links ]
Gama, P. B. S.; Inanaga, S.; Tanaka, K. and Nakazawa, R. 2007.Physiological response of common bean (Phaseolus vulgaris L.) seedlings to salinity stress. Afr. J. Biotechnol. 6:79-88. [ Links ]
Gutiérrez, R. M.; Escalante, E. J. A. and Rodríguez, G. M. T. 2005. Canopy reflectance, stomatal conductance, and yield of Phaseolus vulgaris L. and Phaseolus coccinues L. under saline field conditions. Inter. J. Agric. Biol. 7:491-494. [ Links ]
Habtamu, A.; Ermias, E.; Muluken, E.; Mulugeta, T. and Shitaye, N.2014. Seed germination and early seedling growth of haricot bean (Phaseolus vulgaris L.) cultivars as influenced by salinity stress. Inter. J. Agric. Sci. 4:125-130. [ Links ]
Hirpara, K. D.; Ramoliya, P. J.; Patel, A. D. and Pandey, A. N 2005. Effect of salinisation of soil on growth and macro- and micro-nutrient accumulation in seedlings of Butea monosperma (Fabaceae). Anales de Biología. 27:3-14. [ Links ]
Hosseini, M. K.; Powell, A. A. and Bingham, I. J. 2002. Comparison of the seed germination and early seedling growth of soybean in saline conditions. Seed Sci. Res. 12:165-172. [ Links ]
Huang, H. and Redmann, R. E. 1995. Salt tolerance of Hordeum and Brassica species during germination and early seedling growth.Can. J. Plant Sci. 75:815-819. [ Links ]
Kao, W. Y.; Tsai, T. T.; Tsai H. C. and Shih, C. N. 2006. Response of three Glycine species to salt stress. Environ. Exp. Bot. 56:120-125. [ Links ]
Keshavarzi, M. H. B. 2012. Studying the effects of different levels of salinity which caused by NaCl on early growth and germination of Lactuca Sativa L. seedling. J. Stress Physiol. Biochem. 8:203-208. [ Links ]
Konak, C.; Yilmaz R. and Arabaci, O. 1999. Ege bölgesi bugdaylarinda tuza tolerans. Tr. J. Agric. Forestry. 23:1223-1229. [ Links ]
Kovda, V. A. 1961. Principles of the theory and practice of reclamation and utilization of saline soils in the arid zones. In: UNESCO (Ed). Salinity problems in the arid zones: proceedings of the Teheran Symposium. Paris, Francia. 201-213 pp. [ Links ]
Kovda, V. A.; Van, D. B. C. and Hagan. R. M. 1973. Irrigation, drainage and salinity. An international source book. Hutchinson/FAO/UNESCO. London, UK. 510 p. [ Links ]
Maas, E. V. and Hoffman, G. J. 1977. Crop salt tolerance-current assessment. Journal Irrigation and Drainage Division 103:115-134. [ Links ]
Maas, E. V. 1990. Crop salt tolerance. In: Tanji, K. K. (Ed.). Agricultural salinity assessment and management. N. Y., USA. 262-304 pp. [ Links ]
Madueño, M. A.; García, P. D.; Martínez, H. J. and Rubio, T. C. 2006. Germinación y crecimiento de frijolillo Rhynchosia minima(L.) DC con diferentes potenciales osmóticos. Terra Latinoam. 24:47-54. [ Links ]
Maynard, D. G.; Mallett, K. I. and Myrholm, C. L. 1997. Sodium carbonate inhibits emergence and growth of greenhouse-grown White spruce. Can. J. Soil Sci 77:99-105. [ Links ]
Misra, N. and Gupta, A. K. 2005. Effect of salt stress on proline metabolism in two high yielding genotypes of green gram.Plant Sci. 169:331-339. [ Links ]
Miyamoto, S. and Pingitore, N. E. 1992. Predicting calcium and magnesium precipitation in saline solutions following evaporation. Soil Sci. Soc. Am. J. 56:1767-1775. [ Links ]
Omamt, E. N.; Hammes, P. S. and Robbertse, P. J. 2006. Differences in salinity tolerance for growth and water-use efficiency in some amaranth (Amaranthus spp.) genotypes. New Zealand J. Crop Hortic. Sci. 34:11-22. [ Links ]
Ottow, E. A.; Brinker, M.; Teichmann, T.; Fritz, E.; Kaiser, W.; Brosché,M.; Kangasjärvi, J.; Jiang, X. and Polle, A. 2005. Populus euphratica displays apoplastic sodium accumulation, osmotic adjustment by decreases in calcium and soluble carbohydrates,and develops leaf succulence under salt stress. Plant Physiol. 139:1762-1772. [ Links ]
Pratap, V. and Sharma, Y. K. 2010. Impact of osmotic stress on seed germination and seedling growth in black gram (Phaseolus mungo). J. Environ. Biol. 31:721-726. [ Links ]
Radi, A. A.; Farghaly, F. A. and Hamada, A. M. 2013. Physiological and biochemical responses of salt-tolerant and salt-sensitive wheat and bean cultivars to salinity. J. Biol. Earth Sci. 3:B72-B88. [ Links ]
Reginato, M.; Sosa, L.; Llanes, A.; Hampp, E.; Vettorazzi, N.; Reinoso,H. and Luna, V. 2014. Growth responses and ion accumulation in the halophytic legume Prosopis strombulifera are determined by Na2SO4 and NaCl. Plant Biol. 16:97-106. [ Links ]
Reyes, R. E.; Padilla, B. L. E.; Pérez, V. O. y López, J. P. 2008. Historia,naturaleza y cualidades alimentarias del frijol. Rev. Invest.Científ. 4:1-21. [ Links ]
Richards, L. A. 1990. Diagnóstico y rehabilitación de suelos salinos y sódicos: manual núm. 60. Sexta reimpresión, Limusa. México.172 p. [ Links ]
Rogers, M. E.; Grieve, C. M. and Shannon, M. C. 1998. The response of lucerne (Medicago sativa L.) to sodium sulphate and chloride salinity. Plant Soil. 202:271-280. [ Links ]
Sánchez, B. E. I.; Ortega, E. M.; Gonzáles, H. V.; Camacho, E. M. y Kohashi, S. J. 2008. Crecimiento de plantas de papa (Solanum tuberosum L.) cv. Alpha, inducido por diversas soluciones salinas. Interciencia. 33:1-9. [ Links ]
Sánchez, B. E. I.; Ortega, E. H. M.; G. T. Sandoval, O. R. A.;Hernández, V. y Estrada, V. C. 2012. Lavado de sales en suelos aluviales costeros de Oaxaca, México, con aguas residuales municipales tratadas. Rev. Inter. Contaminación Amb. 28:343-360. [ Links ]
Shen, Y. Y.; Li, Y. and Yan, S. G. 2003. Effects of salinity on germination of six salt-tolerant forage species and their recovery from saline conditions. New Zealand J. Agric. Res. 46:263-269. [ Links ]
Slama, I.; Ghnaya, T.; Messedi, D.; Hessini, K.; Labidi, N.; Savoure, A. and Abdelly, C. 2007. Effect of sodium chloride on the response of the halophyte species Sesuvium portulacastrum grown in mannitol-induced water stress. J. Plant Res. 120:291-299. [ Links ]
SAS Institute. 1999. Statistical Analysis System (SAS) Versión 8 User’s guide. North Carolina, USA. 584 p. [ Links ]
Strogonov, B. P. 1964. Physiological basis of salt tolerance of plants (as affected by various types of salinity). Israel Program for Scientific Translations. New York, USA. 279 p. [ Links ]
Subbarao, G. V. and Johansen, C. 1994. Potential for genetic improvement in salinity tolerance in Legumes: Pigeon Pea. In: M. Pessarakli (Ed.). Handbook of Plants and Crop Stress. Marcel Dekker.New York, USA. 581-595 pp. [ Links ]
Velázquez, M. M. A.; Ortega, E. M.; Martínez, G. Á.; Kohashi, S. J. y García, C. N. 2002. Relación funcional PSI-RAS en las aguas residuales y suelos del Valle del Mezquital, Hidalgo, México.Terra Latinoam. 20:459-464. [ Links ]
Yildirim, E.; Güvenc, I. and Turkey. E. 2006. Salt tolerance of pepper cultivars during germination and seedling growth. Turk. J.Agric. For. 30:347-353. [ Links ]
Yokoishi, T. and Tanimoto, S. 1994. Seed germination of the halophyte Suaeda japonica under salt stress. Journal Plant Res.107:385-388. [ Links ]
Received: May 2017; Accepted: August 2017