Introduction
Sorghum has the potential to be incorporated into the diets of human populations worldwide, since being a gluten-free cereal represents an excellent option for people who suffer from intolerance or allergy to gluten. The whole or decorticated sorghum grain is widely used in different countries of Africa and Asia to prepare porridges, granulated foods, sausages, tea beverages, flour or semolina, from which pasta, cookies, tortillas, bread, and snacks are made. Statisticals indicate that more than 300 million people from developing countries use sorghum as their main source of energy (Awika and Rooney 2004, p. 1199; Kulamarva et al., 2009, p. 55; Yokomichi et al., p. 2015, 955; Xiong et al., 2019, p. 6).
Sorghum, represent one of the five most produced cereal worldwide and has been characterized as the cereal with the highest content of phenolic compounds, among which phenolic acids, 3-deoxianthocyanidins, and particularly procyanidins (PCs), stand out. Some pigmented varieties of sorghum reach PCs contents as high as 7-8%, concentrated in the outer grain layers which make up the bran (Desphande et al., 1986, p. 401). This value is comparable to 5-12% of PCs detected in grape seeds, one of the plant tissues with the highest content of PCs (Nakamura et al., 2003, p. 45).
Although PCs are often associated with negative physiological effects, such as reduction of minerals availability, and digestibility of starch and proteins (Cardoso et al., 2017, p. 372), they also represent multifunctional compounds that have the potential to inhibit the detrimental actions of a wide range of free radicals, metals, and enzymes involved on the oxidation of foods and on the pathogenesis of cardiovascular diseases and cancer (Dykes et al., 2014, p. 138). Because of the latter, cultivation and use of pigmented sorghum varieties (red and brown) with moderate and high content of PCs, has increased in agriculture and food industry, respectively (Awika and Rooney, 2004, p. 1199).
It is important to mention that sorghum PCs have structural characteristics different from the PCs of other foods frequently consumed within human diet, such as apple, cocoa, and grape (Gu et al., 2003, p. 7513). Sorghum PCs are distinguished by being oligomers or polymers constituted by (−)-epicatechin monomers as extension unit and (+)-catechin as terminal unit (Fig. 1). Unlike, apple and cocoa PCs are mainly composed of (−)-epicatechin units, whereas in the grape PCs, the gallated forms of (−)-epicatechin and (+)-catechin predominate.
Because of the importance of sorghum as a rich source of phenolic compounds that are beneficial for health, for several years some research groups have carried out relevant works focused on studying the antioxidant potential of these compounds in different varieties of sorghum and their products. Similarly, those studies have evaluated the antiradical potential of sorghum and its products based on two common free radicals: ABTS and DPPH (Awika et al., 2003, p. 6657; Dykes et al., 2005, p. 6813; Pontieri et al., 2016, p. 904; Wu et al., 2017, p. 17; Rao et al., 2018, p. 103; Xiong et al., 2019, p. 6). Other methods that correlate with biological effects have also been included, among these the oxygen radical absorbance capacity (ORAC) (Awika et al., 2003, p. 6657), the Ty1antiROS cell-based test (Pontieri et al., 2016, 904), and the ferric reducing ability of plasma assay (FRAP) (Rao et al., 2018, p. 103). In general, these methods are based on spectrophotometric measurements at a given time. Nowaday, data on the kinetic behavior, antiradical reactions that involve oxidative enzymes, and complexing of ion metals with PCs isolated from plant extracts, specially sorghum, are scarce.
The present work intends to broaden the knowledge of the antioxidant profile of sorghum phenolic compounds, focusing exclusively on PCs due to their abundance in this cereal, employing methodologies complementary to those used in the previously described studies. Among such methodologies are: monitoring of second-order rate constants (Ks) from the initial step to the overall reaction of PCs with DPPH, using a stopped-flow system; the easiness to reduce the peroxidase-generated free radicals (ABTS); inhibition of lipid peroxidation in a linoleic acid emulsion, as well as the ability to chelate copper [Cu(II)] and zinc [Zn(II)].
Considering the differences in the structure of sorghum PCs with respect to PCs of other plant tissues, their study may also reveal important aspects of the antioxidant structure-activity relationship against certain free radicals, enzymes, and metals involved in oxidation processes. One of the structural factors that has been poorly studied and which more controversial results has generated, is the degree of polymerization, given the structural diversity of PCs. Therefore, we have a special interest in comparing the antioxidant potential (antiradical and chelating) of the distinctive monomer and the most abundant and representative dimer of sorghum PCs, in this case (+)-catechin (C) and procyanidin B1 (PB1), respectively, against a procyanidin-rich fraction (PRF) that includes different degrees of polymerization. Under this perspective, the objective of the present study was to determine the antiradical and chelating abilities of (+)-catechin and procyanidin B1, in their pure form, and a PRF isolated from brown sorghum bran.
Materials and methods
Sorghum sample and bran
The experiment was carried out employing a variety of brown sorghum (SXR-19C), provided by a seed supplier from Los Mochis, Sinaloa, Mexico. Ten kg of cleaned sorghum sample were decorticated during 1.5 min to obtain the bran, using a decorticator developed and operated in the Laboratory of Silos and Mills of the Department of Research and Postgraduate in Food (DIPA) of the University of Sonora. The bran obtained was reduced to 40 mesh particle size, using an Epices Krups GX4100 mill, and then was frozen at −40 °C.
Chemicals
Procyanidin B1 was acquired from Chromadex (Irvine, California, USA). (+)-catechin, DPPH, ABTS, horseradish peroxidase type I, hydrogen peroxide (H2O2), linoleic acid, ammonium thiocyanate, ferrous chloride, potassium chloride (KCl), cupric sulfate pentahydrate (CuSO4.5H2O), zinc chloride (ZnCl2), hexamine, murexide, acetic acid, formic acid, ethanol, acetone, and methanol, were purchased from Sigma-Aldrich (St. Louis MO, USA). Acetonitrile and hydrochloric acid were acquired from J.T. Baker (Edo. de Méx., México). Deionized water (18 MΩ•cm-1) was prepared with a Milli-Q system (Millipore, Bedford, MA, USA).
Isolation of PRF
The isolation of PRF was performed from a phenolic extract of brown sorghum bran in a Sephadex LH-20 column (6 x 1.5 cm) manually packed and equilibrated with a mixture of 30% methanol/water (v/v) over 4 h. The phenolic extract was obtained as described in Mendoza-Wilson et al. (2016, 1). This extract was loaded onto the column and washed with 60 mL of neutral water, followed by 40 mL of 30% methanol/water (v/v). The PRF was finally recovered from the column by eluting with 80 mL of 70% (v/v) aqueous acetone. The eluents were evaporated to dryness under vacuum at 35 °C in a rotary evaporator. The dried sample was dissolved in milli-Q water for subsequent freezing and lyophilization (Gu et al., 2002, p. 4852).
Analysis of PRF by UHPLC-DAD-ESI-QTOF-MS system
The PRF was analyzed in an Agilent 1290 ultra-high performance liquid chromatography system (UHPLC) (Agilent Technologies, Santa Clara, CA), equipped with a UV-Vis diode array detector (DAD) model 1290, as well as electrospray ionization (ESI) and quadruple time of flight (QTOF) mass spectrometry (MS) detectors, Agilent 6530. The ESI was used in negative mode with a dual stream jet ionization source, with negative polarity and fragmentor 170. The equipment was operated using the MassHunter software (Agilent Technologies, Santa Clara, CA). Separation of compounds was carried out in an Agilent Eclipse Plus C18 reverse phase column of 1.8 μm and 50 x 2.1 mm. The injection volume was 10 μL, previous filtration of sample and standards through PVDF filters, 13 mm, GD/X, pore size 0.2 μm (Whatman, USA). The binary mobile phase consisted of (A) 0.1 % acetic acid/water (v/v), and (B) 0.1% acetic acid/acetonitrile (v/v) with a flow of 0.2 mL/min. The gradient used was based on Wu et al. (2017) with the following modifications: 0−0.5 min, 5% B; 0.5−10 min, 5−30% B; 10−12.5 min, 30−80% B; 12.5−15 min, 80% B; 15−15.5 min, 80−5% B; 15.5−23 min, 5% B. Detection was monitored at 280 nm and the run time was 23 min.
Determination of Ks during inhibition of DPPH free radical
The study of the kinetic behavior of C, PB1, and PRF during the inhibition of DPPH radical was performed through the calculation of their Ks. The initial stage (first hydrogen atom transfer) and the overall reaction were monitored, as explained in detail by Mendoza-Wilson et al. (2013, p. 61).The concentration of DPPH was 25 µg/mL, whereas a range of concentrations (10, 15, 20, 25 and 30 µg/mL) was used for C, PB1, and PRF. The solutions of DPPH, C, PB1, or PRF were prepared individually in 95% ethanol. For the kinetic reaction monitored during 300 s at 25 °C, the DPPH solution and the corresponding solution of PCs were mixed in equal volumes in a stopped-flow spectrometer, model SX20 (Applied Photophysycs Ltd, UK), equipped with two rapid mixing syringes and a 20 µL cell (dead-time 1 ms).
The absorption decay of DPPH was measured at 515 nm during reaction with each concentration of C, PB1 and PRF solutions, represented as ArOH in equation (1). In this way the pseudo-first-order rate constant (K obs) were obtained, based on the following model:
To calculate the Kobs, the experimental data of DPPH were transformed initially to natural logarithm (ln), and adjusted to a linear regression model. Later, to improve the linearity of the data, the values of DPPH at zero time ([DPPH]0) and Kobs were subjected to a second exponential transformation of the values of DPPH by the following equation:
As a last step, the Ks were obtained from the slope of a linear regression fit at the different concentrations of solutions of C, PB1, or PRF (ArOH).
Ability to reduce the peroxidase-generated ABTS radical cation
For the determination of the antiradical ability of C, PB1, and PRF in an oxidative reaction catalyzed by an enzyme present in living organisms, the easiness to reduce the peroxidase-generated ABTS radical cation was measured. The experiments were performed using the stopped-flow spectrometer SX20. Syringe 1, contained the peroxidase at concentration of 0.8 units/mL in 0.1 M phosphate buffer, pH 7.2. Syringe 2, contained the ABTS (400 μg), H2O2 (400 μg), and the PCs (40 μg); the solution was prepared in the same buffer. The ABTS formation was measured during 5 min by monitoring the absorbance at 734 nm (Chan et al., 2003, p. 787).
Inhibition of lipid peroxidation
For the assay of inhibition of lipid peroxidation, an emulsion was prepared by mixing 320 μL of Tween 20, 310 μL of linoleic acid, and 99.37 mL of 0.04 M phosphate buffer, pH 7.0. This mix was stirred in ultraturrax, 11,000 rpm for 3 min in the dark. Then, a reaction mixture containing 2.5 mL of emulsion plus 1 mL of solution of C, PB1, or PRF at 0.5 mg/mL, and 1.5 mL of 0.04 M phospate buffer pH 7.0, was prepared and kept 72 h at 40 °C in darkness. Peroxidation levels were determined by the thiocyanate method (Gülҫin et al., 2004, p. 393) and the percentage of inhibition of lipid peroxidation was calculated at 24, 48, or 72 h by the following equation:
Where AbsS = absorbance of the sample and AbsC = absorbance of the control.
Ability to chelate Cu(II) and Zn(II)
For determination of Cu(II) and Zn(II) chelating abilities, PCs samples were dissolved and diluted into the range 0.25-1.0 mg/mL, using 0.01 M hexamine/HCl buffer pH 5.0 with addition of 0.01 M KCl. Solutions of 0.25 mM CuSO4·5H2O or 0.8 mM ZnCl2 were prepared in the same buffer. Subsequently, a mixture was prepared adding 0.25 mL of sample solution, 0.25 mL of hexamine buffer, 0.5 mL of CuSO4.5H2O or ZnCl2, and 0.05 mL of 1 mM murexide. The mixture was subjected to vortex agitation and allowed to stand 10 min at room temperature. Absorbance was recorded at 482 nm and 530 nm for Cu(II), and 462 nm and 530 nm for Zn(II), employing the Cary 60 Bio spectrophotometer. Later the ratio of these absorbances (A482/A530 or A462/A530) was calculated. The amount of total or free Cu(II) and Zn(II) was determined in samples from a standard curve with concentrations in the range of 0.025−0.125 mM of CuSO4.5H2O or 0.4−2.0 mM of ZnCl2. The percentage of chelated Cu(II) or Zn(II) was calculated as the difference between total and free contents (Karamakć, 2009, p. 5485).
Statistical Analysis
All analyses were carried out in triplicate and the standard deviations were calculated. Linear or nonlinear regression analyses were performed for the calculation of Kobs and Ks; in this case, the NCCS statistical package was used, and the corresponding standard error was reported.
Results and discussion
Identification of compounds in PRF by UHPLC-DAD-ESI-QTOF-MS system
The isolated PRF from brown sorghum bran was subjected to analysis by UHPLC-DAD-ESI-QTOF-MS system and oligomers of PCs from trimer to decamer were identified, which were distributed in ten peaks, as is shown in Fig. 2a and Table 1.
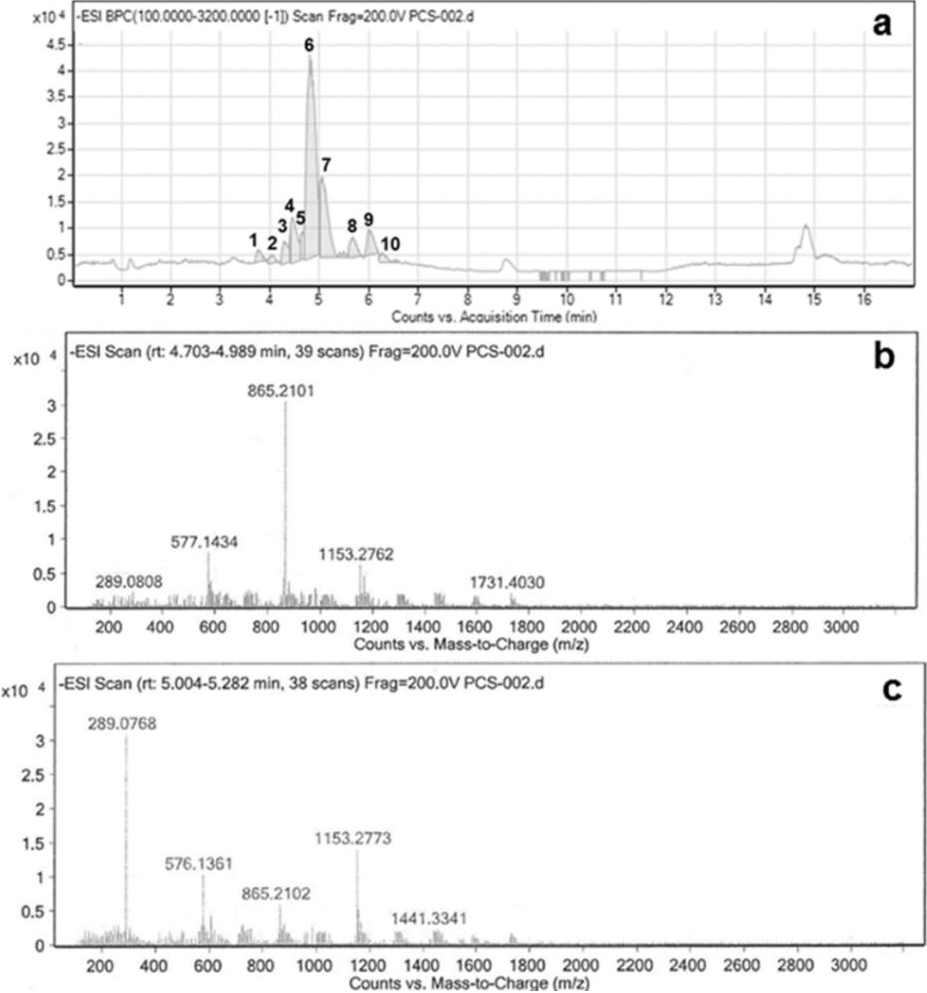
Fig. 2 Chromatogram of the procyanidin-rich fraction (PRF) analyzed in an ultra-high performance liquid chromatography system (UHPLC) equipped with an UV-Vis diode array detector (DAD), and detectors of mass spectrometry of electrospray ionization (ESI) and quadruple time of flight (QTOF). (a) Main fragments at m/z 1731, 1153, 865, 577, and 289, detected during identification of a decamer of PCs (peak 6); (b) Main fragments at m/z 1441, 1153, 865, 577, and 289, detected during identification of a nonamer of PCs (peak 7) (c).
Table 1 Identification of compounds in the procyanidin-rich fraction (PRF) of brown sorghum bran by UHPLC-DAD-ESI-QTOF system, in reverse phase, at 280 nm.
Peaks | Degree
of polimerization |
Retention time (min) |
Molecular ions (m/z) | Fragments of ions (m/z) |
---|---|---|---|---|
1 | Trimer | 3.754 | 865.21 | 863, 577, 575, 289 |
2 | Tetramer | 4.025 | 1153.26, 576.13 [M−2H]-2 | 865, 863, 577, 451, 289 |
3 | Tetramer | 4.394 | 576.13 [M−2H]-2 | 865, 863, 590, 577, 575, 289 |
4 | Pentamer | 4.462 | 1441.32, 719.66 [M−2H]-2 | 1153, 865, 863, 577, 575, 451, 425, 289, 287 |
5 | Pentamer | 4.703 | 719.66 [M−2H]-2 | 1153, 865, 863, 577, 575, 425, 289, 287 |
6 | Decamer | 4.8−4.989 | 1450.33 [M−2H]-2 | 1731, 1441, 1153, 865, 863, 577, 575, 289 |
7 | Nonamer | 5.004−5.282 | 1305.29 [M−2H]-2 | 1441, 1153, 865, 863,577, 576, 289, 287 |
8 | Heptamer | 5.659 | 1008.23 [M−2H]-2 | 1729, 1441, 1153, 865, 863, 713, 577 |
9 | Hexamer | 5.923−6.171 | 1729.40, 864.20 [M−2H]-2 | 1441, 1153, 865, 863, 575, 287 |
10 | Octamer | 6.209−6.585 | 1154.27 [M−2H]-2 | 1441, 865, 863, 577, 575 |
Peak 1. A trimer of PCs was detected which showed the molecular ion [M−H]− at m/z 865.21 and main fragments at m/z 577 and 289, produced by the loss of one and two units of (−)-epicatechin/(+)-catechin. Additional fragments were found at m/z 863 and 575, similar to those previously reported in other works (Helström et al., 2007, p. 157; Mendoza-Wilson et al., 2016, p. 1).
Peaks 2 and 3. Tetramers of PCs were identified in these peaks by their characteristic ion [M−H]− at m/z 1153.26 and also the double-charged ion [M−H]−2 at m/z 576.13. Main fragments at m/z 865, 577, and 289, plus additional fragments at m/z 575, 451, 425, and 287 were observed, which had already been reported in other works (Helström et al., 2007, p. 157; Mendoza-Wilson et al., 2016, p. 1).
Peaks 4 and 5. Pentamers of PCs were found in these peaks due to the production of a molecular ion [M−H]− at m/z 1441.32 and the double-charged molecular ion [M−H]−2 at m/z 719.66. Main fragments at m/z 1153, 865, 577, and 289, as well as additional fragments at m/z 863, 575, 451, 425, and 287 were detected, which were corroborated with similar studies works (Helström et al., 2007, p. 157; Mendoza-Wilson et al., 2016, p. 1).
Peak 6. An abundant decamer of PCs was identified by the presence of a double-charged molecular ion [M−H]−2 at m/z 1450.33. Main fragments at m/z 1731, 1441, 1153, 865, 577, and 289 (Fig. 2b), plus additional fragments at m/z 863 and 575 supported the presence of this decamer in agreement with previous reference works (Helström et al., 2007, p. 157).
Peak 7. The identification of a nonamer was done due to the presence of a double-charged molecular ion [M−H]−2 at m/z 1305.29. Main fragments were observed at m/z 1441, 1153, 865, 577, and 289 (Fig. 2c). This result is supported by similar reports (Helström et al., 2007, p. 157).
Peak 8. A heptamer was found by the presence of a double-charged molecular ion [M−H]−2 at m/z 1008.23. Main fragments were visualized at m/z 1729, 1441, 1153, 865, and 577, besides of additional fragments at m/z 863 and 713, as in previous works (Helström et al., 2007, p. 157; Mendoza-Wilson et al., 2016, p. 1).
Peak 9. The hexamer located in this chromatogram region showed an ion [M−H]− at m/z 1729.40 and the double-charged ion [M−H]−2 at m/z 864.20. Main fragments at m/z 1441, 1153, 865, and 577 and three additional fragments at m/z 863, 575, and 287 were observed in agreement with previously reported data (Helström et al., 2007, p. 157; Mendoza-Wilson et al., 2016, p. 1).
Peak 10. A octamer also was detected due to the presence of a double-charged molecular ion [M−H]−2 at m/z 1154.27. Main fragments at m/z 1441, 1153, 865 and 577, as well as additional fragments at m/z 863, and 575, were observed similar to Helström et al. (2007, p. 157).
Antiradical ability
Free radicals can be generated in both food and living organisms, in media of different polarities, for example in an aqueous medium (polar) or colloidal medium such as the cell membrane (non-polar). For this reason, in the present work was determined the ability of C, PB1 and PRF to inhibit two free radicals (DPPH and ABTS) in polar solutions, as well as the lipid peroxidation of linoleic acid in emulsion.
Determination of Ks during the inhibition of DPPH free radical
Antioxidants have the function of reacting with oxidizing agents (free radicals, enzymes, transition metals) at a rate faster than biomolecules to prevent the oxidation of the latter. In this sense, the use of stopped-flow methods, which monitor the reaction of antioxidants with free radicals from the beginning, and in the course of time (kinetics) until reaching the equilibrium phase, are relevant (Mendoza-Wilson et al., 2013, 61). The latter is due to the capacity of stopped-flow methods to detect absorbance changes in the order of 0.002 units. These changes, although small, implicate a great difference in antiradical ability (Hiromi and Kanaya, 1988, 445).
As is appreciated in Fig. 3a, the kinetic reaction of C, PB1, and PRF with DPPH, occurred in two stages: an initial short-fast and a longer-slow one. The short-fast stage, during the first 5 s, established a clear difference among the samples. C showed the lowest value of Ks in the short-fast stage, 790 (μg/mL)-1 s-1 compared to 6870 and 1640 (μg/mL)-1 s-1 for PB1 and PRF, respectively. This result suggest that the oligomers of PCs were notably more effective (2−8 times) than the monomer to inhibit the DPPH radical. The fact that the PB1 dimer showed a Ks 4 times higher than the PRF, can be attributed to that PB1 was in its pure form, while PRF was a complex mixture of larger oligomers, ranging from trimer to decamers, competing to react with DPPH.
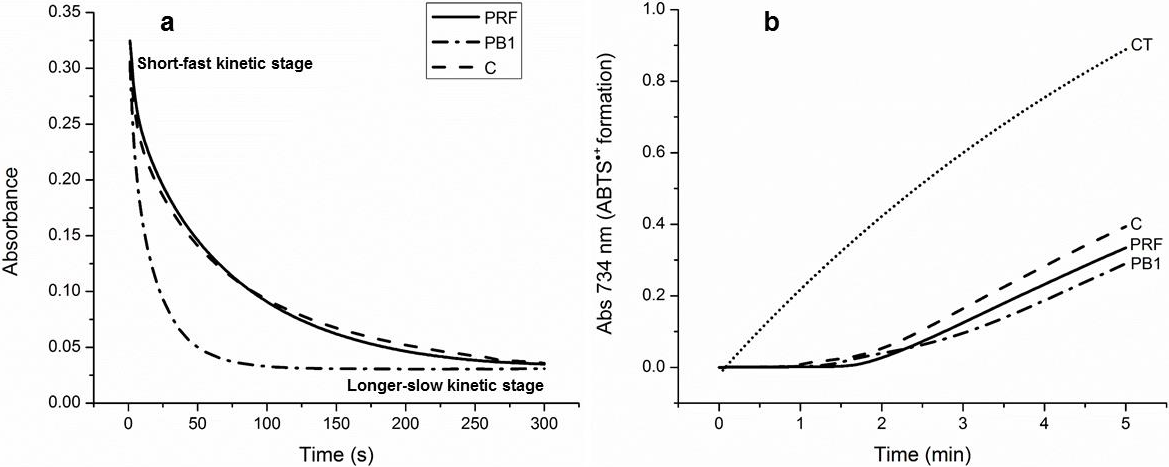
Fig. 3 Representative kinetic behavior of (+)-catechin (C), procyanidin B1 (PB1), and the procyanidin-rich fraction (PRF) within 300 s in the reaction with the DPPH free radical (each at concentration of 25 μg/mL), in 95% ethanol at 25 °C. (a) Ability of (+)-catechin (C), procyanidin B1 (PB1), the procyanidin-rich fraction (PRF) and a sample control (CT) to reduce the ABTS generated by peroxidase during 5 min of reaction; (b) Data are expressed as the mean of three replicates within the same experiment; standard error were very low, in the order of 10-4 − 10-6, so are no reported.
The overall reaction was monitored for 300 s (5 min) and it was observed that, at the beginning of the longer-slow stage, Ks decreased considerably, which may be due to side reactions that lead to mixtures of oxidation products (Tsimogiannis et al., 2006, 140). However, it is worth noting that at this stage PRF reached higher Ks , 120 (μg/mL)-1 s-1, than PB1, 83 (μg/mL)-1 s-1 and C, 82 (μg/mL)-1 s-1. These results indicate that the mixture of oligomers with higher degree of polymerization, was more effective than PB1 and C to inhibit free radicals in the longer-slow stage. The latter is probably due to the formation of intermediates that can maintain their stability for a longer time during redox reactions.
Ability to reduce the peroxidase-generated ABTS radical cation
Peroxidases are a group of enzymes present in human, animals, and plants that catalyze the simultaneous oxidation of two substrates using H2O2 as electron acceptor. The phenolic compounds, depending on their structure, can act as substrates or inhibitors of peroxidases and, in both cases, to exert antioxidant effects. However, when acting as substrates, sometimes form reactive phenoxyl radicals that co-oxidize free radicals, generating pro-oxidant activity (Chan et al., 2003, p. 787). This pro-oxidant activity therefore needs to be taken into account when quantifying antioxidant activity. Among the methods used for determining the antioxidant profile, the easiness to reduce or co-oxidize the peroxidase-generated ABTS radical cation provides a way to measure also pro-oxidant effects. Currently, no published data have been found, which simultaneously relate the antioxidant and pro-oxidant potential of the compounds analyzed in this study. The knowledge of such data is relevant considering that the antioxidant effects or, on the contrary, the oxidative damage that PCs could cause if they will form unstable phenoxy radicals when reacting with peroxidases, could occur in vivo.
In relation to the above, Fig. 3b shows that during the first min of reaction, C, PB1, and PRF (peroxidase substrates) avoided the formation of ABTS. Then the production of this radical cation began at a very low rate and it stayed that way during the 5 min in that the reaction was monitored. The initial rate of formation of ABTS was 0.0108±0.0002 Abs734/min for C; 0.00888±0.0004 Abs734/min for PB1; and 0.0086±0.0003 Abs734/min for PRF. All of these initial rates of formation of ABTS were significantly lower than that shown by the control (0.2083±0.0008 Abs734/min). This result indicates that C, PB1, and PRF did not produce pro-oxidant effects and therefore act as effective antioxidants in oxidation reactions catalyzed by the peroxidase enzyme. It should be noted that PB1 and PRF, that is, the oligomers of PCs, showed greater antioxidant potential than monomer C during peroxidase activity, since their initial rates of formation of ABTS were lower.
Inhibition of lipid peroxidation
Hydroperoxides are reactive compounds that are formed from fatty acids which are susceptible to oxidation, such as linoleic acid, leading to generation of free radicals at the level of cell membrane, and in dietary matrices rich in fatty acids (García et al., 2010, p. 28). For this reason, the determination of inhibition of lipid peroxidation in an emulsion system with linoleic acid, can provide information about the ability of PCs to exert antiradical activity in heterophasic systems that simulate both polar and non-polar phases of membranes and food matrices.
Analysis carried out in this regard showed that both C, PB1, and PRF mantained a tendency to increase the percent of inhibition of lipid peroxidation during 24, 48, or 72 h at 40 °C; reaching the maximum values at a concentration of 0.50 mg/mL (Fig. 4). In the evaluated time intervals, PB1 showed higher values (85.19%, 85.70%, 96.25%) than PRF (72.31%, 83.20%, 91.64%), and C (78.89%, 83.39%, 89.93%). Based on these data, it can be deduced that the degree of polymerization is not a determining factor in the behavior of PCs, when they inhibit the free radicals generated in emulsion systems with linoleic acid. However, the percent of inhibition of lipid peroxidation of sorghum PCs, in pure form and as a mixture, were higher than those reported in wheat bran (70.7%) and rice bran (69.5%) (Sultana et al., 2008, p. 205).These results are important, since they place the brown sorghum bran as a tissue constituted by bioactive compounds with high capacity to protect linoleic acid from oxidation.
Ability to chelate Cu(II) and Zn(II)
Cu(II) and Zn(II) are vital dietary nutrients required in small amounts for the proper functioning of human organism (Osredkar and Sustar, 2011, p. 001). These metals are in their free ionic form or linked to proteins, acting as a catalytic or structural component of an extensive number of enzymes and proteins involved in normal cellular processes. Nevertheless, they are also related to some adverse effects, especially at excessive concentrations (Quesada et al., 2011, p. 153). In the case of Cu(II), this can act as a catalyst in oxidation reactions promoting the formation of highly harmful free radicals (hydroxyl radical) by reacting with H2O2, generating even pro-oxidant effects (Fenton reactions) (Fernández et al., 2002, p. 105). On the other hand, excess Zn(II) particularly impairs Cu(II) absorption in intestinal cells, causing an imbalance Zn(II)/Cu(II) that can generate diseases (Osredkar and Sustar, 2011, p. 001). Both metals have pH- sensitive binding sites on intestinal mucus that affect their absorption. The binding of metal ions, which is a proton-coupled process, is favored at the mildly acidic pH typically found in the micro-environment of the gastrointestinal mucus layer (pH 5.0 − 5.5). Aversely, values of pH around 5.5 to 6 may facilitate dissociation of the metal-mucus complex and decrease their absorption, as with Zn(II) (Powell et al., 1999, p. 147). Antioxidants can prevent adverse reactions of metals that are absorbed at high concentrations, by chelating them. Due to the aforementioned reasons and to the fact that most metal chelation studies have been made at physiological pH (7.4), it is important to measure the affinity towards Cu(II) and Zn(II) of compounds with chelating ability, such as PCs, at pH 5. The affinity of PCs towards the transition metals depends on their structure, concentration, and pH.
In Fig. 5, it is clear that the PRF had a greater ability than PB1 and C to form complexes with Cu(II), as they reached maximum percentages of chelation of 81%, 64.6%, and 62.9%, respectively, at a concentration of 1.0 mg/mL. This result indicates that the ability of sorghum PCs to chelate Cu(II) at pH 5, increase with the degree of polymerization. Under these conditions, the PRF of sorghum showed chelating properties of Cu (II) similar to those reported for an extract rich in PCs from almonds (80-90%) (Karamać, 2009, p. 5485).
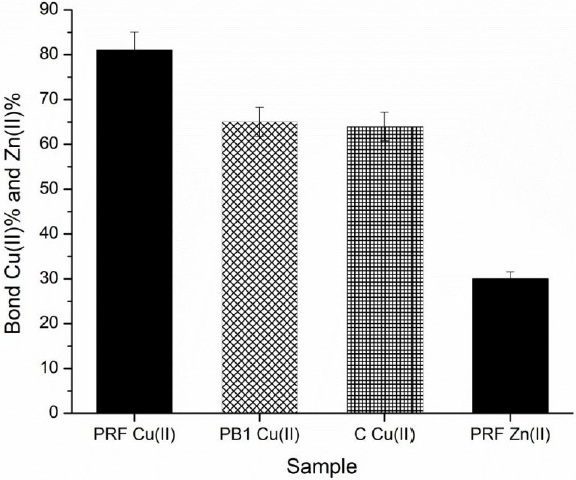
Fig. 5 Percentages of bonded Cu(II) by (+)-catechin (C), procyanidin B1 (PB1) and the procyanidin-rich fraction (PRF), plus the percentage of bonded Zn(II) by PRF, at pH 5.0.
Regarding Zn(II) chelating ability, PRF showed very low affinity for this metal compared to CU(II), since it reached a chelation percentage of 30.3%. Although it is noteworthy that PCs from sorghum showed greater capacity to form complexes with Zn(II) unlike the PCs of walnuts, hazelnuts, and almonds, which reached percentages of chelation of 0%, 2% and 28.4%, respectively, under the same conditions (pH 5, 1mg/mL) (Karamać, 2009, p. 5485).
Previous research had already shown that the dimers and monomers of PCs have low affinity for Zn(II) at acid pH, reason why the percentage of chelation of Zn(II) in C and PB1 was not determined in the present work. In this context, a study conducted by Karamać (2007, p. 357), using a buckwheat groats tannin fraction with high content of low molecular weight oligomers, including procyanidin dimer B2 (PB2), found a low percentage of Zn(II) chelation (13.5%) at pH 5. Similarly, the C monomer, in pure form, showed very low affinity for Zn(II) at pH 5. In this case the interaction was measured as zinc precipitation and resulted less than 20% (McDonald et al., 1996, p. 599).
The affinity for Zn(II) at acid pH from fractions rich in PCs isolated from vegetal tissues, it had only been published for buckwheat, buckwheat groats, chestnut, quebracho, halzenut almonds, and walnuts, but not for sorghum PCs. Although a similar trend is observed in the PCs of different cereals and fruits, in that their affinity for Zn(II) is low at pH 5, sorghum PCs showed greater ability to chelate this metal in such conditions.
Conclusions
In general terms, data obtained in this study corroborated that PCs of sorghum, mainly PRF (mixture of oligomers from trimer to decamer), possess great potential to inhibit free radicals such as DPPH, ABTS, and those generated from the peroxidation of linoleic acid, as well as for the chelation of Cu (II), but showed very low affinity for Zn (II) at acid pH. Additionally, C, PB1, and PRF did not produce prooxidant effects when reacting with free radicals (ABTS) generated by oxidative enzymes such as peroxidase.
The degree of polymerization marked a very important effect on the activity of the PCs against free radicals DPPH and ABTS, in a polar reaction medium after the reaction time elapsed. In both cases, the dimer PB1 and PRF were more effective than monomer C to directly inhibit free radicals and also to decrease the rates of formation of these in the presence of peroxidase. However, apparently the degree of polymerization is not a determining factor in the behavior of PCs to inhibit free radicals formed during the peroxidation of linoleic acid in a emulsion system.
Regarding the chelating ability, this was greater as the degree of polymerization increased, as can be visualized in the following order: monomer (C) < dimer (PB1) < trimer − decamer (PRF).