Introduction
Bathomorphi is a diverse monophyletic lineage comprising around 689 species, representing more than half of the chondrichthyan diversity (Ehemann et al., 2018; Last et al., 2016; Nelson, 2016; Serena et al., 2020; Weigmann, 2016). Yet, approximately 36% of batomorphs are listed by the IUCN Red List of Threatened Species in endangered categories (i.e., critically endangered, endangered, and vulnerable) and more than 12.3% of the total are in the category deficient data (Dulvy et al., 2014, 2021; IUCN, 2020; Last et al., 2016), indicating the lack of knowledge in this diverse group. Rays are endangered by a variety of threats and as a predatory, opportunistic, forager group in complex food webs, the reduction of the batomorphs populations can impact and alter the functioning of ecosystems (Dulvy & Reynols, 2002; Graham et al., 2001).
The general body plan of batomorphs includes dorsoventrally flattened bodies, enlarged pectoral fins connected to the head, and ventrally located gill slits (Ebert & Winton, 2010; McEachran & de Carvalho, 2002); however, within this basic shape this group shows diverse morphologies and sizes (Compagno, 1999; Rosenberger, 2001). Its interspecific and intraspecific variations are related to ecological and phylogenetic patterns (Ekstrom & Kajiura, 2013; Franklin et al., 2014). The interspecific variations of the disc, ranging from rhomboidal to circular, were found to be related to locomotion and habitat use (Ekstrom & Kajiura, 2013; Franklin et al., 2014; Parson et al., 2011). While intraspecific variation in tooth shape was related to sexual dimorphism (Feduccia & Slaughther, 1974; Navarro-González et al., 2018; Sáez & Lamilla, 2004). In general, morphological variation in teeth was related to the diet of the species; however, males also use their more pointed teeth to hold onto the female during mating (Kajiura & Tricas, 1996).
In fish species, body shape is important in determining swimming performance (Aguilar-Medrano et al., 2013; Fulton, 2007; Wainwright et al., 2002). However, a number of other aspects of the organism’s function were also linked to its morphology, such as diet and reproduction, and therefore could also interact with the evolution of locomotor capacities (Collar et al., 2008). Because the phenotypic and trophic variation of the species has been used to lay the basis to determine their functional relationships (Aguilar-Medrano et al., 2019; Braga et al., 2012; Fabre et al., 2016; Lobato et al., 2014; Losos, 2009; Price et al., 2010), in the present study, the morphological variation of the disc and feeding structures, as well as trophic data of 9 species of Bathomorphi were analyzed and phylogenetic comparative methods were used to determine whether the morphological variation is related to the trophic ecology of the group. Finally, because the locomotor system in fish is conditioned by factors linked to the ecological mechanisms and responsible for their evolution, we expected that disc shape would be phylogenetically related to feeding structures (Meckel’s cartilage -MC from now on-, and teeth) of the species here analyzed (Langerhans & Reznick, 2010).
Materials and methods
Specimens used in this study (Table 1) were obtained from the Ichthyological Collection of the Center for Research and Advanced Studies of the National Polytechnic Institute (CINV-NEC), Merida, Mexico. No living specimens were collected or killed during this study. The study followed the protocols of use and management of organisms of the ichthyological collection, which operates according to the national protocols of the Ministry of Environment and Natural Resources (Semarnat).
Table 1 Museum specimens used in the present study obtained from the Ichthyological Collection of the Center for Research and Advanced Studies of the National Polytechnic Institute (CINV-NEC). WD: Disc width range and mean; n: number of organisms studied; TI: trophic index. Items found in their diet of the species, BF: bonny fish; JF: juvenile-small fish; SH: shrimp; CR: crabs; CL: clams; WO: worms.
Common name | Order | Species | WD | WD | WD | n | TI | BF | JF | SH | CR | CL | WO |
---|---|---|---|---|---|---|---|---|---|---|---|---|---|
min | max | mean | |||||||||||
cm | cm | cm | |||||||||||
Sting rays | Myliobatiformes | Hypanus americanus | 18.2 | 32.4 | 24.3 | 9 | 3.5 | 1 | 1 | 1 | 1 | 1 | |
Gymnura micrura | 19.7 | 67.0 | 29.3 | 45 | 3.9 | 1 | 1 | 1 | 1 | ||||
Urobatis jamaicensis | 6.5 | 21.5 | 13.4 | 26 | 3.6 | 1 | 1 | 1 | 1 | ||||
Hardnose skates | Rajiformes | Dipturus olseni | 8.9 | 46.6 | 31.5 | 16 | 3.8 | 1 | 1 | 1 | |||
Fenestraja sinusmexicanus | 3.9 | 21.8 | 15.0 | 22 | 3.9 | 1 | 1 | 1 | |||||
Rostroraja ackleyi | 16.3 | 21.4 | 19.6 | 8 | 3.7 | 1 | 1 | 1 | 1 | ||||
Rostroraja texana | 6.0 | 37.4 | 20.2 | 36 | 3.8 | 1 | 1 | 1 | |||||
Guitarfish | Rhinopristiformes | Pseudobatos lentiginosus | 9.7 | 21.3 | 14.8 | 24 | 3.6 | 1 | 1 | 1 | |||
Electric ray | Torpediniformes | Narcine bancroftii | 3.1 | 23.6 | 13.8 | 40 | 3.1 | 1 | 1 | 1 | 1 |
The selection of species was based on taxonomy, trying to have the broadest taxonomic representation, and on the availability of specimens in the Ichthyological Collection for morphometric analyses and mandibular bone extraction. Nine species representing the 4 orders of batomorphs were analyzed (Last et al., 2016). From the order Myliobatiformes, we included Hypanus americanus (Hildebrand & Schroeder, 1928), Gymnura micruraYokota & De Carvalho, 2017, and Urobatis jamaicensis (Cuvier, 1816). From the order Rajiformes, we included Dipturus olseni (Bigelow & Schroeder, 1951), Fenestraja sinusmexicanus (Bigelow & Schroeder, 1950), Rostroraja ackleyiGarman, 1881, and Rostroraja texanaChandler, 1921. From the order Rhinopristiformes, we included Pseudobatos lentiginosus (Garman, 1880), and from the order Torpediniformes, we included Narcine bancroftii (Griffith & Smith, 1834).
Although the diet of the species may change according to the availability of resources and geographical distribution for the purpose of this study, the general diet is necessary to understand changes in the morphology of the species -e.g., if the species feeds on soft or hardshell items, or if the items are mobile or static (Ross, 1986). To determine the diet of the species, a list of items consumed by each species was constructed with information collected from Fishbase (Froese & Pauly, 2019), Robertson and Van Tassell (2019), and specialized literature (Jargowsky et al., 2019; Michael 1993; O’Shea et al., 2017; Queiroz et al., 2019; Stehmann et al., 1978; Yokota & De Carvalho, 2017). The trophic index value (TI) was also obtained from Fishbase. The TI is calculated using a list of items known to occur in the diet of each species, based on information of stomach content published in books and scientific articles, and results in a numerical value ranging from 2.0 to 4.7, where a primary consumer, which consumes mainly plant/detritus (herbivores), may have a TI between 2.0 and 2.19, while consumers which consume mainly animals (carnivores) may have values equal to or greater than 2.8 (Costa & Cataudella, 2007).
To analyze the morphological variation of the disc, photographs of 226 museum specimens in dorsal and ventral views were taken and the disc width was measured. We tried to ensure that there was a representation of females and males for each species. Geometric morphometric methods were used to quantify the variation in the shape and size of the specimens (Rohlf, 1999; Zelditch et al., 2004). To analyze the geometric morphometric variation, 28 marks were used in the dorsal, and 28 in the ventral view (Fig. 1 a, b). The configurations were optimally superimposed using a Generalized Procrustes Analysis to obtain a shape coordinates matrix (Rohlf, 1999; Rohlf & Slice, 1990). Centroid size (CS), which is an estimator of size was calculated (Bookstein, 1991; Monteiro et al., 2005). Relative Warps (RWs), including both uniform and non-uniform components were calculated from the Procrustes data and used as shape variables (Bookstein, 1991; Rohlf, 1993). The main axes of shape variation were explored by a principal component analysis (PCA) and to get a better appreciation of the shape variation of the main PCA axes, a thin-plate spline algorithm was used to produce transformation grids that represented the extreme positive and negative deviations along the axis (Bookstein, 1991). Geometric morphometric analyses were performed in the Tps software series (Rohlf, 2015).

Figure 1 Morphological analyses of batoids of the Gulf of Mexico. a, Marks in the dorsal view. b, Marks in ventral view. Red dots: landmarks, blue dots: semilandmarks. E: Eye, Sp: spiracle, PrF: pectoral fin, PvF: pelvic fin, Cp: clasper, T: tail. N: Nostril, NC: nasal curtain, M: mouth, G: gills slits, Cc: cloaca. c, Mandible showing the tooth pad with red cross lines. TP: Tooth pad, PQ: palatoquadrate, C: condilo, MC: Meckel’s cartilage, S: sinfisis, MS: mandibular sinfisis. d, Lengths of the MC showing the tooth pad with red cross lines. 1: Total length of the MC; 2: total length of the tooth pad (TP) in the MC; 3: height of the anterior axis of the MC; 4: height of the TP on the anterior axis of the MC; 5: height of the TP in the central area of the MC; 6: height of the central area of the MC; 7: height of the MC at the posterior axis of the TP. e, Types of crowns found in the teeth of the species studied.
To test if there are sexual or allometric constraints in the observed morphological variation of the disc, size, using the centroid size (CS), and sexes, segregating the shape variation in groups of females and males per species were analyzed. The relationship of the shape variables (landmarks matrix) and the centroid size (CS) was tested using regression analyses in TpsRegr, version 1.41 (Rohlf, 2015). Then, we searched for differences in the morphological variation by sexes. ANOVA was performed on the shape variables using sexes. All statistical analyses were done in PAST 3.11 (Hammer et al., 2001).
To analyze the variation of the MC (Fig. 1 c, d), the mandibles were removed, cleaned, and measured. Following specialized literature, 7 measurements were taken in the MC: 1) total length of the MC, 2) total length of the tooth pad (TP), 3) height of the anterior axis of the MC, 4) height of the anterior axis of the TP, 5) height of the TP in the central area, 6) height of the central area of the MC, and 7) height of the MC at the posterior axis of the TP (Dean & Motta, 2004; Navarro-González et al., 2018; Sáez & Lamilla, 1997, 2004, 2012). To avoid the effect of the size of the specimens, all measurements were presented as proportions of the total length by dividing by the total length of the MC. The resulting values were transformed by logarithm and segregated by sexes. PCA was performed to determine the main axis of variation and the possible grouping of the species.
The tooth pad of the palatoquadrate and MC (Fig. 1 e) were photographed, and the teeth were classified according to the shape of the crown (enameled part of the tooth). The main characteristics considered from the crown were if the surface was smooth or bumpy, the presence of a cusp or ridges, and when the cusp was present, if it was rounded or angular (Supplementary material 1; Radinsky, 1961; Underwood et al., 2015). The teeth of each species were described by sexes. Using the teeth classification by species and sex, a PCA was performed to determine the main axis of variation and possible grouping.
The molecular sequences of the COI mitochondrial gene were downloaded from BoldSystems (http://www.boldsystems.org) and GenBank (http://www.ncbi.nlm.nih.gov/genbank/) for 7 species (Supplementary material 2). Phylogenetic reconstruction was inferred with the maximum likelihood method and the Tamura-Nei model, using 1,000 bootstrap replicas in MegaX (Felsenstein, 1985; Kumar et al., 2018; Tamura & Nei, 1993).
The phylogenetic independent contrasts (PIC) model was used to evaluate the correlation between morphological (disc-shape, MC, and tooth shape), trophic, and size variation throughout the phylogeny. The PIC model estimates the regression parameters for a phylogenetic generalized least-squares analysis (Felsenstein, 1973, 1985; Freckleton, 2012). Finally, the variables with significant relationships were optimized onto the phylogeny to examine trait evolution. Estimates of the state of the ancestral nodes were made using maximum likelihood on a Brownian motion model. PIC model, optimization, and estimation of the ancestral nodes were carried out in the R environment version 3.6.2 (R Development Core Team, 2011) using the packages ape (Paradis et al., 2004), geiger (Harmon et al., 2008), Phytools (Revell, 2012), fastAnc (Revell, 2012), contMap (Revell, 2013), and nlme (Pinheiro et al., 2014).
Results
The Rajiformes D. olseni, F. sinusmexicanus, R. ackleyi, and R. texana, as well as the Myliobatiformes species G. micrura, presented the highest values of the trophic index, ranging from 3.7 to 3.9. The main items in their diet were shrimp and crab, but also included bony fish and worms. The Myliobatiformes, H. americanus, and U. jamaicensis, showed mean trophic index values of 3.5 and 3.6; they feed on shrimp, clams, bony fish, crabs, and worms. Pseudobatos lentiginosus also had a mean trophic index value of 3.6, and feeds on shrimp, crabs, and clams. Finally, N. bancroftii had the lowest trophic index value, 3.1, which was calculated based on closely related species; however, it feeds on the same items as the Rajiformes, thus we considered its trophic index value should be revised (Table 1).
The results of the PCA of the dorsal view (Fig. 2) indicated that 2 PCs were sufficient to explain 82% of the morphological variation within all species. The first PC summarized 64% of the variation and separated rhomboidal disc-shapes, with high-aspect ratio pectoral fins, short space between both pectoral fin insertions, and small eyes (i.e., G. micrura PC1-) from arrow-shaped discs, with low-aspect ratio pectoral fins, wide space between both pectoral fin insertions, and large eyes (i.e., P. lentiginosus PC1+). The second PC summarized 18% of the variation and separated rounded disc-shapes with low-aspect ratio pectoral fins with a short distance between the eye and the snout (i.e., H. americanus, PC2-), from rhomboidal disc-shapes, with medium-aspect ratio pectoral fins with a larger distance between the eye and the snout (i.e., R. texana PC2+).
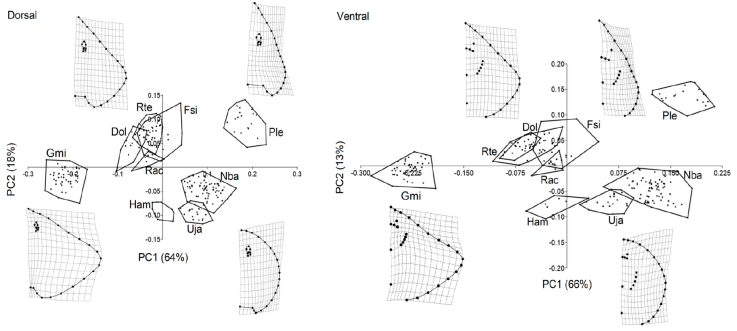
Figure 2 Principal component analyses (PCA) of the dorsal and ventral view of the disc. Species codes. Dol: Dipturus olseni, Gmi: Gymnura micrura, Ham: Hypanus americanus, Fsi: Fenestraja sinusmexicana, Nba: Narcine bancroftii, Ple: Pseudobatos lentiginosus, Rac: Rostroraja ackleyi, Rte: Rostroraja texana, Uja: Urobatis jamaicensis.
The PCA of the ventral view (Fig. 2) indicated that 2 PCs were sufficient to explain 79% of the morphological variation within all species. The first PC summarized 66% of the variation and separated wide rhomboidal disc-shapes, with high-aspect ratio pectoral fins, short mouth, nearby gills, and all in a higher position relative to the pectoral girdle (i.e., G. micrura PC1-), from narrow, rounded disc-shapes, wider mouths, separate gills, and the most posterior below the height of the pectoral girdle (i.e., N. bancroftii PC1+). The second PC summarized 13% of the variation and separated the rounded disc-shapes, with narrowed mouths and close to the snout, separated gills, and the most posterior in a position below the height of the pectoral girdle (i.e., H. americanus, PC2-), from arrow-shaped discs, wide mouths, far from the snout, nearby gills, and all higher than the pectoral girdle (i.e., F. sinusmexicanus PC2+).
The regression results indicated that the morphology of F. sinusmexicanus, G. micrura, N. bancroftii, P. lentiginosus, R. texana, and U. jamaicensis changed with development (Table 2). The main variation between small and large specimens was the width and length of the disc and the length of the snout. Small specimens had shorter (length, snout to tail) and wider discs, and an elongated snout, while larger specimens had elongated (length, snout to tail) discs, and shorter snouts (Fig. 3). ANOVA only showed sexual dimorphism in G. micrura and R. texana (Table 3). Males of G. micrura and R. texana had more elongated snouts and discs (snout to tail) than females (Fig. 3).
Table 2 Generalized Goodall F-test of the morphological variation and size (centroid size) of 9 species of Batoids.
Views | Dorsal | Ventral | |||
---|---|---|---|---|---|
df | F | p | F | p | |
D. olseni | 52, 676 | 0.67 | 0.97 | 0.57 | 0.99 |
F. sinusmexicanus | 52, 1,040 | 4.94 | 0.00 | 3.75 | 0.00 |
G. micrura | 52, 2,340 | 1.37 | 0.04 | 1.06 | 0.37 |
H. americanus | 52, 312 | 1.30 | 0.09 | 0.76 | 0.89 |
N. bancroftii | 52, 1,924 | 6.44 | 0.00 | 1.11 | 0.28 |
P. lentiginosus | 52, 1,144 | 1.11 | 0.28 | 2.18 | 0.00 |
R. ackleyi | 52, 364 | 0.55 | 0.99 | 0.77 | 0.88 |
R. texana | 52, 1,768 | 3.25 | 0.00 | 4.86 | 0.00 |
U. jamaicensis | 52, 1,196 | 1.56 | 0.01 | 1.27 | 0.11 |

Figure 3 Allometric variation by size and sex observed in the dorsal and ventral views. Species codes. Fsi: Fenestraja sinusmexicana, Rte: Rostroraja texana, Gmi: Gymnura micrura, Ple: Pseudobatos lentiginosus, Nba: Narcine bancroftii, Uja: Urobatis jamaicensis. CS: Centroid size, smaller shapes (-); larger sizes (+). Sex: Sexual dimorfism, females (F), males (M).
Table 3 ANOVA comparing disc shape among sexes of 9 species of Batoids. F: Female, M: male, n: number of organisms.
Species | Fn | Mn | p dorsal | p ventral |
---|---|---|---|---|
D. olseni | 8 | 7 | 0.277 | 0.858 |
F. sinusmexicanus | 10 | 12 | 0.324 | 0.260 |
G. micrura | 22 | 24 | 0.027 | 0.172 |
H. americanus | 6 | 2 | fail | fail |
N. bancroftii | 41 | 29 | 0.320 | 0.231 |
P. lentiginosus | 11 | 12 | 0.378 | 0.165 |
R. texana | 19 | 17 | 0.031 | 0.066 |
U. jamaicensis | 17 | 8 | 0.951 | 0.578 |
No sexual dimorphism was found in the MC (F = 0.398; p = 0.864). Two components were sufficient to add 96% of the variation. The first PC1 added 91% of the variation and segregated G. micrura (PC1+) from the rest of the species because it did not have exposed teeth on the front of the MC. On the other edge of the axis, on the very edge was U. jamaicensis (PC1-) with a wide tooth pad. PC2 added 5% of the variation and segregated N. bancroftii (PC2+) in the extreme due to a very reduced tooth pad, from D. olseni, P. lentiginosus, R. ackleyi, and F. sinusmexicanus, which presented an elongated tooth pad (Fig. 4; Table 4).
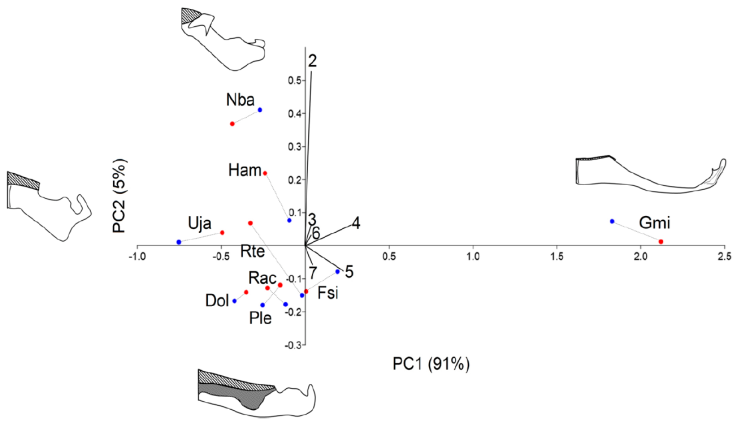
Figure 4 Principal component analyses (PCA) of the measurements of the Meckel’s cartilage (MC). All measurements were transformed, dividing them by the MC total length. 2: Total length of the tooth pad (TP) in the MC; 3: height of the anterior axis of the MC; 4: height of the TP on the anterior axis of the MC; 5: height of the TP in the central area of the MC; 6: height of the central area of the MC; 7: height of the MC at the posterior axis of the TP. Species codes. Dol: Dipturus olseni, Gmi: Gymnura micrura, Ham: Hypanus americanus, Fsi: Fenestraja sinusmexicana, Nba: Narcine bancroftii, Ple: Pseudobatos lentiginosus, Rac: Rostroraja ackleyi, Rte: Rostroraja texana, Uja: Urobatis jamaicensis. Blue dots: males; red dots: females. Meckel’s cartilage illustrations representing the variation on each axis, PC1+G. lessae, PC1-U. jamaicensis, PC2+N. bancroftii, PC2-P. lentiginosus. Toothed area lined.
Table 4 Mean values per species of the Meckel’s cartilage (MC) measurements. All measurements were transformed, dividing them by the MC total length. 2: Total length of the tooth pad (TP); 3: height of the anterior axis of the MC; 4: height of the anterior axis of the TP; 5: height of the TP in the central area; 6: height of the central area of the MC; 7: height of the MC at the posterior axis of the TP.
Species | 2 | 3 | 4 | 5 | 6 | 7 |
---|---|---|---|---|---|---|
D. olseni | 1.53 | 4.85 | 8.38 | 16.66 | 4.92 | 5.33 |
F. sinusmexicanus | 1.64 | 7.13 | 20.07 | 30.71 | 6.30 | 6.03 |
G. micrura | 2.52 | 8.13 | 541.50 | 541.50 | 6.25 | 7.32 |
H. americanus | 2.95 | 4.92 | 15.12 | 19.08 | 4.92 | 4.28 |
N. bancroftii | 5.15 | 6.05 | 9.85 | 15.41 | 4.83 | 3.99 |
P. lentiginosus | 1.49 | 4.93 | 12.88 | 20.52 | 4.27 | 4.30 |
R. ackleyi | 1.56 | 5.19 | 11.11 | 27.56 | 4.08 | 3.84 |
R. texana | 1.95 | 4.54 | 14.14 | 22.19 | 4.56 | 4.22 |
U. jamaicensis | 2.17 | 3.06 | 8.24 | 9.54 | 2.96 | 3.27 |
The teeth were classified by species and sexes (Table 5). Males tend to have more angular pointed teeth than females and the palatoquadrate had sharper teeth than the MC. Fourteen types of teeth were registered (Fig. 5): a and b are globe-shaped teeth, the crown is soft and rounded, b has a small cusp with soft edges, both shapes, a and b, were specific to P. lentiginosus; c to g were the most common teeth, they had smooth crowns and range from flat (a and d), to rounded (e and f) and angular pointed (g) cusps; h and i presented and 2 ridges, respectively, h is specific of P. lentiginosus; j, k, and l presented bumpy crowns and ranged from flat to medium-size, rounded cusp, k and l were specific to U. jamaicensis. Finally, in m and n, the crown is an angular cusp; these teeth are specific to G. micrura. The PC1 (Fig. 5) segregated teeth with smooth crowns (i.e., D. olseni; PC1+) from teeth with bumpy crowns (i.e., P. lentiginosus, H. americanus; PC1-), while PC2 segregated crowns with a cusp (i.e., N. bancroftii; PC2+), from flat crowns (i.e., R. ackleyi; PC2-).
Table 5 Teeth morphology. F: female; M: male. MC: Meckel’s cartilage; PQ: palatoquadrate. 1: presence. Crown shapes described in Figure 1. Main characteristics of the crowns, s: smooth; b: bumpy; c: with cusp; r with ridges
Crown shape | s | c | s | c | c | c | c | r | r | b | bc | bc | c | c | ||
---|---|---|---|---|---|---|---|---|---|---|---|---|---|---|---|---|
Order /species | Sex | Structure | a | b | c | d | e | f | g | h | i | j | k | l | m | n |
H. americanus | F | MC | 1 | |||||||||||||
PQ | 1 | |||||||||||||||
M | MC | 1 | ||||||||||||||
PQ | 1 | |||||||||||||||
G. micrura | F | MC | 1 | |||||||||||||
PQ | 1 | |||||||||||||||
M | MC | 1 | ||||||||||||||
PQ | 1 | |||||||||||||||
U. jamaicensis | F | MC | 1 | 1 | ||||||||||||
PQ | 1 | 1 | ||||||||||||||
M | MC | 1 | ||||||||||||||
PQ | 1 | 1 | ||||||||||||||
D.olseni | F | MC | 1 | 1 | ||||||||||||
PQ | 1 | 1 | 1 | |||||||||||||
M | MC | 1 | 1 | 1 | 1 | |||||||||||
PQ | 1 | 1 | 1 | 1 | 1 | |||||||||||
F. sinusmexicanus | F | MC | 1 | |||||||||||||
PQ | 1 | 1 | ||||||||||||||
M | MC | 1 | 1 | 1 | ||||||||||||
PQ | 1 | 1 | ||||||||||||||
R. ackleyi | F | MC | 1 | 1 | ||||||||||||
PQ | 1 | |||||||||||||||
M | MC | 1 | 1 | |||||||||||||
PQ | 1 | |||||||||||||||
R. texana | F | MC | 1 | 1 | ||||||||||||
PQ | 1 | |||||||||||||||
M | MC | 1 | 1 | |||||||||||||
PQ | 1 | 1 | ||||||||||||||
P. lentiginosus | F | MC | 1 | 1 | ||||||||||||
PQ | 1 | |||||||||||||||
M | MC | 1 | 1 | |||||||||||||
PQ | 1 | |||||||||||||||
N. bancroftii | F | MC | 1 | 1 | ||||||||||||
PQ | 1 | 1 | ||||||||||||||
M | MC | 1 | 1 | |||||||||||||
PQ | 1 | 1 |

Figure 5 Principal component analyses (PCA) of the teeth morphology. Species codes. Dol: Dipturus olseni, Gmi: Gymnura micrura, Ham: Hypanus americanus, Fsi: Fenestraja sinusmexicana, Nba: Narcine bancroftii, Ple: Pseudobatos lentiginosus, Rac: Rostroraja ackleyi, Rte: Rostroraja texana, Uja: Urobatis jamaicensis. Blue dots: males; red dots: females.
Two of the 4 Rajiformes species were not included in the phylogeny, F. sinusmexicanus, R. ackleyi, because no molecular sequences of the COI mitochondrial gene were found. The phylogeny produced 3 clades: one for Rajiformes, R. texana, and D. olseni; a second for Myliobatiformes, G. micrura, H. americanus, and U. jamaicensis; and a third clade groups the Rhinopristiformes P. lentiginosus, and Torpediniformes N. bancroftii. The bootstrap consensus showed the strongest support, 99% for the 2 Rajiformes, followed by H. americanus, and U. jamaicensis with 70%, and P. lentiginosus and N. bancroftii with 62%.
PIC showed a significant relationship between the PC1 of MC and the ventral (r = 0.67; p = 0.02) and dorsal (r = 0.64; p = 0.03) views of the disk-shape (Fig. 6). PC2 separated the MC and the ventral (r = 0.86; p = 0.002) and dorsal (r = 0.72; p = 0.01) views of the disk-shape. PC1 captured the tooth variation and PC2 the dorsal view (r = 0.57; p = 0.04) of the disk-shape. Finally, the only morphological variable related to the trophic index was PC2 of the Meckel’s cartilage (r = 0.81; p = 0.005; Supplementary material 3).

Figure 6 Morphological and trophic characters with significant relationship analyzed with the phylogenetic independent contrasts method. PC: Principal component. T: Teeth variation, TI: trophic index, DSD: disc-shape dorsal view, DSV: dish-shape ventral view, MC: Meckel’s cartilage. Rte: Rostroraja texana, Dol: Dipturus olseni, Nba: Narcine bancroftii, Ple: Pseudobatos lentiginosus, Gmi: Gymnura micrura, Uja: Urobatis jamaicensis, Ham: Hypanus americanus. Transformation grids and illustrations of the structures represent the extremes and central variation of the character through the axis measured. Red arrows indicate the variables that are related. For regression values see Supplementary material 3.
Discussion
Factors such as the ecosystems in which the species inhabit, their sexual behavior, and their phylogenetic relationships act synergistically, producing the current morphological diversity of species. The present study was able to reveal a wide range of morphological variations by analyzing different morphological structures. Furthermore, it was possible to determine the morphological characters that phylogenetically covary with the diet of the species by analyzing the morphological and trophic variation through the evolutionary history of species.
With the analyses of the morphological variation of the disc, 3 main morphologies were obtained. Both views of the disc, dorsal and ventral, showed the same grouping pattern, indicating that the shape of the disc is the variable that summarizes most of the variation of the group. The first morphology of the disc was represented by P. lentiginosus, which has a very angular disc, with low-aspect ratio pectoral fins, and a wide space between the insertions of the pectoral fins. This specific disc morphology of P. lentiginosus is also combined with an elongated body and strong tail, characters not considered in the present study. This species swims by moving its thick tail and caudal fin in side-to-side motions and only uses its pectoral fins for acceleration and maneuvering similar to the way sharks swim (Rosenberger, 2001; Wilga & Lauder, 2000). This style was also found in most basal members of the batomorph clade, such as the sawfishes, which primarily used their thick tails to swim through the water (Rosenberger, 2001; Wilga & Lauder, 2000).
The second disc morphology, wide rhomboidal discs, groups D. olseni, F. sinusmexicanus, R. ackleyi, R. texana, and G. micrura. This last species presented the widest disc and because of that, it was separated from the main group (Fig. 2). All these species are demersal inhabitants (Robertson & Van Tasell, 2019). Species with this morphology mainly use intermediate fin movements between undulatory and oscillatory locomotion; when in the benthos they use an undulatory behavior and switch to a more oscillatory motion when swimming in the water column (Franklin et al., 2014; Rosenberger, 2001).
The third disc morphology, circular disc-shapes with low aspect-ratio, groups U. jamaicensis, H. americanus, and N. bancroftii. This disc shape has been related to undulatory-style locomotion, which is related to benthic habitats and mainly sedentary species that swim slowly along the bottom (Franklin et al., 2014; Rosenberger, 2001). However, N. bancroftii has a thick tail that, like P. lentiginosus, it uses for locomotion along with its caudal and pectoral fins. Narcine bancroftii is a demersal species, found in shallow coastal waters buried beneath the sand, mud, or swimming among the seagrass beds (Di Santo & Kenaley, 2016; Last et al., 2016).
The morphological variation of the MC was similar to that of the disc and was the only variable evolutionarily related to the diet of the species. The species D. olseni, F. sinusmexicanus, R. ackleyi, R. texana, and P. lentiginosus presented even, elongated, and strong MC with an elongated tooth pad. These species showed high values of the trophic index, and among their diet, crabs were a shared prey, thus a strong and elongated MC and tooth pad can help to exert powerful compressive forces for durophagy (Dean & Motta, 2004). On the other hand, in U. jamaicensis and H. americanus, the MC and tooth pad were smaller and less thick; these 2 species have a broader diet, sharing prey such as fish, shrimp, clams, and worms. The analysis showed 2 MC independent morphologies, N. bancroftii, which has a thick MC in an angular position and the shortest tooth pad. This morphology has been related to protrusion, allowing the species to have functional flexibility to capture buried prey (Dean et al., 2008). The other extreme is represented by G. micrura, which presented a very elongated and thin MC without frontal teeth, and a wider mouth useful for feeding on large prey like fish, shrimp, crabs, and clams (Dean et al., 2008).
The teeth of all the Rajiformes studied here, as well as G. micrura and N. brancroftii, had smooth crowns, while the majority of Myliobatiformes and the F. sinusmexicanus species had teeth with bumpy crowns. According to our results, tooth variation was evolutionarily related to the disc shape and was sexually constrained in most species, except for 2 non-dimorphic species, R. ackleyi and P. lentiginosus. In all other species, the males had sharper teeth than the females; also, the palatoquadrate had sharper teeth than the MC, which could indicate that the main grip depends on the palatoquadrate when the males hold onto the females during mating (Kajiura & Tricas, 1996). Allometry was observed for most species. Smaller specimens had wider discs and a longer snout, while larger specimens had longer discs and a smaller snout. This may indicate that as the specimen develops, snout growth decelerates, while the disc width and length expand, and consequently that the growth rate of the snout is different from that of the rest of the body (fins).
Two of the species studied here had unique teeth; G. micrura had teeth with angular cusps, while P. lentiginosus had crowns with ridges and smooth. A species that also featured a wide rhomboidal disc and a slender and elongated MC is G. micrura, a demersal species with feeding preferences for mobile prey such as fish and shrimp. For this type of prey, wide and light mandibular structures allow fast movements, further, pointy teeth allow it to hold and tear prey (Aguilar-Medrano, 2017; Burres et al., 2015; Dean et al., 2007), while a wide disc favors fast movements. On the other hand, P. lentiginosus, which presented a highly angular disc with low aspect-ratio and thick and elongated MC, is a benthic species with feeding preferences for prey such as mollusks and crustaceans, which may include hard-shell prey. For this type of prey, wide and heavy mandibular structures combined with smooth and ridged teeth allow exerting powerful compressive forces for durophagy (Aschliman, 2014; Rutledge et al., 2019; Summers, 2000). Considering the above discussed, the combined analyses of morphological structures in batomorphs can reveal a wide range of ecological information, especially useful for those poorly known species.
There were species with different teeth between females and males such as F. sinusmexicanus, H. americanus, G. micrura, and U. jamaicensis. From these species, our study also found sexual dimorphism in the disc shape of G. lessae, and Jargowsky et al. (2019) found sexual variation in its diet. The complete difference in the morphology of the teeth may be related to resource partitioning, that is, differential feeding to alleviate intraspecific competition for food (Albo-Puigserver et al., 2015; Platell et al., 1998; Rastgoo et al., 2018; Taniuchi & Shimizu, 1993).
All the Myliobatiformes analyzed here are distributed in the Gulf of Mexico and the Caribbean, while the Rajiformes are mainly distributed in the Gulf of Mexico (Last et al., 2016; Robertson & Van Tassell, 2019). According to our results, the morphological variation in all the structures analyzed here was more cohesive among Rajiformes than in Myliobatiformes. Previous studies have indicated that diversification rates are higher in the tropics than in the temperate biomes (Rolland et al., 2014), a hypothesis that fits our results, where those species inhabiting the Caribbean and the Gulf of Mexico presented a broader morphological diversification than those that only inhabit the Gulf of Mexico.
Our results support the wide and intricate morphological variation in batomorphs. However, we recommend caution in the interpretation of the results for H. americanus and R. ackleyi for which we have a low sample (Table 1). An interesting result of this study is the phylogenetic relationship of the MC with the diet of the species. Meckel’s cartilage plays a central role in food capture and processing, as in P. lentiginosus, where the strong and thick mandibular structures allow durophagy, in G. micrura, where elongated and thin mandibular structures allow a wide mouth opening, and in N. bancroftii, where the thick and angular mandibular structures allow protrusion.
Another interesting result of this analysis is the evolutionary relationship of the disc shape, MC, and teeth, indicating that the morphological variation of the different structures studied is coupled to enhance the swimming and feeding process. However, since the morphological variation of batomorphs plays an important role in determining their habitat and lifestyle, deviations from the general pattern have important consequences. This is seen in the demersal species N. brancroftii, which presents similar disc morphology to that of the 2 benthic species, U. jamaicensis and H. americanus, but the presence of a thick tail allows N. brancroftii to use a different locomotion style and a different habitat.
Although our results account for sexual dimorphism in the teeth of some species, to delve into this topic, it would be necessary to develop more specific analyses of the diet of the species by sex, since, although the trophic index is useful in the absence of other data, it also limits the analysis and comparison between species. Finally, our study summarizes an important amount of information, concluding that the morphological variation of the disc, mandibular structures, and teeth are correlated through the evolution of the group and respond to functional patterns such as swimming and feeding, which finally, determine the ecology of the species.