Introduction
The betalains, naturally occurring pigments in most of the families of the plant order Caryophyllales [1], fungi of the genus Amanita [2], and proteobacterium Gluconacetobacter diazotrophicus [3], are responsible for the red-violet and yellow-orange colour found in flowers, bracts, fruits, stems, leaves, and roots [4]. The genus Amaranthus belongs to the order Caryophyllales and to the largest eudicot clade with C4-based photosynthetic pathway species and consists of nearly 75 species found worldwide [5-7]. Amaranth was sacred to and eaten by Aztecs [6]. Examination of an archaeological grain of amaranth found in Coxcatlan cave in Puebla, Mexico, has revealed that Amaranthus hypochondriacus L. and Amaranthus cruentus L. were harvested for thousands of years [8]. Despite the genetic diversity among the large number of species in the genus Amaranthus, the A. hypochondriacus is the major crop cultivated in Mexico.
The discoveries on the high content in high-quality protein, essential minerals including iron, calcium, magnesium, phosphorus, and potassium, and unsaturated fatty acids in amaranth (Amaranthus spp) overcoming other cereals such as wheat, maize, or rice made important to introduce amaranth and its products into the market [9,10]. Besides Mexico’s traditional amaranth foods such as “Alegria” that is popped amaranth seeds confection, there is an array of consumer food products including breakfast cereals, baked items, sprouts, and betalains pigments [11]. Thus, molecular characterizing amaranth species may facilitate the amaranth breeding to feed the ever-growing consumer demand for amaranth.
Betanin, which is a betalain, was first isolated from “red beetroot” (Beta vulgaris L.) in 1918 by Schudel [12] and approved as natural food colourant E162 in defined amounts specified by the Food and Drug Administration (FDA). Betalains from amaranth were approved as a natural food colourant in China as well (National Food Safety Standard Use for Food Additive, GB2760-2011). Betalains are divided into two classes the betacyanins and betaxanthins. Red-violet betacyanins are an array of compounds that appear as betalamic acid condensed with either cyclo-DOPA or glycosylated cyclo-DOPA that can be further decorated with added glucosyl or glucuronosyl groups or subjected to acylation of sugar moieties, decarboxylation by removing carboxyl groups, and dehydrogenation, whereas yellow-orange betaxanthins are compounds with frameworks displaying the betalamic acid condensed with an imino or amino group of amino acids [4, 13,14].
Betacyanins are organized in at least seven distinctive groups that differ in attaching glucosyl groups to the oxygen atoms in the ortho position on the cyclo-DOPA moiety as well as in the position of the glycosyl- or glucuronosyl-linkage moites [4, 15]: betanin-type, gomphrenin-type, amaranthin-type, melocactin-type (Bougainvillea-r-I-type), oleracin-type, apiocactin-type, and glabranin-type (Bougainvillea-v-type). Amaranthin is the main betacyanin in the genus Amaranthus. Besides the betacyanin behaviour as pigment, biological activities have been reported for betalains such as anti-inflammatory-, antioxidant-, and immunomodulatory-activities and cancer chemoprotective properties [16, 17].
Most efforts have been focused on quantifying betacyanins and betaxanthins using multicomponent spectrometric analysis in the presence of interfering substances [18]. Despite multiple betacyanins and betaxanthins are present in the plant tissue samples, the concentration of betacyanins based on betanin and betaxanthins based on vulgaxanthin-I give in the total betalains content [19]. High-Performance Liquid Chromatography coupled with a diode array detector (DAD) has simplified to survey different betacyanins and betaxanthins, and LC coupled to tandem MS has been led by recording the m/z value of the parent ion and its tandem fragmentation to distinguish different betacyanins and betaxanthins, and its derivatives. Here, we used LC-MS to define the betalain composition in red amaranth (A. cruentus) to valorize the leaves and inflorescences as betalain pigments source.
Experimental
Plant material
The A. cruentus seeds were originally collected in Valladolid, Yucatan in 2021, and identified by Ph.D. Ivonne Sánchez del Pino (Taxonomist) at Scientific Research Center of Yucatán (CICY). A. cruentus plants were grown at the outdoor experimental garden of CICY. The leaves and inflorescences were collected from 60-days-old A. cruentus plants, immediately frozen in liquid nitrogen, lyophilized at -51 °C for 72 h, ground to powder with a blade mill, and stored at -20 °C until use.
Chemicals and reagents
Methanol (MeOH) and water (H2O) HPLC-grade were from Tedia (Fairfield, USA). Methanol and water LC-MS-grade were obtained from Tedia (Fairfield, USA). Acetic acid LC-MS-grade was obtained from Fisher Scientific (Waltham, USA). Red beet extract was obtained from Sigma-Aldrich (Cat. # 901266, St. Louis, MO, USA) and used as reference material (RM). Sorbent BondElut-C18 was purchased from Agilent Technologies (40 mm particle size, Santa Clara, CA, USA).
Betalain extraction from plants tissues
Total betalains were extracted from 100 mg of tissue powder. After 400 mg of sorbent BondElut-C18 was ground with tissue powder with mortar and pestle at room temperature, the mix was packed in an empty SPE cartridge. Next, elution was performed with 9 mL of H2O made 0.1 % acetic acid, and then with 9 mL of MeOH:H2O (1:1, v/v) made 0.1 % acetic acid using a vacuum manifold system (Visiprep SPE Vacuum Manifold, Sigma-Aldrich, United States), both eluents were collected separately and evaporated. The residues were resuspended in 1 mL of H2O made 0.1 % acetic acid and transferred to an amber vial (MS-grade) for LC-MS analyses.
High-Performance Liquid Chromatography (LC) coupled with LTQ-Orbitrap Mass Spectrometry (MS)
The analysis by LC-MS Orbitrap was performed using an Ultimate 3000 UHPLC (Thermo Scientific, Waltham, MA, USA) coupled to an UV/Vis detector (Ultimate 3000 UV/VIS detector, Dionex), and to a LTQ-Orbitrap Elite mass spectrometer (Thermo Fisher Scientific, San Jose, CA) equipped with a Heated Electrospray Ionization interface (HESI-II, Thermo Fisher Scientific, Waltham, MA, USA). A 20 mL aliquot of the sample was injected, transferred to an stainless steel loop and then to a Hypersil GOLD C18 column (100 × 2.1 mm, 1.9 mm particle size) at a flow rate of 300 mL/min. Sample was eluted from the column at a flow rate of 300 mL/min using a binary gradient composed of H2O made 0.1% acetic acid (solvent A) and MeOH made 0.1% acetic acid (solvent B). The gradient settings were as follows: 0-30 min from 5% to 100%, isocratic for 10 min, then returning to 5% and isocratic for 10 min for column reconditioning. UV/Vis spectra data were collected at 480 and 540 nm. MS spectra data were acquired in positive mode: MS1 resolution 60,000, scan range 100-1500 m/z; MS2 resolution 60,000, scan range 100-600 m/z. Higher-energy collisional dissociation (HCD) was used as a fragmentation method by applying 50 eV. Peak areas of each compound based in MS1 mode using the extracted ion chromatograms were determined with Xcalibur 4.1 software (Thermo Scientific, USA). Graphs were obtained using GraphPad Prism version 9.0 (GraphPad, San Diego, CA, USA).
Results and discussion
Betalains determination in commercial reference material (RM)
Given that reference betalains are unavailable, RM got from Sigma-Aldrich was used as a reference to identify betanin chromatographic peaks accurately and to further characterize the chromatographic behavior of other unavailable betalains. The HPLC chromatogram of the RM obtained at 540 nm wavelength showed two high absorbing peaks with retention time (Rt) of 3.93 and 6.76 min, respectively (Fig. 1(A)). Both peaks were baseline separated and displayed an intense [M+H]+ ion at m/z 551.1482, as expected for betanin and isobetanin, and MS2 spectra got with HCD 50 eV were almost same. The two peaks yielded the MS2 ion at m/z 389.09, suggesting the presence of hexoside (Fig. 1(B) and 1(C)). In betanin-type betalains, the glucoside is attached through the C5-oxygen [20]. Isobetanin is the epimer of betanin at the chiral center of C15, and greater Rt value has been reported for isobetanin than betanin that is explained to some extent by slightly less polar isobetanin than betanin based on dipole moment estimation [21, 22]. The baseline separation of the two peaks, MS1, and MS2 spectra data lead to confident identification of betanin and isobetanin.
Identification and relative quantification of 43 betalains by LC-MS
Next, we used betanin as a reference, recording the absorbance at 540 nm in-line with LC-MS analysis, to find out betalains in leaves and inflorescences samples from the red amaranth (A. cruentus). Red amaranth plant parts were almost, if not all, red colours. Stem, petioles, and leaves, as well inflorescences display red colours (Fig. 2). A catalog of betalains consisting of 68 betalains chromatographically separated and confident identified by Xie and Chen (2021) [23] from Chenopodium formosanum cultivars was also used as a refence since C18 column was used in this study as well. Overall, the high of the absorbing peaks in the leaves were lower than in the pair peaks in inflorescences, and the highest absorbing peaks matched to the peaks 1 and 2, which are the major compounds in both chromatograms, corresponding to amaranthin and isoamaranthin (Fig. 3(A) and 3(B)). The [M+H]+ ion at m/z 727.1803 and the MS2 ions after fragmented with 50 eV in HCD showed in the Fig. 3(C) and 3(D) were similar to the data from the literature for amaranthin isolated from stems and inflorescences of Amaranthaceae plants including the genus Amaranthus [20, 24, 25], petal of Gomphrena globosa [26], and inflorescences of Celosia argentea var. cristata and Celosia argentea var. plumosa [27]. The lower retention got for both amaranthin and isoamaranthin than betanin agrees with the previously reported data [23, 24]. The fact that Rt value for amaranthin is smaller than that of betanin can be explained by the glucuronic acid attached to the glucose moiety. In amaranthin-type betalains, the glucuronic acid is attached through the C2-oxygen of the glucose moiety.
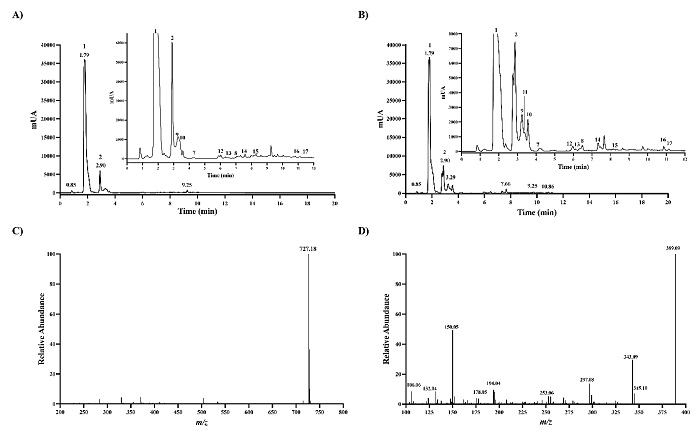
Fig. 3 LC-MS analysis of red amaranth samples. Liquid chromatography-ultraviolet chromatogram got at 540 nm in leaves samples (A) and inflorescence samples (B). The inset shows the expanded region of the chromatogram with retention time of 1-12 min. (C) MS1 spectra got for peak 1. (D) MS2 spectra got with HCD 50 eV for peak 1.
Based on the mass measurement accuracy on Orbitrap Elite, 43 betalains were identified with mass measurement errors of less than 5 parts per million (ppm) (Table 1). MS2 spectra data obtained for betalains were used to confirm the accurate betanin identification in amaranth samples and to compare with MS2 spectra data previously reported for samples of Chenopodium formosanum cultivars [23], Melocactus species [28], Beta vulgaris cultivars [29, 30], and Amaranthus species [24]. Among the betalains in the samples of red amaranth, six amaranthin-type, two gomphrenin-type, 22 betanin-type, and 13 betaxanthins were detected. Amaranthin-type includes amaranthin, isoamaranthin that is the epimer of amaranthin, and amaranthin-derivatives (Fig. 4). Gomphrenin-type includes gomphrenin-I and its epimer. Betanin-type include prebetatin, betanin, betanidin, isobetanin, phyllocactin, isophyllocactin, lampranthin II, isolampranthin II, and betanin-derivatives. It has been reported that decarboxylation and oxidation of betacyanins produce betacyanin-derivatives, usually strengthened by high temperatures [2, 31, 32]. When comparing the betacyanin-derivatives, we found a smaller number of amaranthin-derivatives than betanin-derivatives. Betaxanthins that were identified in red amaranth include amine-derived betaxanthins, for instance, histamine-, tyramine-, and dopamine-betaxanthin. Non-polar amino acid-derived betaxanthins, for instance, tryptophan-, phenylalanine-, leucine-, alanine-, and proline-betaxanthin, and polar amino acid-derived betaxanthins such as serine-, threonine-, and glutamine-betaxanthin were also identified.
Table 1 Chromatographic and MS data for betalains in red amaranth samples.
# | Compound | Retention time (Rt) | Relative Rt to betanin | Chemical Formula | Theorical m/z [M+H] | Observed m/z [M+H] | Mass error (ppm) | Fragments | ||
Amaranthin-type | ||||||||||
1 | Amaranthin | 1.85 | 0.53 | C30H34N2O19 | 727.1826 | 727.1803 | -2.3 | 389.09, 343.09, 297.08, 194.04, 150.05 | ||
2 | Isoamaranthin | 2.70 | 0.78 | C30H34N2O19 | 727.1826 | 727.1806 | -2.0 | 389.09, 343.09, 297.08, 194.04, 150.05 | ||
3 | 17-decarboxy-amaranthin | 3.07 | 0.89 | C29H34N2O17 | 683.1930 | 683.1918 | 1.2 | 389.09, 343.09, 297.08, 194.04, 150.05 | ||
4 | 17-decarboxy-isoamaranthin | 6.05 | 1.76 | C29H34N2O17 | 683.1930 | 683.1915 | 1.5 | 389.09, 343.09, 297.08, 194.04, 150.05 | ||
5 | Decarboxy-(iso)amaranthin | 7.68 | 2.23 | C29H34N2O17 | 683.1930 | 683.1922 | 0.8 | 389.09, 343.09, 297.08, 194.04, 150.05 | ||
6 | Neo-amaranthin | 7.25 | 2.11 | C30H32N2O19 | 725.1672 | 725.1628 | 4.3 | 389.09, 343.09, 297.08, 194.04, 150.05 | ||
Gomphrenin-type | ||||||||||
7 | Gomphrenin-I | 4.06 | 1.18 | C24H26N2O13 | 551.1508 | 551.1475 | -3.26 | 389.09, 343.09, 297.08, 194.04, 150.05 | ||
8 | Isogomphrenin-I | 6.87 | 2.00 | C24H26N2O13 | 551.1508 | 551.1473 | -3.53 | 389.09, 343.09, 297.08, 194.04, 150.05 | ||
Betanin-type | ||||||||||
9 | Prebetanin | 3.19 | 0.93 | C24H26N2O16S | 630.1003 | 630.0961 | -4.24 | 389.09, 343.09, 297.08, 194.04, 150.05 | ||
10 | Betanin | 3.93 | 1.00 | C24H26N2O13 | 551.1508 | 551.1483 | -2.51 | 389.09, 343.09, 297.08, 194.04, 150.05 | ||
11 | Betanidin | 3.44 | 1.00 | C18H16N2O8 | 389.0979 | 389.0941 | -3.77 | 389.09, 343.09, 297.08, 194.04, 150.05 | ||
12 | Isoprebetanin | 6.01 | 1.75 | C24H26N2O16S | 630.1003 | 630.0979 | -2.39 | 389.09, 343.09, 297.08, 194.04, 150.05 | ||
13 | Isobetanin | 6.76 | 1.92 | C24H26N2O13 | 551.1508 | 551.1481 | -2.69 | 389.09, 343.09, 297.08, 194.04, 150.05 | ||
14 | Phyllocactin | 7.71 | 2.25 | C27H29N2O16 | 638.1596 | 638.1574 | -2.17 | 389.09, 343.09, 297.08, 194.04, 150.05 | ||
15 | Isophyllocactin | 8.46 | 2.47 | C27H29N2O16 | 638.1596 | 638.1574 | -2.23 | 389.09, 343.09, 297.08, 194.04, 150.05 | ||
16 | Lampranthin II | 11.00 | 3.21 | C34H34N2O16 | 727.1989 | 727.1946 | -4.31 | 389.09, 343.09, 297.08, 194.04, 150.05 | ||
17 | Isolampranthin II | 11.34 | 3.31 | C34H34N2O16 | 727.1989 | 727.1946 | -4.29 | 389.09, 343.09, 297.08, 194.04, 150.05 | ||
18 | 17-decarboxy-betanin | 5.83 | 1.70 | C23H26N2O11 | 507.1609 | 507.1574 | -3.52 | 345.10, 299.10, 194.04,150.05, 106.06 | ||
# | Compound | Retention time (Rt) | Relative Rt to betanin | Chemical Formula | Theorical m/z [M+H] | Observed m/z [M+H] | Mass error (ppm) | Fragments | ||
19 | 17-decarboxy-isobetanin | 7.69 | 2.24 | C23H26N2O11 | 507.1609 | 507.1587 | -2.24 | 345.10, 299.10, 194.04,150.05, 106.06 | ||
20 | 15-decarboxy-betanin | 8.29 | 2.42 | C23H26N2O11 | 507.1609 | 507.1574 | -3.57 | 345.10, 299.10, 194.04,150.05, 106.06 | ||
21 | 2-descarboxy-phyllocactin | 9.21 | 2.69 | C26H29N2O14 | 593.1613 | 593.1578 | -3.51 | 345.10, 299.10, 194.04,150.05, 106.06 | ||
22 | 2-descarboxy-isophyllocactin | 9.41 | 2.74 | C26H29N2O14 | 593.1613 | 593.1589 | -2.43 | 345.10, 299.10, 194.04,150.05, 106.06 | ||
23 | 17-decarboxy-neobetanin | 3.62 | 1.06 | C23H24N2O11 | 505.1453 | 505.142 | -3.31 | 343.09, 297.08, 194.04,150.05, 106.06 | ||
24 | Neobetanin, decarboxylated | 6.6 | 1.92 | C23H24N2O11 | 505.1453 | 505.1415 | -3.83 | 343.09, 297.08, 194.04,150.05, 106.06 | ||
25 | 2-decarboxy-neobetanin | 6.71 | 1.96 | C23H24N2O11 | 505.1453 | 505.1421 | -3.24 | 343.09, 297.08, 194.04,150.05, 106.06 | ||
26 | Bidecarboxy-dehydro-betanin | 7.61 | 2.22 | C22H24N2O9 | 461.1555 | 461.1508 | -4.71 | 299.10, 297.08, 194.04,150.05, 106.06 | ||
27 | Neobetanin | 8.31 | 2.42 | C24H24N2O13 | 549.1351 | 549.1306 | -4.50 | 387.07, 297.08, 194.04,150.05, 106.06 | ||
28 | 2-decarboxy-isoneobetanin | 8.39 | 2.45 | C23H24N2O11 | 505.1453 | 505.1404 | -4.90 | 343.09, 297.08, 194.04,150.05, 106.06 | ||
29 | 2–decarboxy-2,3-dehydro–neobetanin | 10.06 | 2.93 | C23H23N2O11 | 503.1296 | 503.1271 | -2.50 | 341.07, 297.08, 194.04,150.05, 106.06 | ||
30 | 2,17-bidecarboxy-2,3-dehydro-neobetanin | 11.14 | 3.25 | C22H22N2O9 | 459.1398 | 459.1354 | -4.41 | 297.08, 269.09, 251.08, 223.08, 195.09 | ||
# | Compound | Retention time (Rt) | Relative Rt to betanin | Chemical Formula | Theorical m/z [M+H] | Observed m/z [M+H] | Mass error (ppm) | Fragments | ||
Betaxanthins | ||||||||||
31 | Histamine-betaxanthin | 9.04 | 2.64 | C14H16N4O4 | 305.1244 | 305.1206 | -3.76 | 287.12, 261.13, 211.07, 194.04, 150.05, 106.06 | ||
32 | Tryptophan-betaxanthin | 10.12 | 2.95 | C20H19N3O6 | 398.1340 | 398.1318 | -2.19 | 269.07, 223.07, 211.07, 194.04, 150.05, 106.06 | ||
33 | Phenylalanine-betaxanthin | 10.15 | 2.96 | C18H18N2O6 | 359.1230 | 359.1199 | -3.15 | 315.13, 313.11, 211.07, 194.04, 150.05, 106.06 | ||
34 | Leucine-betaxanthin (Vulgaxanthin IV) | 10.01 | 2.92 | C15H20N2O6 | 325.1387 | 325.1361 | -2.56 | 281.14, 251.13, 211.07, 194.04, 150.05, 106.06 | ||
35 | Alanine-betaxanthin | 2.02 | 0.59 | C12H14N2O6 | 283.0920 | 283.0887 | -3.30 | 237.08, 211.07, 194.04, 150.05, 106.06 | ||
36 | Proline-betaxanthin (Indicaxanthin) | 3.04 | 0.89 | C14H16N2O6 | 309.1075 | 309.1047 | -2.82 | 265.11, 211.07, 194.04, 150.05, 106.06 | ||
37 | Serine-betaxanthin | 4.77 | 1.39 | C12H14N2O7 | 299.0869 | 299.0831 | -3.84 | 255.09, 253.09, 211.07, 194.04, 150.05, 106.06 | ||
38 | Threonine-betaxanthin | 1.52 | 0.44 | C13H16N2O7 | 313.1022 | 313.1004 | -1.83 | 269.09, 267.09, 211.07, 194.04, 150.05, 106.06 | ||
39 | Glutamine-betaxanthin (Vulgaxanthin I) | 1.20 | 0.35 | C14H17N3O7 | 340.1134 | 340.1102 | -3.20 | 323.08, 277.08, 211.07, 194.04, 150.05, 106.06 | ||
40 | Glycine-betaxanthin (Portulacaxanthin II) | 6.49 | 1.89 | C11H12N2O6 | 269.0756 | 269.0738 | -1.78 | 331.12, 239.11, 211.07, 194.04, 150.05, 106.06 | ||
41 | Methionine-betaxanthin | 7.30 | 2.13 | C14H18N2O6S | 343.095 | 343.0916 | -3.36 | 315.09, 269.09, 211.07, 194.04, 150.05, 106.06 | ||
42 | Tyramine-betaxanthin (Miraxanthin III) | 8.64 | 2.52 | C17H18N2O5 | 331.1281 | 331.1239 | -4.21 | 287.13, 239.11, 211.07, 194.04, 150.05, 106.06 | ||
43 | Dopamine-betaxanthin (Miraxanthin V) | 7.67 | 2.24 | C17H18N2O6 | 347.123 | 347.1213 | -1.75 | 255.11, 211.07, 194.04, 150.05, 106.06 |
To reveal the most abundant betalains in red amaranth, relative quantification was performed since no commercially available analytical standards for betalains were available. Peak areas of each betalain in MS1 mode using the extracted ion chromatograms based on the precursor ion [M+H]+ were measured and log10-transformed (Fig. 5). The heat map highlights the most abundant betacyanins and betaxanthins (the darker the colour, the higher the concentration). The amaranthin with the highest abundance in the red amaranth samples agrees with previous studies [20, 25, 33]. Amaranthin was four times more abundant in the inflorescences than in leaves and was nearly 70 % of the betacyanin composition. Isoamaranthin, which is the epimer of amaranthin, was also detected but is less abundant than amaranthin in both inflorescences and leaves of red amaranth. Amaranthin and its epimer isoamaranthin were reported as abundant betacyanins and widely distributed in thirty-seven species of eight genera of Amaranthus [24]. Among the forty accessions studied by Cai et al. (2001) [24], amaranthin and isoamaranthin were displayed up to 91.5 and 2.8 % in average of the betacyanin composition. The amaranthin-derivatives abundance was higher than that of betanin-derivatives; we found three and ten times more amaranthin-derivatives than betanin-derivatives in inflorescences than in leaves, respectively. This finding agrees with the fact that betacyanin-derivatives were generated spontaneously from betacyanins, thus more abundance of amaranthin than betanin can result in higher abundance of either 17-decarboxy-amaranthin, decarboxy-(iso)amaranthin, and neo-amaranthin than betanin-derivatives. The main betaxanthins in leaves and inflorescences of red amaranth were histamine-betaxanthin, non-polar amino acid-derived betaxanthins (tryptophan-, phenylalanine-, leucine-, alanine-, proline-betaxanthin) and polar amino acid-derived betaxanthins (serine-, threonine-, glutamine-betaxanthin), which form about 90 % of the betaxanthin’s composition. A comparison of individual betaxanthins abundance revealed that there was a higher abundance in leaves than in inflorescences. These findings suggest that both betacyanins and betaxanthins can be produced in red amaranth.

Fig. 5 Relative abundances of main betalains in red amaranth. (A) Heat map of relative abundances calculated in log10 for main betacyanins. (B) Heat map of relative abundances calculated in log10 for main betaxanthins. The colour bar indicates the log10-transformed peak area values.
Given that red amaranth used in this study was red colour in almost, if not all, tissues including stems, leaves, and inflorescences, the betacyanins and betaxanthins detected imply that red amaranth is supported by a betalains biosynthetic pathway. There have been studies focused on improving the production of betalains in plants that have revealed the L-tyrosine use and key genes responsible for the overaccumulation of betacyanins and betaxanthins [34, 35]. Betalains are synthesized from L-tyrosine that is hydroxylated to L-DOPA by a group of redundant tyrosine hydroxylases (CYP76AD1/5/6/15). L-DOPA is further converted to 4,5-seco-DOPA by L-DOPA 4,5-dioxygenase (DODA), and then to betalamic acid after spontaneous intramolecular condensation occurs in the unstable 4,5-seco-DOPA [35, 36]. L-DOPA can be alternatively oxidized to cyclo-DOPA by CYP76AD1/3. When the betalamic acid is conjugated with cyclo-DOPA, the red-violet betacyanins are generated, whereas the conjugation with imino or amino group of amino acids generates yellow betaxanthins. Wang and co-workers (2017) [34], using red beat varieties that produce high yield of betacyanins and yellow beat varieties that produce high yields of betaxanthins, have found that accumulation of betacyanins needs high concentration of L-tyrosine whereas betaxanthins requires lowering to convert L-DOPA to cyclo-DOPA. As most research on amaranth has been focused on nutrient values, our LC-MS analysis of the leaves and inflorescences allowed the characterization of the 43 betalains in red amaranth. This data expands the information for betalain content in A. cruentus got with spectrophotometric techniques [18]. New products and technologies based on the use of A. cruentus have been generated recently [37, 38].
Conclusions
In the present study, we tried to valorize the red amaranth (A. cruentus L.) as betalain pigments source and develop the LC-MS platform to monitor the composition of betalains. Consequently, betalains from the leaves and inflorescences were identified and analyzed by LC-MS to determine the composition of betalains. Our findings define the main betalains in the leaves and inflorescences of red amaranth (A. cruentus L.), consisting of six amaranthin-type, two gomphrenin-type, 22 betanin-type, and 13 betaxanthins. LC coupled with high-resolution mass spectrometry allowed to identify the betalains with mass measurement errors of less than 5 ppm. Betacyanins-derivatives were included in the identified betalains. The wide variety of betalins content underlines the importance of using leaves and inflorescences as sources of colourants. The composition of betalains in red amaranth can allow the future characterization of these colourants. This study served as a platform to monitoring the composition of betalains useful for revealing their impact in key biological and pharmacological activities