Introduction
Vegetable oils are of particular interest in Brazil, as the country is one of the major producers of this type of oil [1]. Juice process by-products including seeds are used to obtain essential and fixed oils of considerable added value and high economic potential. Food waste biorefineries have been little explored as sources of bioactive compounds for the production of products of industrial interest as biopesticides, bioplastics, drugs, food supplements and colorants [2].
Previous studies have shown that seed oils can be used as an alternative source to traditional antimicrobial agents [3]. By-products of fruit juice processing, including seeds used to obtain essential and fixed oils, have added value and high economic potential [4]. The extraction yields of oils obtained from seeds vary significantly, however, the chemical composition of seed oils basically consists of triglycerides and free fatty acids [5]. The most common fatty acids found in seed oil are palmitic, palmitoleic, stearic, oleic, linoleic and linolenic acids [6]. Free fatty acids exhibit a variety of biological activities, with the ability to kill or inhibit the growth of microorganisms being the most potent activity exhibited. [7].
The aim of the present study was to characterize the chemical components of oils extracted from the seeds of tropical fruits (Annona squamosa, Annona muricata, Annona atemoya, Carica papaya L., Cucumis melo, Cucurbita moschata, Citrus reticulata, Eugenia uniflora, Malpighia emarginata and Citrullus lanatus) using GC-MS and evaluate the antimicrobial activity of the oils based on the minimum inhibitory concentration.
Material and methods
Oil extraction
The fresh fruits of A. muricata (soursop), A. squamosa (sugar apple), A. atemoya (atemoya), C. papaya (papaya), C. melo (melon), C. moschata (crookneck pumpkin), C. reticulata (mandarin orange), C. lanatus (watermelon), E. uniflora (surinam cherry) and M. emarginata (acerola cherry) were purchased commercially and the seeds manually separated. Seeds were dehydrated at 50 °C for 24 h, ground in a knife mill with a screen (3 mm) and stored at 18 °C until use. The extraction of the oil from the ground seeds was performed using a Soxhlet extractor with n-hexane as the solvent by 6h to 50 ºC [8]. The solvent was evaporated from the remaining product using a rotary evaporator at 50 °C (condenser at 10 °C). The oil was stored sheltered from light and air at a temperature of 18 °C.
Transesterification reaction
Esters were obtained by transesterification reaction performed in reflux using a molar ratio of 6:1:0.1 of methanol/lipid/catalyst under agitation as described previously [9].
Characterization of methyl esters
Oil samples of 1mg.mL-1 were analyzed using GC-MS in the Clarus 580 chromatograph (PerkinElmer) coupled to the Clarus SQ8S mass spectrometer with an elite-5MS column (30 m x 0.25 mm x 0.25 µm) and split flow injection system. The injection volume of the sample was 1 µL. The injector temperature was 250 °C, with helium gas flow of 1 mL.min-1. The initial temperature of the column was 100 °C. After the injection of the sample, the temperature was increased at a rate of 10 °C min-1 until reaching 290 °C, which was maintained for 10 min. Methyl esters were identified using the NIST 2.2 spectrum library, with the comparison of peak retention times to a standard mixture of methyl esters (C4 - C24) of the respective fatty acids. The standard was acquired from Sigma Aldrich. The areas of the peaks in the chromatograms were integrated to obtain the total ionic current signal, which was used to determine the relative percentages of fatty acids in each sample.
In vitro antimicrobial activity assay
The antimicrobial potential of the oils obtained from the fruit seeds was tested against the bacteria Staphylococcus aureus (UFPEDA 02), Pseudomonas aeruginosa (UFPEDA 416), Klebsiella pneumoniae (UFPEDA 396), Enterococcus faecalis (UFPEDA 138) as well as the fungus Microsporum gypseum (UFPEDA 2565) and yeast Candida utilis (UFPEDA 1009). The microorganisms were kept in nutrient agar and stored at 4 °C. The evaluation of antimicrobial activity was performed with the determination of minimum inhibitory concentration (MIC), as described elsewhere [10,11]. The antimicrobials metronidazole (10 µg mL-1), gentamicin (20 µg mL-1) and ketoconazole (50 µg mL-1) were used as positive controls.
Statistical analyses
Based on the results of the GC-MS analysis of the fatty acids in the oils from the seeds of the species A. squamosa, A. muricata, A. atemoya, C. papaya L., C. melo, C. moschata, C. reticulata, E. uniflora, M emarginata and C. lanatus, a principal component analysis (PCA) graph was generated from the dataset used to evaluate the composition of the seed oils and percentages of fatty acids. GC-MS data were exported in ASCII format to Microsoft Excel to produce a sample data matrix versus percentage peaks of the fatty acids with the associated areas of the peaks. All analyses were performed with the aid of Unscrambler® version 9.5 [12].
Results and discussion
The hexane extracts of the seed oils varied little in appearance with a color ranging from light to dark yellow and a consistency ranging from liquid to pasty at room temperature. The exception was the E. uniflora seed oil, which had a dark green color. Extraction was performed in triplicate and yields ranged from 0.53 to 35.10 % (Table I). The lowest yield was found for the seeds from E. uniflora and the highest yield was found for the seeds from C. reticulata.
Table 1 Mass values for yields of oils extracted from tropical fruit seeds.
Fruit species | Fresh seeds (g) | Dry seeds (g) | Oils (g) | Yields (%) |
---|---|---|---|---|
A. muricata | 10 | 5.62 | 1.18 ± 0.08 | 20.92 |
A. squamosa | 10 | 7.08 | 1.08 ± 0.03 | 15.22 |
A. atemoya | 10 | 5.95 | 1.91 ± 0.01 | 32.02 |
C. papaya | 10 | 5.70 | 0.99 ± 0.02 | 17.36 |
C. melo | 10 | 7.07 | 1.74 ± 0.02 | 24.62 |
C. moschata | 10 | 8.26 | 2.07 ± 0.03 | 25.07 |
C. reticulata | 10 | 6.02 | 2.11 ± 0.06 | 35.10 |
C. lanatus | 10 | 6.16 | 1.09 ± 0.10 | 17.75 |
E. uniflora | 10 | 5.11 | 0.27 ± 1.33 | 5.28 |
M. emarginata | 10 | 2.37 | 0.52 ± 0.04 | 21.94 |
The yield from the E. uniflora seeds was similar to that reported by Santos and collaborators, who described a yield ranging from 0.16 to 0.48 % [13]. Anwar et al., [14] reported a yield ranging from 32.4 to 36.4 % for C. reticulata seeds, which is similar to the yield found in the present investigation (35.10 %). A good yield was also achieved with A. atemoya seeds (32.02 %), which is higher than the percentage described by Da Cruz et al. [15] (25.92 %). The crude fat content extracted from the C. melo seed oil (24.62 %) was lower than the yield of 31.68 % reported by Raji and Orelaja [16]. The greatest divergence in yield was found for C. lanatus (17.75 %), which was much lower than the percentage of 57.26 % described by Essien and Eduok [17].
All oils were submitted the transesterification reaction and showed a dark yellow color with the exception of the esterified oil of the seeds from E. uniflora, which had a dark green color. Mass after the transesterification reaction ranged from 0.134 g to 0.412 g (Table 2).
Table 2 Mass of oils obtained after transesterification reaction.
Fruit species | Mass (g) |
---|---|
A. muricata | 0.202 |
A. squamosa | 0.265 |
A. atemoya | 0.356 |
C. papaya | 0.196 |
C. melo | 0.187 |
C. moschata | 0.412 |
C. reticulata | 0.209 |
C. lanatus | 0.261 |
E. uniflora | 0.134 |
M. emarginata | 0.210 |
Based on the GC-MS analysis it was possible to identify ten fatty acids including six saturated (myristic, palmitic, stearic, arachidic, gondoic and lignoceric acids) and four unsaturated (palmitoleic, oleic, linoleic, and linolenic acids) (Table 3). Palmitic, stearic, oleic, and linoleic acids were found in all samples analyzed. Six fatty acids were identified as major constituents of A. muricata seed oil, with 32.28 % saturation and 68.71 % unsaturation of methyl esters, values similar to the reported by Pinto et al.,[18] (26.1 % saturated acids and 76.72 % unsaturated acids). Oleic acid was the major constituent of the A. muricata seed oil, accounting for 37.53 ± 1.27 %. In A. squamosa seed oil were identified six fatty acids accounting for 98.07 % of the fatty acids. The proportion of saturation was 36.15 %, whereas the proportion of unsaturation was 61.92 % of methyl esters. A higher proportion of unsaturation (70.3 %) and lower proportion of saturation (28 %) were found by Rana [19]. Oleic acid was the major constituent, accounting for 39.71 ± 0.01 %.
Table 3 Free fatty acid composition (%) in seed oil.
Fatty acids | Oil samples | |||||||||
A. muricata | A. squamosa | A. atemoya | C. papaya | C. melo | C. moschata | C. reticulata | C. lanatus | E. uniflora | M. emarginata | |
Myristic (C 14:0) | - | - | - | 0.20 ± 0.01 | - | - | 0.41 ± 0.01 | - | 1.14 ± 0.08 | 0.53 ± 0.00 |
Palmitic (C 16:0) | 24.37 ± 0.09 | 18.43 ± 1.20 | 15.47 ± 1.74 | 20.41 ± 1.00 | 17.53 ± 1.63 | 15.34 ± 0.04 | 23.46 ± 1.45 | 14.12 ± 1.39 | 28.61 ± 2.81 | 21.07 ± 2.79 |
Palmitoleic (C 16:1) | 1.73 ± 0.02 | - | 0.92 ± 0.00 | 0.23 ± 0.00 | - | - | 2.74 ± 0.02 | - | 3.92 ± 0.00 | 1.00 ± 0.01 |
Stearic (C 18:0) | 6.91 ± 0.01 | 15.40 ± 0.08 | 17.78 ± 0.76 | 3.35 ± 0.04 | 6.45 ± 0.01 | 10.31 ± 0.15 | 7.22 ± 0.00 | 6.95 ± 0.05 | 4.10 ± 0.00 | 13.72 ± 1.23 |
Oleic (C 18:1) | 37.53 ± 1.27 | 39.71 ± 0.01 | 44.95 ± 2.10 | 70,33 ± 2.03 | 14.36 ± 1.90 | 40.89 ± 1.78 | 21.86 ± 1.69 | 18.81 ± 1.11 | 18.86 ± 1.80 | 29.42 ± 1.99 |
Linoleic (C 18:2) | 27.91 ± 1.12 | 21.31 ± 0.05 | 21.50 ± 1.30 | 4.31 ± 0.00 | 59.60 ± 3.21 | 29.28 ± 2.31 | 39.03 ± 2.12 | 57.35 ± 3.76 | 29.08 ± 2.56 | 30.70 ± 0.03 |
Linolenic (C 18:3) | 0.01 ± 0.00 | - | - | - | - | 0.44 ± 0.00 | 0.69 ± 0.00 | 0.83 ± 0.00 | 2.67 ± 0.01 | - |
Arachidic (C 20:0) | 1.52 ± 0.04 | 2.32 ± 0.00 | 0,19 ± 0,00 | 0.87 ± .0.20 | 0.99 ± 0.01 | 1.22 ± 0.02 | 1.56 ± 0.02 | 0.59 ± 0.00 | 2.70 ± 0.02 | 2.73 ± 0.00 |
Gondoic (C 22:0) | - | - | - | - | - | - | 0.91 ± 0.06 | - | - | - |
Lignoceric (C 24:0) | - | - | - | - | - | - | 1.24 ± 0.01 | - | 1.46 ± 0.00 | 0.79 ± 0.02 |
∑ Saturated | 32.80 | 36.15 | 33.44 | 25.54 | 24.97 | 26.87 | 34.80 | 21.66 | 38.01 | 38.84 |
∑ Unsaturated | 67.18 | 61.02 | 66.56 | 74.87 | 73.96 | 70.61 | 64.32 | 76.99 | 54.53 | 61.12 |
Palmitic (15.47 ± 1.74 %), stearic (17.78 ± 0.76 %), linoleic (21.5 ± 1.30 %) and oleic (44.95 ± 2.10 %) acids were the major constituents in A. atemoya seed oil. Previous study showed proportions of 13.4 % palmitic acid, 14.5 % stearic acid and 46.80 % oleic acid for A. atemoya seed oil [15].
Chemical profile of the C. papaya seed oil revealed seven fatty acids accounting for 99.7 % of the oil. A large quantity of oleic acid was found (70.33 ± 2.03 %), followed by palmitic acid (20.41 ± 1.00 %). A previous study of C. papaya seed oil also found a high percentage of oleic acid (72.5 %) followed by palmitic acid (13.5 %) [20]. Major constituent of the C. melo seed oil was linoleic acid, accounting for 59.60 ± 3.21 % of the oil total.
Nine compounds were found in the esterified oil from seeds of C. reticulata. The major constituents were palmitic (23.46 ± 1.45 %), linoleic (39.03 ± 2.12 %) and oleic (21.86 ± 1.69 %) acids. Similar results were reported by Santos et al., [13], who found 25.79 % palmitic acid, 39.55 % linoleic acid and 24.05 % oleic acid.
In C. moschata seed oil was identified seven fatty and as major saturated compounds palmitic (15.34 ± 0.04 %) and stearic acids (10.31 ± 0.15 %), while the oleic acid (40.89 ± 1.78 %) and linoleic acids as major unsaturated compounds (29.28 ± 2.31 %). Applequist et al., [21] reported different results regarding unsaturated acids, with a lower proportion of oleic acid (25.78 %) and with linoleic acid as the major constituent (53.53 %).
The chemical profile of the E. uniflora seed oil had palmitic acid as the major saturated compound (28.61 ± 2.81 %), whereas linoleic acid (29.08 ± 2.56 %) and oleic acid (18.86 ± 2.56 %) were the major unsaturated compounds. Interestingly, α-selinene accounted for 7.41% of the oil. This compound has not been previously reported in the seed oil. α-Selinene has been found in the essential oil of the fruit [22].
Eight fatty acids were identified in the M. emarginata seed oil: saturated acids accounted for 38.84 % of the oil, among which the major constituents were palmitic acid (21.07 ± 2.79 %) and stearic acid (13.72 ± 1.23 %). Unsaturated compounds accounted for approximately 61.1 % of the oil, the major constituents of which were linoleic acid (30.70 ± 0.03 %), followed by oleic acid (29.42 ± 1.99 %). A similar proportion has been previously reported for saturated and unsaturated acids, such as 21.80 % palmitic, 13.9 % stearic, 31.90 % oleic and 29.20 % linoleic acids [23]. Here, arachidic acid was found by first times in the M. emarginata seed oil.
The four saturated fatty acids accounted for 22.45 % were identified in the C. lanatus seed oil including as major constituents palmitic (14.12 ± 1.39 %) and stearic (6.95 ± 0.05 %) acids. The major constituent of the unsaturated component was linoleic acid (57.35 %), followed by oleic acid (18.81 %). The compounds and percentages were similar to those reported in a previous study [17].
All seed oils tested exhibited antimicrobial activity (Table 4). MIC values ≤ 500 μg/ml were considered strong inhibitors; MIC values from 600 to 1500 µg/ml were considered moderate inhibitors; values ˃ 1500 μg/ml were considered weak inhibitors [24,25].
Table 4 Minimum inhibitory concentration at μg mL-1 of seed oils.
Oil Samples Positive control | Gram-positive bacteria | Gram-negative bacteria | Yeast | Fungus | ||
S. aureus | E. faecalis | P. aeruginosa | K. pneumoniae | C. utilis | M. gypseum | |
E. uniflora | 39.0 | 39.0 | 1250 | 39.0 | 312.5 | 1250 |
C. moschata | 2500 | 2500 | 2500 | 2500 | 1250 | 2500 |
A. squamosa | 2500 | 2500 | 2500 | 2500 | 1250 | 2500 |
C. reticulata | 2500 | 2500 | 2500 | 2500 | 1250 | 2500 |
A. muricata | 2500 | 2500 | 2500 | 1250 | 2500 | 2500 |
C. papaya | 2500 | 2500 | 2500 | 2500 | 2500 | 2500 |
C. anatus | 2500 | 2500 | 2500 | 2500 | 1250 | >2500 |
C. melo | 2500 | 2500 | 2500 | 2500 | 250 | >2500 |
M. emarginata | 1250 | 1250 | 1250 | 1250 | 625 | 1250 |
A. atemoya | 2500 | 2500 | 2500 | 1250 | 2500 | 2500 |
Metronidazole | 19.5 | 19.5 | 635 | 19.5 | - | - |
Gentamicin | 4.8 | 4.8 | 4.8 | 4.8 | - | - |
Ketoconazole | - | - | - | - | 2500 | 2500 |
For the bacteria, the fixed oil extracted from the E. uniflora seeds showed best antimicrobial activity, with MICs ranging from 39.0 to 1250 μg mL-1. The lowest MICs were found for the bacteria S. aureus, E. faecalis and K. pneumoniae, indicating that the E. uniflora seed oil has strong antimicrobial activity toward these bacteria, whereas as moderate antimicrobial activity was found for the strain of P. aeruginosa, with an MIC of 1250 μg mL-1. No reports were found in the literature on the antimicrobial activity of oil extracted from E. uniflora seeds.
The M. emarginata seed oil had moderate inhibitory activity toward all bacteria tested (MIC = 1250 μg mL-1). The A. muricata and A. atemoya seed oils had moderate inhibitory activity toward the bacterium K. pneumoniae (MIC = 1250 μg mL-1). The A. atemoya seed oil had weak inhibitory activity toward the other bacteria tested. Moreover, the other oils analyzed had weak inhibitory activity toward all bacteria tested (MIC = 2500 μg mL-1).
Regarding the fungus and yeast, the results of the oils ranged from inactivity to strong inhibitory activity. The oil extracted from E. uniflora seeds had strong antimicrobial activity toward C. utilis (MIC = 312.5 μg.mL-1) and moderate activity toward M. gypseum (MIC = 1250 μg mL-1). These MIC values were lower than those of the positive control for both fungi. No reports were found in the literature on the inhibitory activity of E. uniflora seed oil toward these fungi.
The fixed oil from M. emarginata seeds exhibited moderate inhibitory activity, with an MIC of 625 μg.mL-1 for C. utilis and 1250 μg mL-1 for M. gypseum. These MICs were lower than the values found for the positive controls for both microorganisms. As occurred with E. uniflora, no data were found in the literature on the antimicrobial activity of the oil from M. emarginata seeds. These two oils could serve as options for the control of dermatophytosis caused by C. utilis and M. gypseum. The fixed oils from the seeds of C. moschata, A. squamosa, C. reticulata and C. melo exhibited moderate antimicrobial activity toward C. utilis with MIC 1250 μg mL-1. The fixed oils from E. uniflora and M. emarginata seed, the other oils had either weak inhibition or were inactive against the fungus M. gypseum.
The esterified seed oils studied had MICs higher than 2500 μg mL-1 for all microorganisms, indicating a lack of antimicrobial activity when esterified. This suggests that long-chain fatty acids are responsible for the antimicrobial activity, as described in the literature [26]. Carboxyl groups are found in the chemical structures of the main commercially available antibiotics, including those of a natural and synthetic origin, such as penicillin and ciprofloxacin [27]. The action mechanism of free fatty acids against bacteria remains unclear elucidated, but the prime target seems to be the bacterial cell membrane and the various essential processes that occur within and at the membrane. Damage to bacteria caused by free fatty acids may be associated with the detergent properties of these compounds due to their amphipathic structure. This property allows free fatty acids to interact with the cell membrane to generate momentary or permanent pores of variable size. At higher concentrations, detergents such as free fatty acids can solubilize the membrane to such an extent that multiple membrane proteins or larger sections of the lipid bilayer are released [28-29].
PCA (Fig. 1) grouped the oil samples based on the composition of fatty acids. The species E. uniflora and C. reticulata were clearly separated from the other samples. No significant differences were found in the lipid profile of the oils beyond variations in the percentages of the acids.
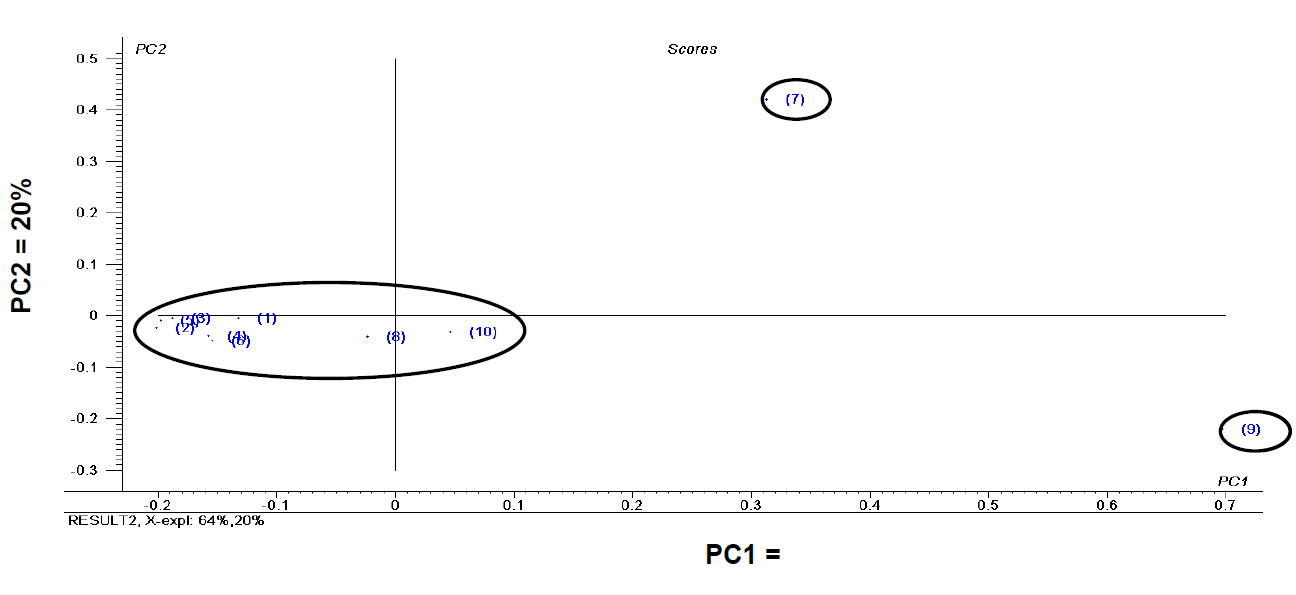
Fig. 1 Principal component analysis of fixed oils extracted from seeds of species analyzed. (1) A. muricata; (2) A. squamosa; (3) A. atemoya; (4) C. papaya; (5) C. melo; (6) C. moschata; (7) C. reticulata; (8) C. lanatus; (9) E. uniflora; (10) M. emarginata.
The first and second components of the PCA explained 84 % of the variability in the data (PC1 = 64 % and PC2 = 20 %). The separation of the samples may be explained by the presence of lignoceric acid (C24:0) in only the seed oils from C. reticulata (1.24 % ± 0.01) and E. uniflora (1.46 % ± 0.00). The separation of C. reticulata may also be explained by the fact that this species had the only seed oil with gondoic acid (C22:0) (0.91 % ± 0.06). The separation of E. uniflora may be explained by the higher proportions of myristic acid (C14:0) (1.14 % ± 0.08 %) and linoleic acid (C18:3) (2.70 % ± 0.02) compared to the other samples.
Conclusion
This study report of the extraction, identification of the chemical composition and evaluation of antimicrobial activity of oils from the seeds of tropical fruits of the species Annona squamosa, Annona muricata, Annona atemoya, Carica papaya L., Cucumis melo, Cucurbita moschata, Citrus reticulata, Eugenia uniflora, Malpighia emarginata and Citrullus lanatus. Saturated (palmitic and stearic) and unsaturated (oleic and linoleic) fatty acids were found in the oils as major compounds.
Considering that seeds are usually discarded by the fruit juice processing industry, generating industrial waste, our results show that the seeds of 10 different types of tropical fruits can be used in the development of antimicrobial products and consequently minimize the environmental contamination generated by food industry.