Introduction
Pharmaceutical drug with poor biopharmaceutical presentation is the main trouble in developing and designing therapeutic measures.[1] The development of the solid medication prescription process is hindered by their low solubility, dissolution, bioavailability and poor physicochemical properties.[2] Synthesis of co- crystal is an innovative and auspicious technique to enhance and improve the properties of Active pharmaceutical ingredients (APIs). Co-crystal is a solids-state form of crystalline single-stage materials made of at least two or more different molecular or ionic compounds commonly in a stoichiometric proportion that are neither solvated nor simple salts. [3,4] The comprehensive definition of co-crystals consists of two or more components that form a unique crystalline form structure, which has extraordinary properties. The design and synthesis of Crystal engineering is the plan and combination of solid-state structures, because of the agreement and utilization of intermolecular interaction, with required properties. [5,6] The principal techniques utilized for crystal engineering design depend on hydrogen bonding. These can be perceived with critical ideas, for example, supramolecular synthons and secondary structure units. [7,8] The co-crystals of the drug are gaining much interest from the research experts because of their capability to modify APIs' physicochemical, mechanical, and pharmacokinetic properties without positioning therapeutic effect. The formulation of pharmaceutical co-crystal could be used as a game-challenging method for the administration of the oral drug in a new crystalline form. co-crystals' diverse, innovative application is analogous to taste concealment, low toxicity, protecting prospect, drug delivery and therapeutic effect of the drug, and commercial application [5,9].
Puerarin (PUE) is a primary active ingredient in Chinese traditional medicine (CTM) that originates from the kudzu root known as Gegen.[10] PUE exhibits poor solubility, leading to low oral bioavailability. According to the biopharmaceutics classification system (BCS), PUE can be categorized as IV class due to its low solubility and limited intestinal permeability. [11-13] This research aims to restate the design and synthesis of co-crystal and their applications in the field of APIs, essentially on enhancements of API physicochemical properties by the formulation of co-crystals. Significant progress has been made during this research work and the development of co-crystals in the pharmaceutical field. The synthesis of co-crystal is a successful strategy for developing the solubility and dissolution rate of APIs of PUE by formulation of its co-crystal with PG. Additionally, co-crystal reveals multifaceted assemblies that can influence APIs' physical and chemical properties with excellent clinical performance and maybe remaining stability during processing and storage.
Material and Experimental
Material and chemical
Puerarin C21H20O9 (PUE) was purchased from Jingxi Pharmaceutical Co, Ltd. L-Pyroglutamic acid C5H7NO3 (PG) were purchased from Aladdin. Both PUE and PG with 99 % purity were at first characterized by X-ray powder diffraction (PXRD). All the other chemicals were of analytical grades.
Synthesis of PUE-PG co-crystal
The co-crystal synthesized by recrystallization method by mixing equal 1:1 molar ratio. Both PUE and PG in the equal molar ration (0.5mM) were added to 70% EtOH Solvent. The solution was stirred, heated at 55 °C for 3 hours. The resulting solution was cool to room temperature and fine rod type crystalline form co-crystal was obtained.
Solid-state Characterization
The Powder diffraction designs were measured on a Rigaku D/Max-2550PC diffractometer employing a pivoting anode Cu-target X-ray (λ= 1.5406 Å) generator worked at 40 kV and 250 mA. The rate Cu Ka X-ray bar was mono chromatized by a vertical evaluated multiplayer reflects to expel the Kβ radiation and get a paralleled pillar with a width of 0.8 mm. The scans were run from 3.0 to 40.0° 2θ, with an expanding step measure of 0.02° and a count time of 0.5 ~ 1 s. About 4-7 mg powder of the sample was used for differential scanning calorimetry (DSC) investigations. The DSC investigation was performed on a TA DSCQ100 differential filtering calorimeter at the heating rate of 10 °C /min beneath a nitrogen stream of 50 cm3 min-1. A temperature extension of 35-220 °C was checked. The DSC temperature pivot was calibrated in an aluminum container with a standard indium test (softening point 156.6 °C, warm of combination 28.45 J g-1). The sample was equilibrated at 25 °C and warmed to 300 °C at a warming rate of 10 °C min-1 beneath a 50 cm3 min-1 nitrogen stream. The calibrated temperature precision was ±0.02 °C, and the calorimetric accuracy for the warm of the combination was ±0.11 %. Before the experiment, about 2 mg samples were blended into 100 mg KBr and the samples were crushed into fine powder. Infrared spectra were recorded on the VECTOR-22 Fourier Infrared Spectrometer within the spectral range of 4000-400 cm-1 in the KBr diffuse reflectance mode. Record data was analyzed utilizing Spectrum software.
Solubility and dissolution studies
Solubility studies of PUE and co-crystal were measured by UV-vis spectrometer in pure water, pH1.2 and pH 6.8 at 37°C and the concentration were calculated employing a standard curve shown in Fig. 3, which was made by measuring the absorbance of different concentrations at their ƛ max. An excess amount of the sample was added to 6 mL of distilled water medium, pH 1.2 acidic and pH 6.8 medium. The supersaturated solution was stirred at 350 RPM employing an magnetic stirrer at 37 °C for 24 hours and the suspension was sieved through Whatman’s 0.45 mm syringe channel. The sifted aliquots diluted sufficiently, and the absorbance was measured at their ƛmax. For the dissolution rate, the sample was particularly poured into 200 mL of pure water, pH 1.2 and pH 6.8 medium and was starrier with 150 RPM for 150 min at 37 °C. At regular interims, 3 mL of the dissolution medium was pulled back and replaced with the volume of the new medium to preserve a steady volume. Each solution expelled measured the absorbance at ƛ max. [14,15]
Result and Discussion
co-crystal synthesis is the design based on understanding and using inter-molecular interactions with desired properties of molecular solid-state assemblies. The primary strategy currently used for co-crystal design is based on hydrogen bonding that can be understood with essential ideas such as supramolecular synthons.[16] The main progress in this obverse emphasis is on designing pharmaceutical co-crystals. Pharmaceutical co- crystals are usually composed of an API (active pharmaceutical ingredient) that is considered safe according to the guidelines given by WHO (World Health Organization). With biocompatible co-former consists of both hydrogen-bonding donor and hydrogen bonding acceptor site, the ability of co-crystallization and well soluble of water. The various properties of an API (such as solubility, dissolution rate, bioavailability, permeability) could successfully be modified by forming pharmaceutical co-crystals. [17,18]. In this work, we successfully enhanced the physicochemical properties of PUE, such as solubility and time-dependent dissolution rate, via recrystallization technique by producing a novel crystalline phase co-crystal with bio-compactable co-former PG.
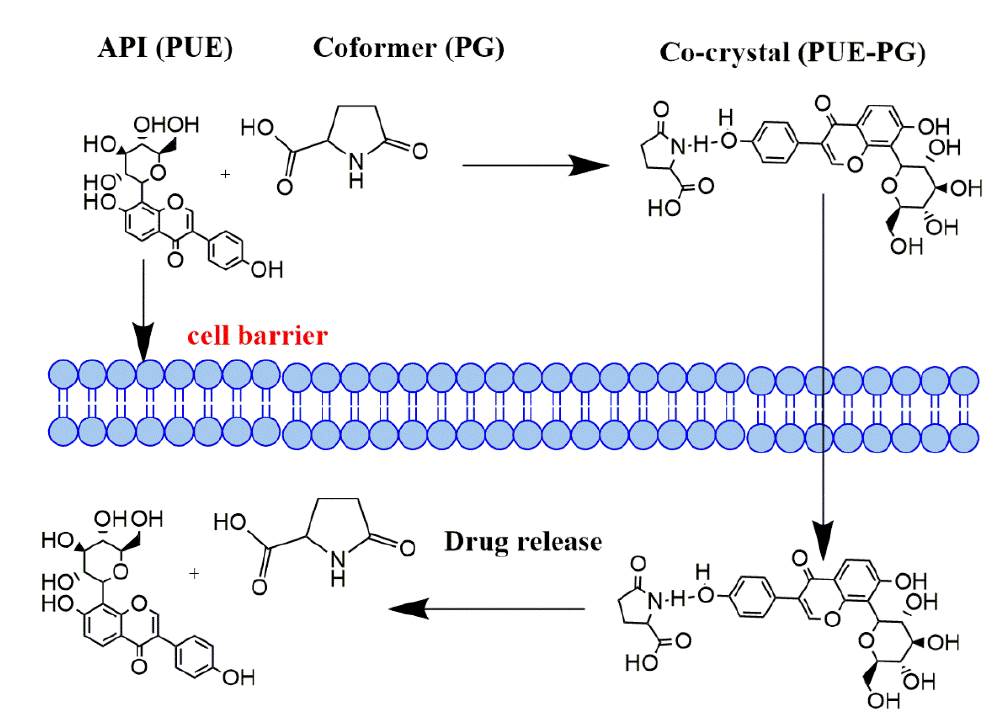
Fig. 4 Schematic diagrams showing the synthesis of co-crystal in which API and co-former mixed in a stochiometric ratio with enhanced physicochemical properties.
Powder X-ray Diffraction (PXRD) analysis
Powder X-ray diffraction (PXRD) analysis is considered a fingerprint technique for determining the crystallinity phase of crystalline material. Each newly crystalline material possesses unique diffractive peaks from its atomic plane.[19] The PUE-PG co-crystal exhibits a new peak at 2θ values of 4.4, 7.3, 9.8, 10.7, 12.0, 15.2, 19.8, 20.3 and 23.5. The position and intensity of the co-crystal PXRD pattern are different when compared with the pure form of PUE and PG as shown in Fig. 5. Changes in the intensity and position of peak indicate the formulation of a new crystalline form of co-crystal.
Differential Scanning Calorimeter (DSC) Analysis
The Differential Scanning Calorimeter (DSC) is also used as an important solid-characterization tool to determine newly synthesized material. Change in the thermal behavior indicates the formulation of new phase material. Fig. 6 represents the endothermal comparison of PUE, PG and co-crystal. Pure PUE exhibits two broad peaks at 110.6 °C and 219.5 °C, while PG exabit a single sharp endothermal peak at 160.7 °C. The PUE- PG co-crystal showed two broad peaks at 99.8 °C and 194.2 °C. The change in co-crystal's endothermal peak from starting material indicates the successful synthesis of co-crystal.
Fourier Transform Infrared Spectroscopy (FT-IR) Spectroscopy Analysis
The Change in the vibrational frequencies of the functional group due to the synthesis of new crystalline form co-crystal could be easily determine by FT-IR analysis.[20] Co-crystal synthesis is based on H-bonding interaction between the functional group of API molecule with co-former, leading to shift in the frequency of the functional group. The PUE consists of a medium peak at 3370cm-1 corresponding for OH, while PG consists of a strong and broad peak at 3300 cm-1 corresponding for OH. In co-crystal, the peak for the -OH functional group slightly changed the frequency and showed a medium peak at 3410 cm-1. Peak observed for -NH functional group in PG at 1670 cm-1, while in the FT-IR spectra of co-crystal the frequency seems to change and observed at 1600 cm-1. all the shift in the frequency is due to the close H-bonding interaction between the functional group of PUE and PG, confirming co-crystal synthesis as shown in Fig. 7.
Molecular Docking
The ability of well refined structure determination of co-crystal is an important tool for drug discovery. It is important to grow suitable single crystal for Single crystal x-ray to analyze crystal structure, however docking still predicts the possible conformation of co-crystal. The proposed and probable structure of PUE-PG Co-crystal is simulated using Auto Dock software, further the data was imported to MURCURY software and generated the probable structure of PUE-PG (Fig. 8(b)). As show in figure, the Co-crystal consist of One molecule of PUE and one molecule of PG in crystal lattice and are connected via H-bonding. Additionally, the co-crystal has great affinity towards protein with low energy (-8.7 kcal), more negative values indicate higher binding affinity as shown in Fig 8(c).

Fig. 8 Represents (a) possible H-bonding interaction between PUE and PG lead to formation of co-crystal, (b) the Probable ORTEP structure with Ball and stick and space filling of co-crystal simulated via Auto Dock and (c) the binding affinity of small molecule legend (co-crystal) with protein with low energy.
Solubility and dissolution
It is well-established that the solid form of APIs has extensive influence on the solubility and dissolution rate of the drug. Consequently, it is vital to choose a suitable API solid form for successful drug expansion. [21,22] According to the biopharmaceutics classification system (BCS), PUE is categorized as a class IV drug, meaning low solubility and low intestinal permeability. Recently the solubility of PUE was effectively enhanced by preparing its co-crystal with L-Proline [23]. The solubility of PUE also enhanced significantly by PG co-former by formulation of its co-crystal. The comprehensive solubility of PUE and co- crystal Water, pH 6.8 and pH 1.2, phosphate is depicted in Fig. 9. co-crystal reveals remarkable enhance solubility in all medium compared to pure form of PUE drug. In all mediums, the time-dependent in vitro dissolution rate is higher than the pure PUE drug. The solubility and dissolution may be due to H-bonding interaction, which leads to improving the solubility of PUE via co-crystallization with PG.
Table 1 Represents the solubility (mg/mL) comparison of Pure drug PUE, previously synthesized co-crystal and new crystalline phase PUE-PG co-crystal.
Compound | pH 1.2 | pH 6.8 | Water |
PUE | 1.5 | 2.3 | 2.8 |
PUE-PRO | 3.9 | 4.6 | 5.8 |
PUE-PG | 4.5 | 5.2 | 5.5 |
Conclusions
A new crystalline phase co-crystal of PUE with biocompatible co-former PG has been successfully synthesized. The solubility and dissolution data suggested that the obtained co-crystal exhibited marginal enhancement of the physicochemical properties compared to the pristine PUE, probably due to its rich hydrogen bonding facilitating the quick diffusion of solution media. Using the biocompatible and non-toxic natural product as a co-delivery reagent may point to new directions in pharmaceutical co-crystallization to product drug formulations with advanced functions beyond merely increasing the solubility and dissolution rate, such as the combination of imaging and therapeutic procedures. Hence co-crystal formulation could be using a novel approach for therapeutic with amended effect.