Introduction
Vitamin E is a group of phytochemicals comprising two major classes, tocopherols and tocotrienols, which share a common chroman-6-ol ring bearing a geranylgeranyl side chain in tocotrienols, and a fully saturated polypropenyl side chain in tocopherols [1]. Both are naturally occurring metabolites found in fruits, vegetables, nuts and edible oils, with tocotrienols having a more restricted distribution [2-3]. All vitamin E isoforms are potent antioxidants and exhibit anti-inflammatory effects. Mechanistic studies have demonstrated that the anti-inflammatory activity in in vitro and in vivo assays occurs through inhibition of ciclooxygenase-2 and 5-lipoxygenase [4], and suppression of signaling pathways of inflammatory mediators NF-kb and JAK-STAT6 and JAK-STAT3 [5]. Currently, inflammatory-related diseases are recognized as a major cause of morbidity in humans; since the inflammatory process can contribute to the development of more severe health problems such as cancer [5], development of anti-inflammatory drugs is an important subject for medicinal chemistry.
Chalcones are a group of secondary metabolites found in fruits and vegetables, characterized by a propenone core linking two phenyl rings [6]. Because of their diverse biological activities, which include antimicrobial, anticancer, antitumor, antioxidant, antihyperglycemic and anti-inflammatory, the chalcone skeleton is considered a privileged scaffold with potential in medicinal chemistry [7]. Recently, prenylated chalcones, and specifically pyranochalcones, have been investigated for their pharmacological properties, particularly a potent anti-inflammatory activity [8]. In view of this, the combination of two well-known precursors known for their anti-inflammatory activity, i.e. a chalcone and vitamin E, to produce a new class of pyranochalcones, represents a useful strategy for the preparation of new hybrids with an improved anti-inflammatory activity. To date, the only report dealing with the preparation of similar chalcone-vitamin E hybrids describes the applicability of a synthetic method, without evaluating their biological activity [9].
Recently, as part of a project dealing with the search for new biological activities in chalcone-vitamin E hybrids we observed a photoisomerization of the propenone moiety in the reaction products. Since spatial arrangement is of great importance in the interaction of drugs with biological targets, careful attention should be paid to changes in the torsion angles of the bonds in the propenone moiety of the chalcone core which can occur as a result of E-Z photoisomerization [10]. Furthermore, the many potential uses for chalcones in technology [11] and medicine [12], makes the possibility of phototransformation a major concern. Recent studies on the photoirradiation of chalcones and anthocyanins have contributed to the understanding of the network of chemical reactions that can occur in different reaction media [13-14], revealing that equilibrium in aqueous medium can lead to new skeletons depending on pH, light, and substitution patterns [13]. We wish to report herein on the conditions favoring the photoisomerization of the propenone moiety in chalcone-vitamin E hybrids as well as on the structure of a number of secondary products obtained under these conditions.

Fig.1. Structures of synthesized chalcone and retrochalcone-vitamin E hybrids: (E)-6’-O-tosyl-3,4,5-trimethoxy-δ-tocopherol-chalcone (1), (E)-3,4,5-trimethoxy-δ-tocopherol-chalcone (2), (E)-6’-O-tosyl-3,4,5-trimethoxy-δ-tocopherol-retrochalcone (3) and (E)-3,4,5-trimethoxy-δ-tocopherol-retrochalcone (4).
Experimental
NMR spectrometry
1H-NMR and 13C NMR spectra were recorded on a Bruker Avance or JEOL HY 400 MHz NMR spectrometers, using the specified deuterated solvents and their corresponding residual solvent signals as internal reference. For monitoring, spectra were recorded at reported irradiation times using 4 mg mL-1 solutions for each analog.
UV-visible spectroscopy
UV-visible spectra (200-800 nm) of 3 were recorded on an Agilent Cary 60 UV-Vis spectrophotometer using the specified solvent and spectra recorded at the specified irradiation times.
Synthetic procedures
( E)-6’-O-tosyl-3,4,5-trimethoxy-δ-tocopherol-chalcone (1) and (E)-6’-O-tosyl-3,4,5-trimethoxy-δ-tocopherol-retrochalcone (3)
In a 50 mL round bottom flask, 5-acetyl-6-O-tosyl-δ-tocopherol or 5-formyl-6-O-tosyl-δ-tocopherol (0.36 mmol) was dissolved with ethanol (10 mL). A solution of 3,4,5-trimethoxybenzaldehyde or 3’,4’,5’-trimethoxyacetophenone (0.37 mmol, 1 equiv.) in ethanol (5 mL), and lithium hydroxide (1.40 mmol, 3.9 equiv.) was added, and the reaction mixture was allowed to stir at room temperature (25 °C), until TLC showed the presence of the chalcone as the major product (cerium ammonium molybdate was used as stain). The reaction was quenched by adding 1M HCl (5 mL) until a color change from orange to yellow was observed; water was then added (10 mL) and the aqueous mixture was extracted three times with methylene chloride (15 mL). The combined organic fractions were dried over anhydrous sodium sulfate, filtered and the solvent removed under reduced pressure. The residue was purified by flash chromatography eluting with a mixture of n-hexane and ethyl acetate 9:1 v/v, to yield 1 or 3.
(E)-6’-O-tosyl-3,4,5-trimethoxy-δ-tocopherol-chalcone (1). (48 % yield) Pale yellow oil R f =0.34 (hexane-ethylacetate 8:2); 1H NMR (acetone-d 6 , 400 MHz) δ 7.66 (2H, d, J = 8.2 Hz), 7.32 (2H, d, J = 8.2 Hz), 7.12 (1H, d, J = 16.1 Hz), 7.01 (1H, s), 6.96 (2H, s), 6.81 (1H, d, J = 16.1 Hz), 3.88 (6H, s), 3.78 (3H, s), 2.57 (2H, dt, J = 7.8, 4.0 Hz), 2.30 (3H, s), 2.18 (3H, s), 1.84 - 1.68 (2H, m), 1.63 - 1.56 (2H, m), 1.55 - 1.49 (2H, m), 1.49 - 1.29 (10H, m), 1.28 (3H, s), 1.27 - 1.21 (2H, m), 1.19 - 1.06 (8H, m), 0.87 (6H, d, J = 6.7 Hz), 0.86 (3H, d, J = 6.7 Hz); 13C NMR (acetone-d 6 , 100 MHz) δ 193.7, 154.8 (2C), 151.5, 146.9, 146.6, 141.7, 139.3, 134.0, 132.4, 131.1, 130.9 (2C), 129.4, 129.2 (2C), 127.8, 122.8, 120.2, 107.1 (2C), 77.4, 60.8, 56.7 (2C), 40.7, 40.2, 38.3 (2C), 38.1, 38.1, 33.6, 33.5, 31.1, 28.8, 25.6, 25.2, 24.5, 23.1, 23.0, 21.7, 21.6, 21.1, 20.2, 20.2, 16.6.
(E)-6’-O-tosyl-3,4,5-trimethoxy-δ-tocopherol-retrochalcone (3). (50 % yield) Pale yellow oil R f =0.37 (hexane-ethylacetate 8:2); 1H NMR (CDCl3, 400 MHz) δ 7.63 (2H, d, J = 8.5 Hz), 7.58 (1H, d, J = 15.9 Hz), 7.48 (1H, d, J = 15.9 Hz), 7.33 (2H, s), 7.18 (2H, d, J = 8.5 Hz), 6.84 (1H, s), 3.97 (6H, s), 3.95 (3H, s), 2.74 (2H, t, J = 6.7 Hz), 2.37 (3H, s), 2.13 (3H, d, J = 0.7 Hz), 1.87 - 1.70 (2H, m), 1.54 - 1.48 (2H, m), 1.45 - 1.26 (9H, m), 1.25 (3H, s), 1.23 - 1.00 (10H, m), 0.86 (9H, d, J = 6.7 Hz), 0.84 (3H, d, J = 6.7 Hz); 13C NMR (CDCl3, 100 MHz) δ 189.0, 153.4 (2C), 151.0, 145.5, 142.6, 140.4, 136.6, 133.5, 133.1, 129.7 (2C), 129.4, 128.7 (2C), 127.7, 125.8, 122.8, 121.5, 106.3 (2C), 76.0, 61.1, 56.5 (2C), 40.0, 39.5, 37.6 (3C), 37.4, 32.9, 32.8, 30.8, 28.1, 24.9, 24.6, 24.0, 22.8, 22.7, 21.8, 21.2, 21.1, 19.9, 19.8, 16.5.
(E)-3,4,5-trimethoxy-δ-tocopherol-chalcone (2) and (E)-3,4,5-trimethoxy-δ-tocopherol-retrochalcone (4)
In a 50 mL round bottom flask, a suspension of 3,4,5-trimethoxy-6’-O-tosyl-δ-tocopherol-chalcone (1) or 3,4,5-trimethoxy-6-O-tosyl-δ-tocopherol-retrochalcone (3) (0.17 mmol) in MeOH (10 mL) was refluxed for 5 minutes until complete dissolution. A methanolic solution (5 mL) of NaOH 2M was added and reflux continued until complete consumption of the starting material was observed by TLC. The reaction was quenched with 1M HCl aqueous solution (15 mL) until a color change from red to yellow was observed; the resulting mixture was diluted with water (10 mL) and extracted three times with methylene chloride (20 mL). The combined organic fractions were dried over anhydrous sodium sulfate, filtered and after removing the solvent under reduced pressure, the crude reaction product was purified by flash chromatography, eluting with a mixture of n-hexane and ethyl acetate 9:1 v/v, to produce 2 or 4.
(E)-3,4,5-trimethoxy-δ-tocopherol-chalcone (2). (43 % yield) Orange oil R f =0.34 (hexane-ethylacetate 8:2); 1H NMR (acetone-d 6 , 400 MHz) δ 8.65 (1H, s), 7.39 (1H, d, J = 16.0 Hz), 7.11 (1H, d, J = 16.0 Hz), 7.03 (2H, s), 6.64 (1H, s), 3.88 (6H, s), 3.76 (3H, s), 2.70 (2H, t, J = 6.8 Hz), 2.14 (3H, s), 1.81 - 1.67 (2H, m), 1.63 - 1.56 (2H, m), 1.55 - 1.48 (2H, m), 1.47 - 1.28 (10H, m), 1.27 (3H, s), 1.20 - 1.03 (7H, m), 0.88 (3H, d, J = 6.7 Hz), 0.87 (6H, d, J = 6.7 Hz), 0.86 (3H, d, J = 6.7 Hz); 13C NMR (acetone-d 6 , 100 MHz) δ 196.9, 154.7 (2C), 149.7, 146.2, 144.7, 141.4, 131.5, 130.8, 128.8, 124.9, 119.8, 117.5, 106.9 (2C), 76.1, 60.7, 56.6 (2C), 40.6, 40.2, 38.3, 38.2, 38.1, 38.1, 33.6, 33.5, 31.9, 28.7, 25.6, 25.2, 24.5, 23.1, 23.0, 21.9, 21.7, 20.2, 20.1, 16.8.
(E)-3,4,5-trimethoxy-δ-tocopherol-retrochalcone (4). (55 % yield) Yellow gum R f =0.20 (hexane-ethylacetate 8:2); 1H NMR (CDCl3, 400 MHz) δ 7.96 (1H, d, J = 15.7 Hz), 7.79 (1H, d, J = 15.7 Hz), 7.29 (2H, d, J = 0.9 Hz), 6.57 (1H, s), 3.93 (9H, s), 2.85 (2H, t, J = 6.8 Hz), 2.16 (3H, s), 1.81 (2H, m), 1.53 - 1.47 (2H, m), 1.43 - 1.27 (9H, m), 1.25 (3H, s), 1.20 - 1.00 (10H, m), 0.86 (6H, d, J = 6.7 Hz), 0.85 (3H, d, J = 6.1 Hz), 0.83 (3H, d, J = 6.5 Hz); 13C NMR (CDCl3, 100 MHz) δ 190.7, 153.2 (2C), 149.1, 146.2, 142.4, 139.1, 134.0, 130.7, 125.3, 121.4, 118.1, 116.8, 106.3 (2C), 75.0, 61.1, 56.4 (2C), 39.8, 39.5, 37.6 (3C), 37.4, 32.9, 32.8, 31.3, 28.1, 24.9, 24.6, 23.8, 22.8, 22.7, 21.3, 21.1, 19.9, 19.8, 16.6.
Results and Discussion
During the synthesis of the chalcone and retrochalcone-vitamin E hybrids 1-4 (Fig. 1), the 1H-NMR spectrum of the purified protected E chalcone 1 showed, in addition to the vinylic proton signals at δ 6.81 (d, J = 16.1 Hz, β) and δ 7.12 (d, J = 16.1 Hz, β), the presence of a new set of signals at δ 6.28 (d, J = 12.8 Hz, β) and δ 6.84 (d, J = 12.8 Hz, β), which appeared over time. This suggested the possibility of an isomerization of the propenone moiety to produce the corresponding Z (s-cis) isomer 5. The fact that these changes were observed only when solutions of 1 in deuterated chloroform or acetone-d6 were exposed to sunlight (Fig. 2), and the absence of this new set of signals when similar samples were kept in the dark, confirmed that the changes were due to a photoisomerization process and ruled out the possibility of impurities present in the solvent being responsible for the changes.
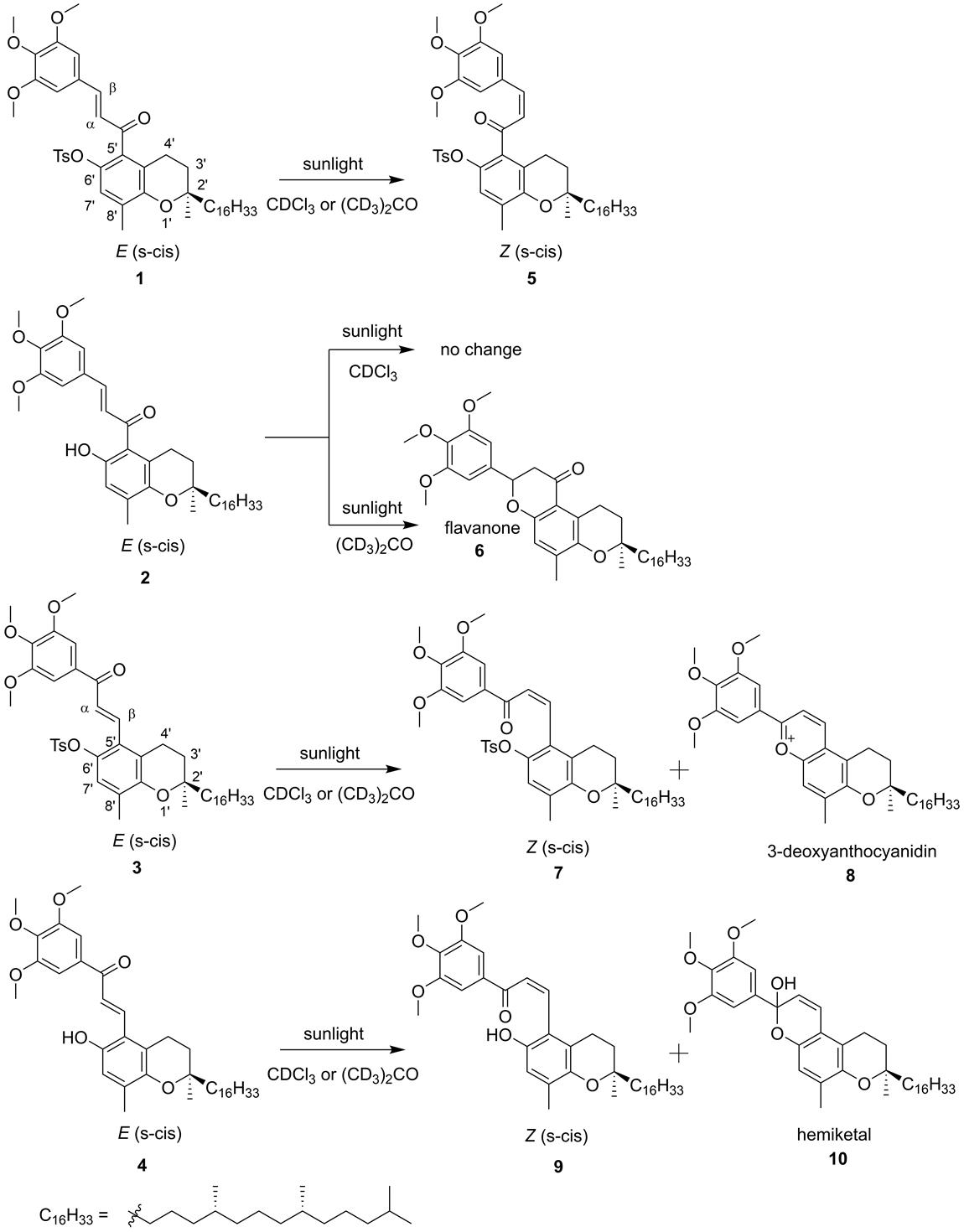
Fig. 2 Chalcone and retrochalcone-vitamin E hybrids 1-4 and their photoisomerization products. (Z)-6’-O-tosyl-3,4,5-trimethoxy-δ-tocopherol-chalcone (5), 3,4,5-trimethoxy-δ-tocopherol-flavanone (6), (Z)-6’-O-tosyl-3,4,5-trimethoxy-δ-tocopherol-retrochalcone (7), 3,4,5-trimethoxy-δ-tocopherol-3-deoxyanthocyanidin (8), (Z)-3,4,5-trimethoxy-δ-tocopherol-retrochalcone (9) and 3,4,5-trimethoxy-δ-tocopherol-3-deoxyanthocyanidin hemiketal (10).
Since photoisomerization of the propenone moiety in chalcones has been previously reported [10-12,13,15], the potential photoisomerization of the chalcone-vitamin E hybrids 1 and 2, together with that of the corresponding retrochalcone hybrids 3 and 4, was studied theoretically using computational chemistry methods. Energetic calculations on the change of the torsion angle of the carbon-carbon double bond in the propenone moiety showed that the two pairs of E chalcone/retrochalcone hybdrids follow a path that goes from the excited triplet state (T1), passing through a conical intersection to get to the singlet state (S0), to yield the corresponding Z isomer (Fig. 3). Since the calculated energy required to excite E chalcones 1-4 from the singlet to the triplet state is between 2.31 - 2.71 eV, and the fact that this amount of energy can be readily supplied by a photon of visible light [16], the photoisomerization of these four hybrids is expected to occur based on theoretical calculations.

Fig. 3 Theoretical energy diagrams of the photoisomerization of the two pairs of E chalcone/retrochalcone hybdrids. (A) tosyloxy-chalcone (1), (B) hydroxy-chalcone (2), (C) tosyloxy-retrochalcone (3), (D) hydroxy-retrochalcone (4). Singlet state ●; triplet state ▲; suggested path for E-Z isomerization ▬.
To demonstrate that this process was responsible for the observed changes, 1H-NMR data was obtained during a time-course study in which samples of each hybrid (1-4), dissolved in either deuterated chloroform or acetone-d6, were exposed to sunlight for different periods of time (Fig. 1S-8S). Samples of the protected chalcone 1 dissolved in deuterated chloroform or acetone-d 6 showed evidence of a light-induced E-Z isomerization by the presence of two AB spin systems corresponding to the α and β protons of the propenone moiety of each isomer (Fig. 4). The photoisomerization of 1 reached equilibrium after 5 min, showing a slightly higher proportion for Z isomer (5) formation in the sample prepared in acetone-d 6 (Fig. 9S). However, when a solution of the unprotected chalcone 2 in deuterated chloroform was exposed to sunlight, no photoisomerization appeared to take place as indicated by the 1H-NMR spectrum of the sample. Alternatively, sunlight exposure of an acetone-d 6 solution of 2 for one hour led to flavanone 6, identified by the absence of the signals for the vinylic protons in its 1H-NMR spectrum and the presence of the characteristic signals corresponding to H-2 (δ 5.39, dd, J= 13.4, 2.9 Hz) and H-3 (δ 3.06, dd, J= 16.4, 13.3 Hz; δ 2.73, dd, J= 16.4, 2.9 Hz) of the flavanone’s C-ring [Fig. 3S(e)].
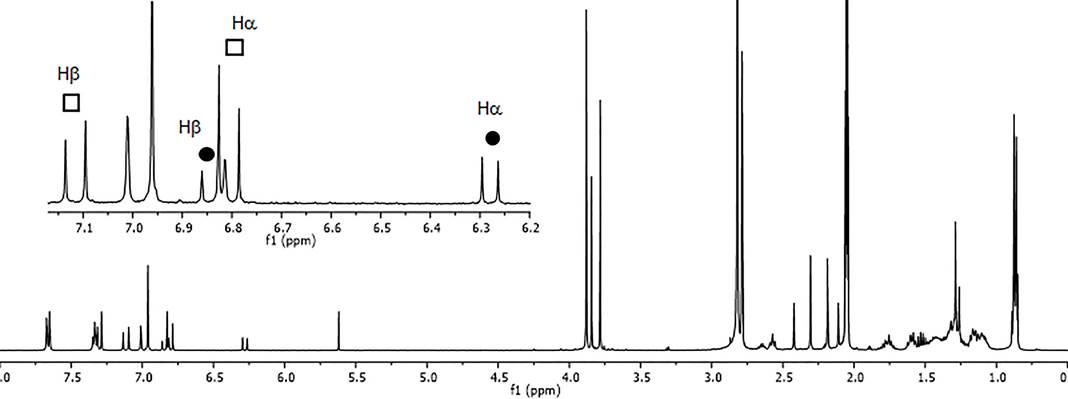
Fig. 4 1H-NMR spectrum (400 MHz, acetone-d 6 ) of 1 after sunlight irradiation (t = 20 min): E (s-trans) isomer , Z (s-cis) isomer .
Even though the photoreactivity of E-chalcones 1 and 2 coincides with that previously described for simpler chalcone analogs [17], the experimental results showed that this reaction does not take place in deuterated chloroform, and that the flavanone 6 is obtained in acetone-d 6 instead of the expected Z-chalcone. Similar cyclizations of simpler chalcone analogs of 2 leading to flavanone-type structures have been previously reported [17-18]; in these cases, formation of a six-membered tautomeric intermediate by means of an intramolecular hydrogen bond, between the carbonyl and the unprotected peri-hydroxyl group, reportedly favors the cyclization of the unprotected E-chalcone 2 rather than the expected Z-isomerization [17,19]. The inability of the E-chalcone 2 to form the flavanone 6 in deuterated chloroform under similar irradiation conditions, suggests that formation of the six-membered intermediate in this solvent is not favored.
Alternatively, sunlight exposure of both E-retrochalcone-hybrids 3 and 4 in deuterated chloroform and acetone-d 6 produced the expected photoisomerization products 7 and 9, respectively; additionally, cyclization products 3-deoxyanthocyanidin 8 and the hemiketal derivative 10 were also observed, with the latter identified on the basis of the characteristic H-3 and H-4 signals [20] at δ 5.86 (d, J= 9.8 Hz) and δ 6.78 (d, J= 9.8 Hz), respectively [Fig. 7S(d)]. The cyclization product 8 was identified on the basis of a new set of signals appearing at δ 9.29 (d, J = 9.1 Hz) and δ 9.66 (d, J = 9.2 Hz), which could be assigned to H-4 and H-3 of a flavylium system (Figures 6S and 10S), respectively [21]. In addition to 7 and 8, the 1H-NMR of the crude photoisomerization reaction product from 3 showed weak signals at δ 4.96 and δ 4.71, suggesting the formation of a dimeric derivative bearing a cyclobutane core [22]. Even though similar dimers have been previously isolated from chalcone-rich sources, the limited number of examples, and the low yields in which they are obtained, indicate that formation of these type of products is not favored [23-25].
The formation of 3-deoxyanthocyanidin (8) could be detected by the presence of a band at 500 nm, characteristic of flavylium-type skeletons [21,26], when using UV-vis spectroscopy to monitor the effect of sunlight irradiation on solutions of 3 in chloroform or acetone (Fig. 11S). These results, together with the development of a red pigmentation during sunlight irradiation of 3, confirmed the identification of 8. Although flavylium salts such as 8 are not expected to be stable in chloroform or acetone, its formation and stability could be explained on the basis of the amphiphilic properties of 8, since its positively charged “head” and its lipophilic “tail” could produce reverse micelle-type structures that encapsulate humidity in the medium (Fig. 12S) [27-28].
The presence of different photoisomerization products during the irradiation of 3 indicates that their formation depends on the solvent used, with faster maxima on the production of both the Z isomer 7 and the 3-deoxyanthocyanidin 8 obtained in deuterated chloroform (Fig. 5). The favored formation of 8 in chloroform can be explained by the heterogeneity of the chloroform-water mixture which stabilizes micellar structures. Alternatively, the more hydrophilic acetone favors solubility and dispersion of the 3-deoxyanthocyanidin 8, which in turn reduces aggregation and, consequently, stability [29-30].

Fig. 5 Formation of photoisomerization products during light irradiation of E-retrochalcone 3 in acetone-d 6 (A); and in deuterated chloroform (B): E-retrochalcone 3 ●, Z-retrochalcone 7 ▲ , 3-d eoxyanthocyanidin 8 ■ , cyclobutane core dimer ▬ .
Finally, unlike the unprotected E-chalcone 2, the unprotected E-retrochalcone 4 did produce the expected photoisomerization product 9, together with the hemiketal derivative 10, when exposed to sunlight in both deuterated chloroform and acetone-d 6 . In this case, the relative positions of the unprotected phenolic group and the conjugated carbonyl group in 4 prevented the cyclization to produce a flavanone-type product [26]. The formation of hemiketal 10 during the photoisomerization of 4, confirms that photoreactivity of chalcones can promote a complex equilibrium network to produce different derivatives [23,31].
It is interesting to mention that while formation of anthocyanins structurally similar to 8 has been reported to occur via an hemiketal intermediate during acid treatment of retrochalcones bearing an unprotected C-2 phenolic group [13], hemiketal 10 and 3-deoxyanthocyanidin 8 were not obtained as an intermediate and a product of the same reaction, but were obtained separately from the photoisomerization of the unprotected and protected E-retrochalcones 4 and 3, respectively. The fact that photoisomerization of the protected E-retrochalcone 3 produces 8, apparently without going through hemiketal intermediate 10, strongly suggests that the tosyloxy group in 3 plays a key role in the reaction, since tosyloxy groups are known to activate specific positions and to participate in different reactions. Even though examples of tosyloxy involvement in photochemical reactions are limited, the proposed photocyclization leading to the formation of 8 can only be explained through the participation of the tosyloxy group, as a leaving group during the final aromatization/oxidation step (Fig. 14S) [32-33].
Photo-induced changes in pharmaceuticals are a major concern during its development since any modification of the original structure and physico-chemical features can decrease, enhance or change a biological activity, and have a great impact in effectiveness and safety. However, the new chemical species obtained during the photoirradiation of chalcone-vitamin E hybrids 1-4 possess the potential to improve desirable properties such as the enhanced antiproliferative activity observed in chalcones isomerized from the E to the Z form [15], or the improved antiproliferative activity and cytotoxicity of chalcone dimers when compared to that of parent chalcones [25].
Conclusion
In summary, the results of this investigation confirm that chalcone-vitamin E hybrids are photosensitive pigments, and that their reactivity depends on both intrinsic and extrinsic factors such as substitution patterns and solvent of choice, respectively. Variations explored in solvents and substitution patterns, can influence the outcome of photo-induced processes, displaying formation of different chemical species. Even though chalcone transformation under light irradiation has been previously described, the results obtained in this investigation show that there exist new pathways in the photochemical reactivity network of chalcones to produce novel derivatives representing relevant scaffolds in the development of new pharmaceuticals.