1. Introduction
Iron is a transition metal ion and most minerals are found on the surface of the earth and the earth's crust. As an element in an organism's biological system, iron play important role in many vital reactions in metabolic activity stages (Gülle & Erbaş, 2017). In achieving its structural objectives, iron specifically binds to proteins and enzymes (Hua et al., 2016). Fe(III) is consumed as a micronutrient mineral and can interact with organic molecules. Deficiency or excess Fe(III) will not only cause dysfunction in several organs in humans including the liver, pancreas, and heart, but also cause hemochromatosis, diabetes, and anemia (Li et al., 2014). This is caused by the failure of hemoglobin, myoglobin, enzymes, and, the basic structure of many cofactors in cellular-level biochemical processes (Li et al., 2014; Li et al., 2016). Therefore, analytical tool for selective and sensitive Fe(III) control becomes very important (Yang et al., 2013).
The general techniques used in Fe(III) detection are Flame Atomic Absorption Spectrometry (FAAS) (Altunay et al., 2016), Capillary Electrophores (CE) (Panchenko et al., 2013) and Inductively Coupled Plasma-Atomic Emission Spectroscopy (ICP-AES) (Yang et al., 2015). Ion selective electrode devices (ISE) that are widely explored by analytical chemists induce the rapid growth of industry and technology worldwide because this technique represents a fast, accurate, and inexpensive method of analysis. The use of PVC membrane as an ISE sensor for the detection of metal as a sensor (Tang et al., 2018) to produces highly selective and sensitive metal sensors (Hassan et al., 2019; Joon et al., 2019). Therefore, intensive ionophore exploration is needed.
Humic compounds can bind to metal ions especially transition metals to form complexes (Hermanto, Ismillayli et al., 2022). This is due to the role of oxygen atoms contained in the functional groups of these compounds (Rigobello et al., 2017; Shah et al., 2018). The functional groups in HA are dominated by carboxylic groups (-COOH) (DiDonato & Hatcher, 2017; Gerke, 2018) and provide the greatest acidic properties compared to other functional groups. In this study, HA was modified to reduce the hydrophilic character of the compound to be applied as a membrane component but still retain its ability to bind metal. Introduce research on the design and synthesis of the PVC membrane as an ISE sensor based on EHA ionophore as an excellent sensory molecule for monitoring Fe(III) in the sample solution was conducted.
2. Materials and methods
2.1. Materials and instruments
The HA used for preparing an ionophore in the membrane sensor was pure HA from Merck (Germany). Pure ethanol (C2H5OH, 99%), chloroform (CHCl3, 99%), hydrochloride acid (HCl, 37%), sodium hydroxide (NaOH, 97%), and sulfuric acid (H2SO4, 99%) were received from Sigma-Aldrich. Polyvinyl chloride (PVC, Sigma) was used as the polymeric support, o-nitrophenyl octyl ether (o-NPOE, Sigma), dioctyl phthalate (DOP, Eastman™) were used as the plasticizers, sodium tetraphenylborate (Na-TPB, Sigma), and oleic acid (OA, Sigma) were use as the ion exchanger. All the components were dissolved in tetrahydrofuran (THF, Aldrich). The analyte solutions were prepared from a solid chemical compound (analytical grade from General Purpose Reagent) dissolved in deionized water.
Electrochemical protocols were performed with a pH and ion meter (TOA Electronic Ltd model IM-20E) with Ag-AgCl comparative electrodes (TOA No. HS-305DS) and modified electrodes for membrane electrodes. Stirrer magnets and heaters (IKA Combimag-RET motor) were used to stir the solution. A Spectrophotometer FTIR-Prestige 21 Shimadzu was employed for all FTIR analyses.
2.2. Synthesis EHA
Esterification of the carboxylate group on pure HA (Merck) produces an esterification derivative of HA (EHA) with a methyl ester from the carboxyl group (Andjelkovic et al., 2006). Among the many methods of esterification described, perhaps one of the simplest and the most efficient methods is a condensation of a carboxylic acid with an alcohol, generally uses sulfuric acid or halide acid which is recognized as an esterifying agent because of complete conversion results (Gunawardena et al., 2017).
EHA was prepared by mixing HA solution and ethanol (99% and used without further purification), as described by Riggs (2017). The solution was made from 5 mL of deionized water and 0.05 mL of NH4OH 14 M was added (approx. pH 10). The HA solution was prepared by dispersing 100 mg HA into the solution, by stirring at 400 rpm (1 h) using a magnetic stirrer. This dispersion was added 50 mL of ethanol with stirring at 400 rpm for 2 h, then placed into a 250 mL single-neck round bottom flask and heated to reflux with a condenser for 1 h. The esterifying agent, H2SO4 (66%) (approx. 0.5 mL) was added to acidify the dispersion to induce Fischer esterification. The dispersion was continued by reflux and mixed for a period of 6 h. The results obtained were filtered and extracted into chloroform-water (1:1), and then chloroform was evaporated at room temperature.
2.3. Electrode preparation
The synthesized EHA has good solubility in PVC membrane (THF) solvents. Ionophore as molecular recognition was immobilized into the supporting matrix (Hermanto, Kuswandi et al., 2019; Hermanto, Mudasir et al., 2019; 2020). EHA as an ionophore could be used well in PVC membranes because of its lipophilicity. PVC membrane was prepared according to general procedures. Materials needed for membrane construction are PVC powder, EHA, plasticizer (o-NPOE and DOP), and anion lipophilic (Sodium-TPB and oleic acid) mixed with a total weight of 100 mg and dissolved in 1 mL of dry THF, according to the composition in Table 1. Mixture the resulting product was transferred to a petri dish (i.d 2 cm) and the solvent is slowly evaporated until an oily concentrated mixture can be obtained. The Pyrex tube (3-5 mm above it) is dipped in a mixture of oily membranes for about 5 sec so that a transparent membrane with a thickness of about 0.3 mm will form. After removing the tube from this mixture, the tube was kept at room temperature for about 24 hours to vaporize THF. The membrane was cut according to the size of the electrode body and attached to the end of the electrode body and filled with an internal filling solution (1.0×10-3 M of Mn+ solution as electrolyte solution). Finally, the electrode was conditioned by immersing it in 1.0×10-2 M of Mn+ solution for 24 hours.
2.4. EMF Measurement
Working electrodes used PVC membrane and wire of Ag-AgCl while internal reference electrode (comparative electrode) utilized Ag-AgCl. Both electrodes were used in the measurement of the electromotive force (emf) (Hermanto, Mudasir, et al., 2022). The emf was measured by membrane electrode with the following cell assembly: Ag-AgCl | internal solution, 1.0 × 10−3 M Mn+ solution| PVC membrane | test solution | Ag-AgCl, KCl (saturated). The brand TOA No. HS-305DS ion meter model IM-20E (TOA Electronic Ltd.) was used for potential measurement.
3. Results and Discussion
Esterification reaction on HA is focused on the mechanism of the reaction between the carboxyl group (-COOH) on HA with alcohol (ethanol) catalyzed acid, without looking at the R group (side chain) of the compound. Then, EHA was formed under the optimum reaction conditions (Figure 1). The synthesized EHA was identified through FTIR analysis and is based on the absorption of certain wavenumbers by the functional groups in the structure of the compound.
It is shown below in Figure 2, the comparison of the FTIR spectra for the EHA samples and pure HA for comparison. The peak of O-H stretching at about 3425.58 cm-1 decreases in intensity, indicating -OH in HA was reduced and -OH derived from carboxylic acid (-COOH) turns into esters (-OR), furthermore the residual is O-H stretching by phenol. The peak for sp2 and sp3 (C-H) stretching at 2924.09; 2854.65 cm-1 for pure HA and EHA increases in intensity indicating -CH in the EHA formed.
The carbonyl peak (C=O stretching) at 1705.07 cm-1 for pure HA shifted to 1720.50 cm-1 for EHA samples (Andjelkovic et al., 2006), and carboxylic acids/esters become more pronounced from HA to EHA (Riggs, 2017). The peak C=C aromatic stretching at 1627.92 cm-1 for pure HA also increases in wavenumber to 1612.49 cm-1, this indicates the presence of conjugated esters (EHA). The peak C-H aliphatic bending at about 1458.18 cm-1 indicates EHA formed. The peak for -COO- at 1381.03 cm-1 (for HA) shifted to 1373.32 cm-1 (for EHA) and the peak for C-O stretching at 1165.00 cm-1 (for HA) also shifted to 1257.59 cm-1, due to loss of OH bending showed that EHA was formed. The peak of -O-C-C stretching at 1033.85 cm-1 indicates that EHA formed from primary alcohol (ethanol). The FTIR spectra of modified pure HA (EHA) confirmed that esterification of the carboxyl groups occurred, due to the differences in the IR absorption before and after esterifying modification.
EHA has excellent solubility in organic solvents (membrane solvents such as THF) and homogeneously mixes with other membrane constituent components, becoming an excellent ionophore in PVC membranes for ISE sensors. In the making process of sensor membranes, an ionophore (EHA) was trapped inside a semipermeable sensor membrane. EHA, as a receptor that selectively interacts with metal ions, can provide sensor responses in the form of changes in physical signals (emf).
According to the Lewis acid-base theory, compounds containing O and N atoms (such as EHA) are donor atoms (Lewis bases), having functions as ionophores on the membrane ISE sensor. The active site in the EHA is the -OH group of phenolic and the -COOR (ester) group is a hard Lewis base and tends to form strong bonds with hard Lewis acids based on HSAB theory or the Pearson principle. In this case, EHA can selectively interact with Fe(III). The complex of Fe(III) (as a guest) with EHA (as a host or ligand) based on their interaction can be assumed as a host-guest model where Fe(III) as a ball is trapped in a cavity-like structure of the EHA that has a cyclic or open chain. The cavity side of the ligand contains polar groups of electronegative atoms such as oxygen and the phenolate-OH group of EHA used to interact with Fe(III).
Characteristic in determining the performance of the ISE sensor is the potentiometric
coefficient, log
Table 2 Selectivity coefficients of the various interfering ions on separate solution method Ag-AgCl | 0.1 M Fe(NO3)3 | PVC membrane | test solution | Ag - AgCl | KCl (saturated).
Metal Ion | log |
|||
---|---|---|---|---|
Membrane 1 | Membrane 2 | Membrane 3 | Membrane 4 | |
Fe(III) | 0.00 | 0.00 | 0.00 | 0.00 |
K(I) | -3.90 | -3.41 | -3.66 | -2.48 |
Na(I) | -4.20 | -3.29 | -3.86 | -2.17 |
Li(I) | -4.14 | -3.07 | -2.71 | -2.22 |
Ag(I) | -3.64 | -2.96 | -2.62 | -2.46 |
Ca(II) | -1.62 | -1.37 | -3.16 | -2.58 |
Pb(II) | -0.98 | -2.24 | -2.67 | -2.47 |
Cu(II) | -1.65 | -1.40 | -2.60 | -2.21 |
Ni(II) | -0.89 | -0.94 | -2.87 | -2.20 |
Zn(II) | -1.82 | -2.15 | -4.70 | -2.43 |
Al(III) | -0.43 | -0.16 | -1.32 | -1.15 |
La(III) | -0.86 | -2.36 | -0.72 | -0.48 |
Cr(III) | -0.39 | -0.83 | -0.51 | -0.35 |
The effect of the plasticizer on the characteristics of the membrane was studied by varying the composition of the membrane, namely the amount of plasticizer with a fixed amount of PVC and ionophores. Based on Table 2, the selectivity coefficient values for membranes 2 and 4 are higher than membranes 1 and 3, meaning that membranes with DOP plasticizer are more selective than membranes with o-NPOE. The level of basicity of DOP is greater than o-NPOE so that the selectivity of the DOP membrane to the cationic analyte increases. In previous work (Mihali & Vaum, 2014), it was found that a high-basicity plasticizer could significantly increase the ISE selectivity. Anion lipophilic also influences ISE selectivity. It was found that oleic acid was better at increasing the ISE selectivity than Na-TPB.
Optimization of pH on the sensor membrane is performed to determine the effect of pH (efficient pH conditions) on the emf measured. The optimum pH of the membrane sensor for the selective electrode ion is pH 3, as shown in Figure 3. At pH <3 Fe (III) protonation occurs (forming hydronium ions) and at base pH, Fe (III) forms iron hydroxide which is easily precipitated so that the sensor response is low.

Figure 3 The pH dependence of the membrane ISE sensor was evaluated over a pH range of 2.5-8.0 in a 1.0×10−3 M Fe(III) solution.
Sensor response time is the efficient time to produce high emf and washing time is the time to reach zero emf. The results of the measurement of response time and washing time on the sensor membrane can be seen in Figure 4, this shows the optimum response time is ± 50 s and the washing time is ± 110 s. The response time is influenced by several factors including membrane resistance, the kinetics of ion transfer reactions, and the process of diffusion in the membrane. It is also influenced by the concentration of the analyte, the concentration of the ionizer, and the speed of stirring the solution.
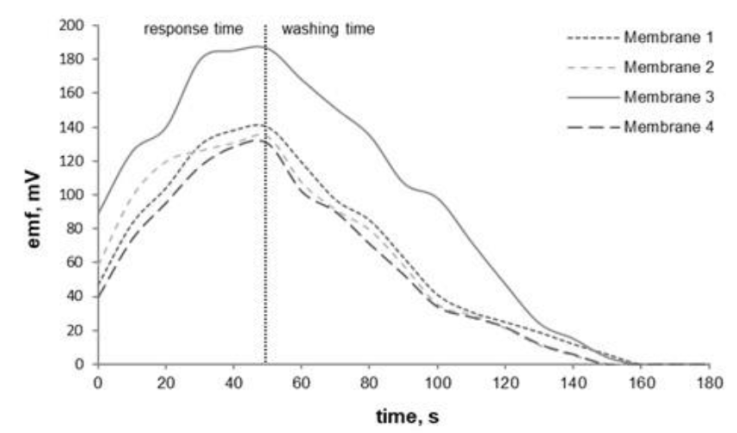
Figure 4 Response time and washing time of the membrane ISE sensor were tested over a time range of 0.0- 180 s in a 1.0×10−3 M Fe(III) solution at pH 3.
Table 3 shows that the Fe (III) ISE sensor calibration curve for each membrane has a dynamic range at a concentration of 10-7-10-1 M for Fe (III) and a detection limit of 10-7 M. The Nernst factor is one of the determinants of ISE sensor performance, this shows the level of electrode sensitivity to the main ion (i) is determined from the calibration curve slope between the emf versus the logarithm of the main ion concentration. Table 3 shows the sensor response slope for membranes 1, 2, 3, and 4 respectively 20.21±0.95; 19.85±0.69; 19.96±0.75 and 19.35±0.68 mV per decade, this didn’t differ significantly from the Nernst factor for trivalent ions theoretically, which is ± 19.72 mV per decade. The use of different plasticizers affected the sensitivity of the ISE sensor as indicated by the Nernst factor value. Membranes 1 and 3 using the o-NPOE plasticizer have higher Nernst factor values than membranes 2 and 4 using the DOP plasticizer. The greater polarizability of o-NPOE may be the cause of the superior sensitivity of membranes using o-NPOE plasticizers. Meanwhile, the effect of anionic lipophilic on membrane sensitivity was shown by the superiority of Na-TPB against oleic acid. This is in line with the previous work by Paul et al. (2018). Table 3 lists and compares the analytical characteristics of the ISE sensor with those of the best Fe (III) ISE sensor reported in the literature (Badakhshan et al., 2019; Ozer & Isildak, 2018, 2019; Zamani et al., 2009). The data in Table 3 clearly shows that the performance of this sensor is superior to that reported by other researchers.
Table 3 Comparison of analytical characteristics of the different Fe(III) ISE sensor.
ISE Sensor | Ionophore | dynamic range (M) | Nernst factor (mV/decade) | limit of detection (M) |
---|---|---|---|---|
Membrane 1 | EHA | 1.0x10-7 to 1.0x10-1 | 20.21±0.95 | 1.0x10-7 |
Membrane 2 | EHA | 1.0x10-7 to 1.0x10-1 | 19.85±0.69 | 1.0x10-7 |
Membrane 3 | EHA | 1.0x10-7 to 1.0x10-1 | 19.96±0.75 | 1.0x10-7 |
Membrane 4 | EHA | 1.0x10-7 to 1.0x10-1 | 19.35±0.68 | 1.0x10-7 |
Zamani et al. (2009) | 4,4’DBTS | 1.0×10−6 to 1.0×10−2 | 19.4±0.5 | 3.6×10−7 |
Ozer & Isildak (2018) | morin-Fe2+ | 1.0×10−6 to 1.0×10−1 | 56.14±0.22 | 4.5×10-7 |
Ozer & Isildak (2019) | Fe(II)phthalocyanine | 1.0×10-6 to 1.0×10-1 | 26.04±0.95 | 1.8±0.5×10-7 |
Badakhshan et al. (2019) | benzo‑18‑ crown‑6 | 1.0×10−6 to 1.0×10−1 | 19.51±0.10 | 8.0×10-7 |
Sensor lifetime is determined by calibration curves every week and the sensor stability is obtained in 5 weeks, as shown in Figure 5. In week 6 there is a deviation from the initial Nernst factor (slope calibration curve) so the optimum sensor is used for 5 weeks. The lifetime of PVC membrane electrodes was generally limited this is due to the slow elution of membrane components (ionophore and plasticizers) into the sample solution. Overall the four membranes have a similar lifetime with membrane 3 being slightly superior to the other three membranes. Lifetime sensors depend on the mechanical properties of membranes such as flexibility, resistance to organic compounds, oxidizing agents, the acidity of solutions, and the degree of solubility in the water phase. The addition of a plasticizer increases membrane flexibility by increasing free volume between polymer chains which allows chain segments to move or rotate more freely as well as the ionophore so that its active site can interact with the analyte. The increase in free volume also facilitates the penetration of the analyte to react with ionophores (Gurtova et al., 2013). The long alkyl chain of the plasticizer increases membrane lipophilicity so that it can suppress ionophore leaching from the membrane (Zahran et al., 2014). This also synergizes with the use of lipophilic anions which have a longer alkyl group, hence membrane 3 with o-NPOE plasticizer and oleic acid as lipophilic anion has the lowest lifetime reduction in the sixth week compared to the other three membranes. Sensor lifetime is determined by calibration curves every week and the sensor stability is obtained in 5 weeks, as shown in Figure 5. In week 6 there is a deviation from the initial Nernst factor (slope calibration curve) so the optimum sensor is used for 5 weeks. The lifetime of PVC membrane electrodes was generally limited this is due to the slow elution of membrane components (ionophore and plasticizers) into the sample solution. Overall the four membranes have a similar lifetime with membrane 3 being slightly superior to the other three membranes. Lifetime sensors depend on the mechanical properties of membranes such as flexibility, resistance to organic compounds, oxidizing agents, the acidity of solutions, and the degree of solubility in the water phase. The addition of a plasticizer increases membrane flexibility by increasing free volume between polymer chains which allows chain segments to move or rotate more freely as well as the ionophore so that its active site can interact with the analyte. The increase in free volume also facilitates the penetration of the analyte to react with ionophores (Gurtova et al., 2013). The long alkyl chain of the plasticizer increases membrane lipophilicity so that it can suppress ionophore leaching from the membrane (Zahran et al., 2014). This also synergizes with the use of lipophilic anions which have a longer alkyl group, hence membrane 3 with o-NPOE plasticizer and oleic acid as lipophilic anion has the lowest lifetime reduction in the sixth week compared to the other three membranes.
To evaluate the capability of the proposed membrane sensor is applied in the analysis of Fe(III) detection of water samples such as tap water and bottled drinking water. The 10 mL sample solution was added to 5 mL H2O2 1N solution and 5 mL HNO3 1N solution to oxidize Fe(II) to Fe(III), and then the solution was diluted with buffer pH solution 3 (range of effective sensor pH) in 100 mL flask measuring. The concentration of Fe(III) in tap water and bottled drinking water samples was determined directly by using a calibration method, the results of these determinations can be seen in Table 4. The results of the study show that the sensor membrane developed has a very good performance for the determination of Fe(III) in the aqueous solution. It could be seen in Table 4 that there is a match of results that did not differ significantly between measurements with the sensor membrane and the reference method using atomic absorption spectrometry (AAS).
4. Conclusions
EHA can be synthesized through the esterification reaction of carboxylic groups from HA and ethanol with sulfuric acid as a catalyst. EHA as an ionophore can be homogeneous on the lipophilic membrane ISE sensor, and the sensor was selective to Fe(III) at its optimum conditions, such as pH 3. The character of selective electrodes Fe(III) based on PVC membrane with EHA as ionophore has a response time of ± 50 seconds, a dynamic range measurement of 10-7-10-1 M, a Nernst factor or a sensitivity of ± 19.72 mV per decade, a detection limit of 1.10-7 M, sensor membrane lifetime for 5 weeks.