1. Introduction
The Ground Penetrating Radar (GPR) is a non-damaging geophysical method that is used in several disciplines for investigating sub-surfaces. By using electromagnetic waves, GPR systems detect changes in the properties of the penetrated surfaces and so concealed objects can be detected. The antenna is considered as an important hardware component in the GPR systems since it generates the necessary electromagnetic waves. Hence, the penetration and the detection capabilities of the GPR systems are decided principally by the performances of the antenna and by the radiated electromagnetic waves (Chaabane & Guerroui, 2021; Grinč, 2015; Guerroui et al., 2021; Hendevari et al., 2018; Raza et al., 2019). The circularly polarized (CP) feature is needed for the GPR antennas since the performances of the GPR systems depend mainly on the reflected waves containing the indications (Takach et al., 2016). In this context, several GPR antennas have been reported in recent literature such as those proposed in (Chaabane & Babouri, 2019; Elsheakh & Abdallah, 2019; Joula et al., 2018; Kundu et al., 2018; Kundu, 2018; Liu et al., 2019; Li et al., 2016; Mohanna et al., 2018; Raza et al., 2020) but only few have CP characteristic like those presented in (Elsaid et al., 2019; Liu et al., 2015; Raychaudhuri et al., 2016, Stillman et al., 2016). Unfortunately, the CP GPR antennas available in the literature have complex structures and/or have enormous voluminous size. Thus, there is a strong need to design easy-to-fabricate and inexpensive GPR antennas that have a high competence of the penetration and of the investigation.
In this study, a compact CP UWB antenna is proposed for GPR applications. The designed antenna is formed by a question mark-shaped radiating patch and a truncated ground plane. The proposed antenna was simulated and optimized by employing the software CST Microwave StudioTM (Computer Simulation Technology, 2016). A prototype for the antenna is fabricated and measured by using R&S®ZNB Vector Network Analyzer. Encouraging results were achieved within the interesting bandwidth which indicate the aptitude of the antenna to work as a GPR antenna.
2. Antenna design and results
Both the front view and the back view of the proposed CP UWB antenna are schematically depicted in the Fig. 1. The antenna design development steps are given in the Fig. 2. The antenna is built on a 1.5-mm-thick FR-4 epoxy with an area of 0.7λ0×0.793λ0 at 7 GHz and a relative permittivity of 4.4. The designed antenna is constructed by a question mark-shaped radiating patch and a truncated ground plane. The goal of the chosen shapes for the radiating patch and for the ground plane is mainly to more enlarge the IBW. Other advantages for the chosen shapes are that the weight of the antenna and the conduction losses can be reduced. The optimized dimensions for the designed antenna are as follows: LS=30 mm, Lg=8 mm, LP=19.5 mm, L1=1.92 mm, L2=5.38 mm, L3=1.58 mm, L4=6 mm, L5=5.2 mm, L6=3.85 mm, L7=3.5 mm, L8=3.85 mm, L9=6.25 mm, L10=1.35 mm, L11=12.4 mm, WS=34 mm, WP=18.6 mm, W1=3.26 mm, W2=1.6 mm, W3=2.3 mm, W4=2.79 mm, W5=6 mm, W6=5.2 mm, W7=3.5 mm, W8=5.8 mm, W9=1.6 mm, W10=2.6 mm, W11=9.8 mm.
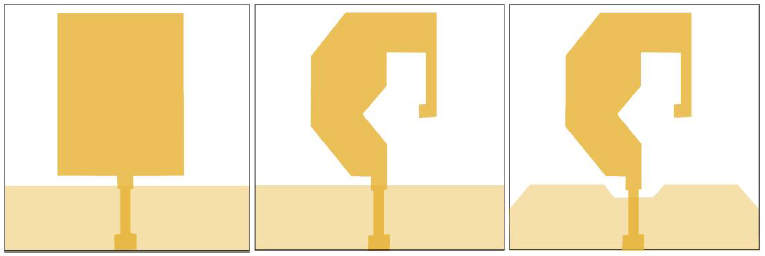
Figure 2 Antenna structure evolution during the design steps, (a) Antenna A, (b) Antenna B, (c) Antenna C.
Fig. 3 shows that the IBW and the impedance matching of the designed antenna are highly improved by modifying the shape of the radiator from the rectangular shape to a question mark shape and by cutting the metal from the truncated ground plane; approximately an enhancement of around 81.51% for the IBW is achieved. Fig. 4 indicates that the CP characteristic is introduced by the use of a question mark-shaped patch; an axial ratio bandwidth (ARBW) of about 73.7% is achieved. This ARBW is further enhanced by eliminating metal from the truncated ground plane; the attained ARBW is approximately around 76.81%.
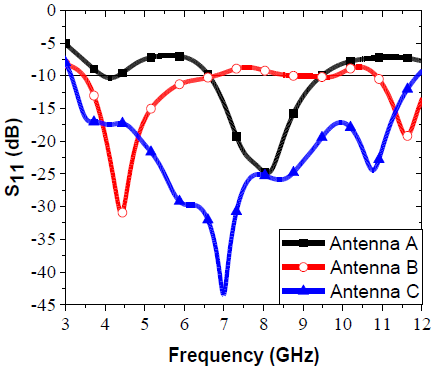
Figure 3 Reflection coefficient comparison of the designed antenna with those of the initial antennas.
Fig. 5 shows that the designed antenna has a good input impedance characteristic within the operating bandwidth which confirms the well adaptation of the antenna. The real part of the input impedance is nearly varying around 50 ohms value while the imaginary part is nearly around zero value. To understand the generation of the circularly polarized radiations, the current distributions on the surface of the radiating patch and on the surface of the truncated ground plane at the frequency 7 GHz for different phases (0°, 90°, 180°, 270°) are depicted in Fig. 6. The principle direction of the current is along the +X-direction for the phase 0° and it is in the opposite direction (-X-direction) for the phase 180°. Besides, the principle direction of the current is along the -Y -direction for the phase 90° and it is in the opposite direction (+Y-direction) for the phase 270°. Thereby, as the phase augments in time, the main current surface in the azimuth plane of the antenna rotates in clockwise trend which shows a left-handed circularly polarized in the direction of propagation.
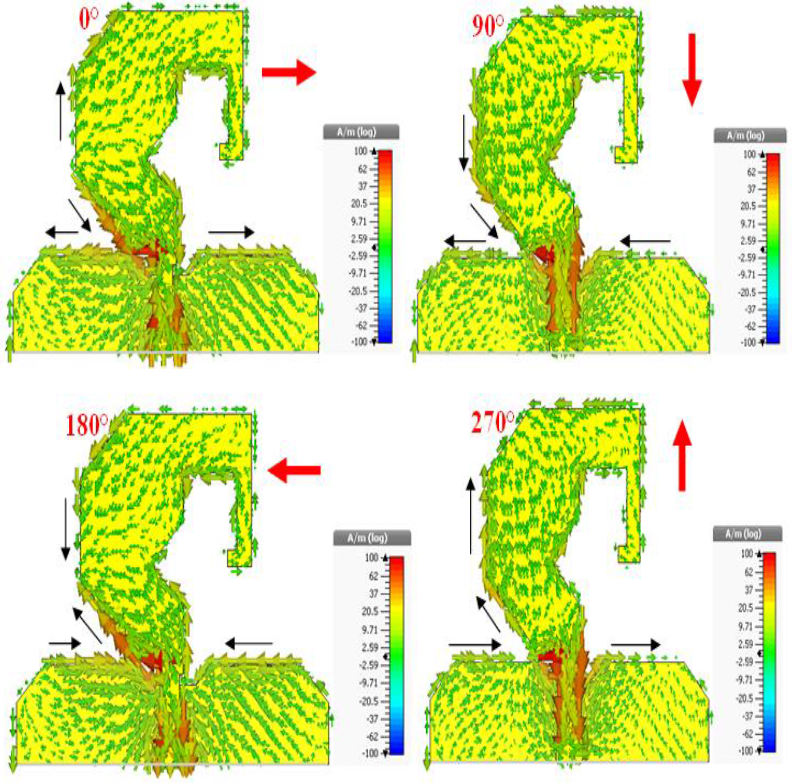
Figure 6 Current distribution on the surface of the antenna at the frequency 7 GHz for different phases.
A fabricated antenna based on the optimized parameters was realized by using a LPKF S103 laser printer. A photo of the fabricated prototype is presented in Fig. 7. The working frequency band of the fabricated prototype was measured by utilizing R&S®ZNB Vector Network Analyzer. It is observed that the experimental result is in a good agreement with the simulated one and the UWB feature is proved. Fig. 8 indicates that the fabricated antenna works in a wide bandwidth ranging nearly from 3.47 GHz to 12.11GHz (110.91%) with a feeble peak of about 2.5 at 4.51GHz which may be attributed to the intolerance of the connector and/or of the soldering thus to the losses in the port of the connection.
The simulated right-handed circularly polarized (RHCP) and left-handed circularly polarized (LHCP) far-field radiation patterns of the antenna in xz- and yz-planes at 5 GHz, 7 GHz, and 9 GHz are depicted and compared in Fig. 9 and 10, respectively. The minor inclinations of the radiation patterns are due to asymmetric geometry of the designed antenna. It is confirmed that the designed antenna radiates LHCP in the direction of the propagation of the antenna (+z-direction) and RHCP in the reverse direction (−z-direction); the opposite circular polarization is due to the type of this antenna that has bidirectional radiation patterns.
The simulated antenna gain and antenna radiation efficiency are presented in Fig. 11. It shows that the antenna exhibits reasonable values of the gain ranging almost between 3.17 dBi and 6 dBi within the operating bandwidth; the achieved values are better than the ones obtained in (Hosain et al., 2020). Moreover, high radiation efficiency values are achieved which are almost over than 80% within the working bandwidth; these values are better than the ones presented in (Saeidi et al., 2019). A comparison between the proposed antenna and some recently published CP antennas is presented in Table 1. It indicates that the characteristics of the proposed structure are better or comparable to those of some previous works
Table 1 A comparative analysis between the proposed antenna and some recently published CP antennas.
Antennas | Substrate | Sizes (mm3) | ARBW | IBW |
---|---|---|---|---|
Pan et al. 2018 | FR4 | 80×80×1.6 | 0.91 GHz - 0.97 GHz (6.4%) | 0.894 GHz - 1.03 GHz (14.1%) |
Verma et al. 2020 | FR4 | 60×60×13.45 | 3.34 GHz - 4.12 GHz (20.91%) | 3.14 GHz - 4.35 GHz (32.31%) |
Cheng et al. 2017 | Rogers 4003 | 70×75×0.8 | 2.1 GHz - 2.7 GHz (25%) | 2.2 GHz - 2.8 GHz (24%) |
Zheng et al. 2018 | Arlon AD350A | 38.8×38.8×3.5 | 5.05 GHz - 6.15 GHz (19.64%) | 4.55 GHz - 6.95 GHz (41.74%) |
Ding et al. 2016 | FR4 | 55×50×1 | 2.05 GHz - 3.95 GHz (63.33%) | 1.48 GHz - 4.24 GHz (96.50%) |
Zahran et al. 2019 | Ultralam 3850 | 50×42×0.1 | 7.9 GHz - 9.9 GHz (22.47%) | 2.6 GHz - 10 GHz (117.46%) |
Chen et al. 2019 | FR4 | 50×50×0.8 | 3.1 GHz - 7 GHz (77.23%) | 2.15 GHz - 6.97 GHz (105.70%) |
Zhang et al. 2018 | FR4 | 58.8×58.5×1.2 | 3 GHz - 6 GHz (66.67%) | 2.9 GHz - 8 GHz (93.5%) |
This work | FR4 | 30×34×1.5 | 4.13 GHz - 9.28 GHz (76.81%) | 3.47 GHz - 12.11 GHz (110.91%) |
3. Conclusions
A compact printed CP UWB antenna with question mark-shaped patch has been proposed for GPR applications. The working bandwidth has been enlarged by eliminating the metal form the truncated ground plane and by using a question mark-shaped radiating patch. The simulated results show that the designed antenna operates between 3.14 GHz and 11.88 GHz. The CP performance for the antenna has been proved showing that it has a 3-dB ARBW ranging from 4.13 GHz to 9.28 GHz (76.81%). Experimental results have revealed that the proposed antenna with the dimensions of 0.7λ0×0.793λ0×0.035λ0 at 7 GHz has an impedance bandwidth extending from 3.47 GHz to 12.11 GHz (110.91%). Hence, these satisfactory results support the candidature of the proposed antenna for GPR applications.
Conflict of interest
The authors have no conflict of interest to declare.
Financing
The authors received no specific funding for this work.