1. Introduction
Pollution from petroleum hydrocarbon products generates a great environmental concern (Mishra et al., 2020; Ramadass et al., 2018). Crude oil and its derivatives pollute the environment mainly during storage and transportation. Events such as oil spills are particularly dangerous because the pollutant fills the pores of the soil, changing its parameters, and can also reach and contaminate groundwater (Mena et al., 2016).
The sludge generated during the washing of vehicles, as well as those that are generated in processes of tanking and oil change in service stations and fuel sales; they can be loaded with hydrocarbons, so it is necessary for these discharges to be pretreated from a grease trap. Under the flotation principle, fats and oils are retained by gravitational separation generating retention of solids that may be contaminated with hydrocarbons (Ministerio de Ambiente, 2007). The bad management of these residues can generate great environmental impacts, being able to remain in some ecosystems for more than six years due to their low rate of degradation, drastically affecting environmental matrices such as soil, water and air, including human health (da Silva & Maranho, 2019; Ramadass et al., 2018; Varjani et al., 2017; Varjani & Upasani, 2017).
Bio-piles, also known as bio-cells or compost piles, are used to reduce total petroleum hydrocarbon (TPH) concentrations through the use of biodegradation. This technique can reduce the presence of TPH by stimulating aerobic microbial activity, through aeration, additions of nutrients, minerals, and moisture (EPA, 2004). The techniques of bioaugmentation (application of nutrients) and biostimulation (application of microorganisms) are the most common techniques used for the remediation of environments contaminated with petroleum derivatives. These techniques allow modifying the nutrients soluble in water, leaching quickly from the sediment (Bai et al., 2020; Li & Yu, 2015; Yun et al., 2017), Nevertheless, its effectiveness depends on different factors such as: microbial population density (colony-forming units - CFU), soil pH, moisture content, soil temperature, nutrient concentration, soil texture, TPH volatility, chemical structure, concentration and toxicity (EPA, 2004).
It has been reported that biostimulation and bioaugmentation can generate 60% and 34% degradation of TPHs, while other studies indicate between 36% and 75% removal efficiency (Adams et al., 2020). The predominant bacteria associated with the bioaugmentation process belong to the Achromobacter, Acinetobacter, Aeromonas, Alcaligenes, Arthrobacter, Pseudomonas, Nocardia, and Bacillus genus (Varjani, 2017). Studies carried out on soils contaminated with petroleum lubricating oils (ALP) showed a significant elimination of the presence of TPH, achieving a 95% reduction in the pollutant load, using slurry and natural surfactant materials to accelerate the microbial remediation (Rodríguez Eugenio, Natalia; McLaughlin & Pennock, 2019). All biological degradation techniques have different degrees of efficiency; however, they present a better cost-benefit ratio, when compared to thermal, physical or chemical technologies (Couto et al., 2010; Gkorezis et al., 2016).
2. Experimental
21 kg samples were collected from the sludge produced in three of the main automotive service stations in the city of Tunja-Boyacá, Colombia. The collection process was carried out according to the NTC 3656 standard. The samples were mixed, homogenized under the ASTM C702 standard to later obtain samples of 4.5 kg. The samples were deposited in high-density polyethylene containers capable of being hermetically sealed.
The material was treated under two bioremediation methods for 12 weeks in samples of 4.5 kg each. In the biostimulation technique (M1), where 50 ml of soil bioremediatory composed of Pseudomona-type- microorganisms were added and supplied by CorpoBoyacá. For the bioaugmentation technique (M2), 50 ml of bioremediator produced from the humus of Californian red worms were added. This bioremediator was produced at the laboratory level, from worm humus and molasses, being this purely organic.
The humus and isolated microorganisms (Pseudomonas, among others - patent pending), were deposited in a bioreactor to which pasteurized molasses and treated water were added to promote their proliferation and growth in the medium. As a result of the composting process, leachates loaded with organic nutrients and pathogens were obtained, for which a recirculation and mixing had to be carried out until these were no longer produced. With the use of certain enzymes (patent pending) the humus was converted from a solid state to a liquid state and then be negatively charged with the help of an electronic source.
After the process, this humus in liquid phase was used directly in the soil contaminated with hydrocarbons to generate a state of biostimulation of the microorganisms present. Finally, the processes were monitored from a control sample (Mc). All the soil samples were periodically mixed three times a week to provide atmospheric oxygen, improve the porosity of the medium, and redistribute the pollutant load, avoiding possible biological contamination due to the transfer of microorganisms between the containers used. In general, the moisture of the soil samples was maintained at a percentage equivalent to 90% of the field capacity (18%).
In the experimentation phase, three replicas were made for each of the techniques (biostimulation and bioaugmentation), having as measurement parameters: pH, moisture (ASTM D-2216), temperature, performing two weekly measurements. The total hydrocarbon content of petroleum (TPH), according to ASTM D-5520 and extraction of material with n-hexane (HEM), according to EPA 9071B, were carried out at 0, 6, and 12 weeks of the experience. Measurements were made by the gravimetric method, using a Soxhlet extractor.
3. Results and discussion
The sludge samples produced in different automotive service stations in the city of Tunja-Boyacá, Colombia, were treated under two bioremediation methods, analyzing their effects during the 12-weeks period. The parameters taken into account for this study were pH, moisture, temperature, and TPH. The initial conditions of the samples are presented in Table 1.
Table 1 Initial conditions of homogenized sample.
Parameter | Unit | Value |
---|---|---|
pH | --- | 7.98 |
Moisture | % | 30.64 |
Temperature | °C | 17.5 |
Total hydrocarbons (wet) | mg·kg-1 | 20411,8 |
Total hydrocarbons (dried) | mg·kg-1 | 29430,4 |
The results of the M1 and M2 techniques were averaged to establish a trend and improve the graphic representation of each of the variables analyzed in this research. Figure 1(a) shows the temperature behavior during the 12 weeks of study. As can be seen, all the samples presented a similar operating temperature range, which was between 14°C and 17.5°C. The ambient temperature range varied from 12°C to 18°C (weather station - UPTC).
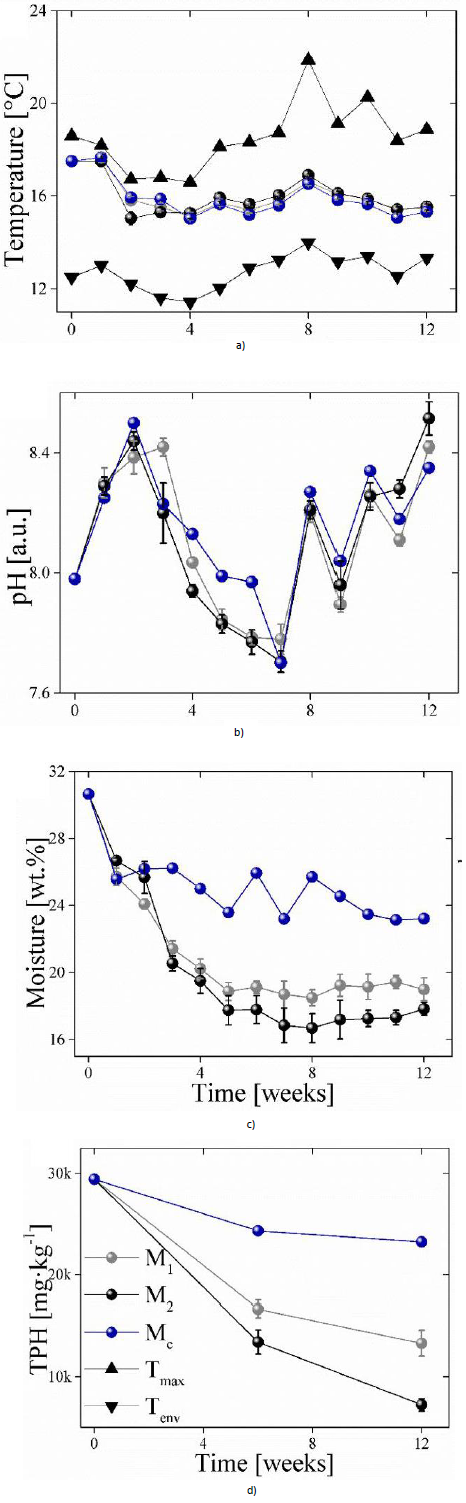
Figure 1 Behavior of the samples during the study in relation to: a) temperature, b) pH, c) moisture, and d) TPH.
The fluctuations in the temperature of the soil samples coincide with the temperatures measured in the environment, showing the same behavior during the study period. As it is a wet sample, the heat loss is greater when this is compared with a dry sample. Regardless of the variation in ambient temperature, the soil samples maintained an almost constant average temperature, standing in the range of 15.5°C. This situation would be associated with the transformation of HPT, a product of microbiological activity. To be effective, bioremediation processes require an operating range between 18°C and 30°C. Nevertheless, some other authors observed degradation processes in broader ranges, which can range from 5°C to 35°C. (Toro & Quirama, 2012).
Figure 1(b) shows the moisture behavior of the M1, M2, and Mc techniques. As mentioned above, moisture was controlled in all samples from fifth week, when a moisture percentage close to the field capacity value was presented. All samples started with a moisture percentage of 30.64% at the start of this study, which gradually decreased to 18% and 17% for M1 and M2 respectively in the same week. From this point on, the moisture was controlled with the addition of water. The sample Mc presented the minimum variation in moisture, establishing an average value of 25% throughout the study. Comparing the moisture values, it was possible to establish that the reduction is more significant in the M2 technique, which could be associated with a higher rate of microbiological activity compared to the M1 technique. The control of soil moisture is important since it favors the solubility of nutrients, improving their availability for microorganisms. Nevertheless, excess moisture can generate saturation of the medium, limiting the entry of oxygen into the soil pores (EPA, 2004).
Figure 1(c) shows the behavior of pH during the bioremediation process. All the soil samples presented an initial pH close to 8.0, being the upper limit in which it is considered that the metabolic rate of the microorganisms will not be affected (6.0 - 8.0) (EPA, 2004); However, it was not decided to alkalize the soil samples since the degradation of the hydrocarbons can generate weak organic acids (H2CO3), which can end up controlling the pH. The ranges of pH values are similar to those presented by Mora et al. 2020, varying from 7.7 to 8.5; however, these values can affect microbial populations, influencing the availability of carbon and energy sources. In addition, pH can also affect the solubilization and absorption of contaminants and ions present in the sample (da Silva & Maranho, 2019; Ubani et al., 2013).
The hydrocarbon determination was carried out in week 0, 6 and 12, with the Soxhlet extraction method and gravimetric determination. Figure 1(d) shows the reduction of TPH for the samples treated by the M1, M2 and Mc techniques during the study period. All soil samples had an initial concentration of 29430.44 mg·kg-1 of TPH at the beginning of the study.
In Figure 2 the TPH reduction percentages are presented for the samples treated by each of the techniques used in this study. In the sixth week, a reduction of 34.6 and 42.5% was observed for the M1 technique and 46.2% and 53.7% for the M2 technique in wet and dry samples respectively. In the twelfth week, a reduction of 46.2% and 54.9% was observed for the M1 technique and 70.9% and 75.4% for the M2 technique in wet and dry samples.
The dry Mc, presented a removal percentage of 17.3% and 21.0% at 6 and 12 weeks, showing microbiological activity due to the reduction of TPH, although losses of hydrocarbons due to volatilization processes, product of mixing, are not ruled out-ground. From the trends it can be established that to achieve a removal greater than 99% of PHT, 21 weeks are required for the M1 technique and only 15 weeks for the M2 technique; Therefore, it is established that bioaugmentation is more effective in terms of treatment time. The effectiveness of this technique presents very favorable values to those found in the literature, with lower initial PHT concentrations and treatment periods longer than those worked in this study (Crisafi et al., 2016; Nova, 2018; Ñustes, 2012).
n general, the removal percentages are much higher during the first 6 weeks of treatment; if compared with those obtained at the end of the study. This kinetic behavior, known as Hockey Stick, is caused by the decrease in nutrients and unfavorable physicochemical conditions such as temperature, pH; among others (Jasmine & Mukherji, 2019; Ñustes, 2012; Ramadass et al., 2018; Speight & El-Gendy, 2018; Varjani et al., 2020). In both M1 and M2 techniques, a growth of plant material was observed, while the control sample did not present any physical change in addition to the loss of moisture.
These results, obtained under real conditions of experimentation, generate a great incentive in the application of this bioremediation product for the treatment of sludge contaminated with hydrocarbons in the region, presenting great results and ease of acquisition. Thus, future research is expected focused on analyzing the effects on the variation of the composition of this material.
4. Conclusions
The bioremediation process was carried out on three samples of sludge contaminated with TPH produced in different automotive service stations in the city of Tunja-Boyacá, Colombia, using biostimulation and bioaugmentation techniques for 12 weeks.
It is observed that the implemented bioaugmentation method (patent-pending) generates a better response in the biodegradation process of soils contaminated with TPH. This technique managed to generate up to a 75.4% reduction in TPH content, while the M1 system achieved a 54.9% reduction in TPH in the same period of time.
The controlled conditions at the laboratory level did not allow the internal temperature of the soil samples to increase in a more significant way, affecting in a certain way the degradation rate of the TPHs, as well as the metabolic activity of the microorganisms. It is presumed that the results could improve in warmer climates, reducing treatment times.
The use of this product as a bioremediation agent can be implemented in the treatment of this type of oily sludge in the region, since it has presented satisfactory results in real conditions on a laboratory scale. Future research is expected to analyze the effect of temperature variation, as well as the composition of this material within the bioremediation processes of sludge contaminated with petroleum hydrocarbons.