Introduction
In nature, three different anhydrous crystalline polymorphs of calcium carbonate (CaCO3) exist (calcite, aragonite, and vaterite), two well-defined hydrous crystalline polymorphs (calcium carbonate monohydrate and calcium carbonate hexahydrate), and one amorphous form (ACC) (Meldrum and Colfen, 2008). The biological process by which CaCO3 polymorphs are synthesized is called biomineralization (Kocot et al., 2016; Song et al., 2019). The shell of mollusks is the most studied CaCO3 biomineral, because is the most abundant biomineral in nature and it is relatively easy to obtain (Demichelis et al., 2018). The shell of bivalves is mainly composed of CaCO3 (95 - 99 %) and an organic matrix containing acidic proteins, ꞵ-chitin, glycoproteins among other molecules (1 - 5 %) that function as a scaffold for mineral nucleation and plate formation (Wolf et al., 2013).
The periostracum, the outer layer of the shell, is the first defense of the organism against external agents, it is not calcified and it is made of organic compounds (Kocot et al., 2016). After the periostracum, the prismatic layer is composed mainly of calcite, the most stable and the second most abundant CaCO3 polymorph in mollusks; it crystallizes in a rhombohedral system making it a resistant and tough material to penetrate by forming prismatic structures (Bahn et al., 2017). Beneath prismatic layer is the mother of pearl (nacre) layer, which is formed by aragonite. This polymorph has an orthorhombic form and it is less stable than calcite in isolated conditions, however, in the shell becomes a tougher, stiffer, and stronger material by assembling aragonite plates into structures that resemble a “brick-wall” (Evans, 2019). Besides calcite and aragonite, mollusks are capable to manipulate vaterite, the most unstable CaCO3 polymorph present in the shell when their shell suffers a deformation (Wilt, 2005).
Vesicles of the mantle in mollusks act as ion storage sites of vaterite or amorphous calcium carbonate (ACC) (Addadi et al., 2006). ACC is the hydrated form of CaCO3 with a poorly order that contains magnesium (Addadi et al., 2003), and acts a transient precursor of more stable CaCO3 polymorphs (Politi et al., 2008). There are two models that try to explain CaCO3 nucleation. The first model, initiates with prenucleation, in which the ionic solution creates metastable clusters to begin the nucleation phase were single ions attach to the cluster and finally, depending on thermodynamic factors, calcite, vaterite, aragonite or ACC will be formed in the postnucleation phase. In second model, the prenucleation phase is pH-dependent to form a stable cluster, where the nucleation phase will begin depending on cluster concentration, its aggregation, or ion attachment. During the postnucleation phase, ACC is formed, and then calcite, aragonite, and vaterite are crystallized (Demichelis et al., 2018).
Shell growth in mollusk is mediated by the shell matrix proteins (SMPs) from the mollusk shells. SMPs are synthesized in the mantle cells and released to the extrapallial fluid (EPF), between the mantle and the inner face of the shell (Wilt, 2005). The EPF contains a variety of ions which interact with SMPs (Wilt, 2005). SMPs are classified as soluble and insoluble proteins according to their solubility after the decalcification procedure used to extract them from the shell. Soluble proteins are rich in acidic hydrophilic residues, they are found between the crystals forming part of the scaffolding where CaCO3 deposits (Levi-Kalisman et al., 2001), while insoluble proteins are found inside the crystals, they have a high proportion of aliphatic amino acids forming short repetitive domains that have been associated with nucleation by promoting the interaction with acidic polyanionic soluble proteins (Wolf et al., 2013; Du et al., 2018).
Crystal polymorph growth in the mollusk shell is modulated by SMPs protein concentration and by the presence of cofactors. Low protein concentration (1 µg/mL) of some SMPs (e.g. PfN44), have been related to calcite growth with few aragonite crystals formation, but the increase in protein concentrations (2.5 µg/mL) have shown a total inhibition of aragonite deposition (Pan et al., 2014). However, other SMPs such as Pif97 and pearlin showed inhibition of calcite crystal growth and ACC stabilization in vitro in increasing amounts of proteins (Montagnani et al., 2011; Bahn et al., 2015). This significant discrepancy between proteins could be related to their function in shell formation, either as a modulator of calcite or aragonite, or both. Besides protein concentration, Mg2+ ions have been reported to modulate aragonite growth (Pan et al., 2014; Ma and Feng, 2015). Low magnesium content induces calcite growth while high magnesium content induces the formation of aragonite (Raz et al., 2000). In Hyriopsis cumingii, the water-soluble matrix of aragonite pearls formed little needle-like crystals, accompanied by irregular Mg-calcite structures when low magnesium is present (10 mM). However, in the presence of high magnesium ions (40 mM), quasi-spherical aragonite aggregates appear from needle-like crystals, coexisting with a few Mg-calcites (Ma and Feng, 2015).
Other proteins, such as SPARC (secreted protein acidic and rich in cysteine), from P. fucata, participate in nacre formation by stabilizing vaterite to inhibit calcite, as well as by forming aragonite in presence of Mg2+ or other proteins (Xie et al., 2016). Nevertheless, there are also proteins able to produce aragonite crystals in presence of magnesium or calcium (40 mM) as cofactors, such as the glycoprotein Ps19 from Pteria sterna (Arroyo-Loranca et al., 2020). Even when this information suggests that SMPs can to modulate crystal polymorphs by protein and ion concentrations, further research is needed to support this statement since the crystallization assay previously reported in several SMPs are not always comparable due to the different preparations used.
To understand the biomineralization processes is necessary to known the mechanism used by SMPs to control crystal deposition, either by protein and/or ion concentration. Therefore, this research aimed to understand the role of Ps19, a novel SMPs, with no homology to previously described proteins, on crystal deposition, by evaluating the effect of protein concentration (0.2 - 1.2 μg·μL-1) and cofactor concentration (40/100 mM of MgCl2, CaCl2, 1:1 MgCl2:CaCl2 and MgSO4) on crystal polymorph deposition in vitro. The results indicate Ps19 is an effective promoter of aragonite and calcite in the presence of MgCl2 and CaCl2, respectively. Also, this positive modulation is dependent on protein and ion concentration. Thus, this suggests that Ps19 may be involved in the prismatic and nacre layer of Pteria sterna.
Material and methods
Ps19 extraction from P. sterna shell
Perlas del Cortez S. de R.L. MI., granted three adult organisms from Bahia de La Paz, B.C.S. Oysters were transported to the Centro de Investigaciones Biológicas del Noroeste S.C. facilities where shells were pulverized, and 20 g of the powder were decalcified with cold acetic acid (10 % v/v) at 4 °C and constant stirring according to Montagnani et al. (2011); later, the solution was centrifuged to obtain the Acetic Soluble Matrix (ASM) and the Acetic Insoluble Matrix (AIM) as described by Arroyo-Loranca et al. (2020).
SDS-PAGE
The AIM, and later the Ps19 protein were loaded into a sodium dodecyl sulphate polyacrylamide gel electrophoresis (SDS-PAGE) according to Laemmli (1970), the samples were treated as previously described by Arroyo-Loranca et al. (2020). A quantity of 60.3 μg of AIM and 15.1 μg of P. sterna shell Ps19 per gel were loaded. An SDS-PAGE molecular weight marker was used for molecular weight comparison (Broad range, Bio-Rad 161-0317). Electrophoresis was conducted at 90-V at room temperature, using a Bio-Rad electrophoresis unit (Protean II). After electrophoresis, the gel was stained with Coomassie Brilliant Blue R250 (CBB) for 2 h, washed out, and analyzed using a Chemi Doc XRS (Bio-Rad).
Protein quantification by densitometry
Ps19 quantification was performed by pixel densitometry in a 16 % SDS-PAGE gel stained with CBB. A protein standard curve was constructed with ovalbumin protein (0.25 - 8.0 μg·μL-1) as described by Arroyo-Loranca et al. (2020). A Chemi Doc XRS (Bio-Rad, California, USA) was used to analyze the image of the gel to obtain the linear equation (1) and calculate the quantity of the proteins present in the samples.
The Ps19 protein was purified from the AIM of P. sterna shell by four preparative polyacrylamide gel electrophoresis according to the Mini-Prep Cell manual (Bio-Rad, 491 Prep Cell) according to Arroyo-Loranca et al. (2020). A total of 100 fractions of 200 μL each were collected, selected fractions were loaded into an SDS-PAGE polyacrylamide gel (16 %) to identify the fractions containing the Ps19 protein. Then, fractions containing Ps19 were pooled, concentrated, and cleaned from the electrophoresis buffer by centrifugation with an Amicon Ultra-4 filter (EMD Millipore) as described by Arroyo-Loranca et al. (2020). The purified protein (380 μL) was stored at -20 °C.
Ps19 identification
The purified Ps19 was analyzed to verify the properties of the previously characterized protein by Arroyo-Loranca et al. (2020). The molecular weight of Ps19 was calculated through its Rf, the calcium-binding capability was corroborated by the Stains-All stain (Green et al., 1973) after electrophoresis separation as previously described. A total of 15.1 μg of Ps19 in an SDS-PAGE 16 % polyacrylamide gel was used.
Calcium carbonate crystallization in vitro
Calcium carbonate (CaCO3) crystallization in the presence of Ps19 was evaluated by the incubation of four saturated solutions and three differente protein concentrations that shown in Table 1. Each combination (10 μL of Ps19 and 50 μL of saturated solution) was placed over a glass coverslip inside a Petri dish with absorbent paper at the bottom to prevent condensation. The Petri dishes were sealed with parafilm, placed into a container, and incubated for 30 days at 4 °C. Controls and solutions 1 to 4 (50 μL) were mixed with sterile distilled water (10 μL) instead of Ps19 and the protein by itself (10 μL) was mixed with 50 μL of sterile distilled water. The morphology of the crystals was analyzed by Scanning Electron Microscopy (SEM) at the Electronic Microscopy Laboratory at Centro de Investigaciones Biológicas del Noroeste (CIBNOR), México. Every experiment and analysis were performed by triplicate.
Tabla 1: Condiciones para la cristalización de CaCO3in vitro en presencia de Ps19.
Solution | Salt | Cofactor | Molarity of the cofactor (Mm) | pH | Ps19 (μg·μL-1) | Expected CaCO3 polymorph | Reference |
1 | 40 mM CaCl2, 100 mM NaHCO3 | MgCl2 | 40 or 100 | 8.2 | 0.2 | Aragonite | (Weiss et al., 2000) |
0.7 | |||||||
1.2 | |||||||
2 | 100 mM Na2CO3 | CaCl2 | 40 or 100 | 8.2 | 0.2 | Calcite | (Declet et al., 2016) |
0.7 | |||||||
1.2 | |||||||
3 | 40 mM CaCl2, 100 mM Na2CO3 | MgSO4 | 40 or 100 | 8.2 | 0.2 | Aragonite | (Nielsen et al., 2016) |
0.7 | |||||||
1.2 | |||||||
4 | 100 mM NaHCO3 | CaCl2 and MgCl2 | 40 or 100 | 8.2 | 0.2 | Calcite/ aragonite | (Loste et al., 2003) |
0.7 | |||||||
1.2 |
Raman spectroscopy
Raman spectra were identified by using an InVia micro-Raman spectrometer (Renishaw, Gloucestershire, UK) with an excitation line of 532 nm provided by a YAG laser of 100 mW and a spot size of 2 μm x 2 μm. For measurements, the slits were set at 200 μm and a 100× objective was used. The crystals were scanned by triplicate for 90 seconds from 100 to 1900 cm−1 for the specific identification.
Results
Ps19 isolation and quantification
The acid-soluble (ASM) and acid-insoluble (AIM) matrixes were obtained by extraction with cold acetic acid. The AIM was separated by denaturalizing electrophoresis and visualized by CBB stain (Figure 1A). The Ps19 protein was identified by determining its molecular weight (~19 kDa) through its relative mobility along with the gel.
Figure 1 Acetic insoluble matrix (AIM) from the shell of Pteria sterna. (A) AIM in a SDS-PAGE 16 % polyacrylamide gel stained with CBB. MWS: molecular weight standard (Bio-Rad 1610317), AIM: acetic-acid insoluble matrix proteins, the arrow indicates the Ps19 protein. (B) Isolated Ps19 shell protein from P. sterna and Ca2+ binding capability (Stain all). Ova: ovalbumin (negative control).
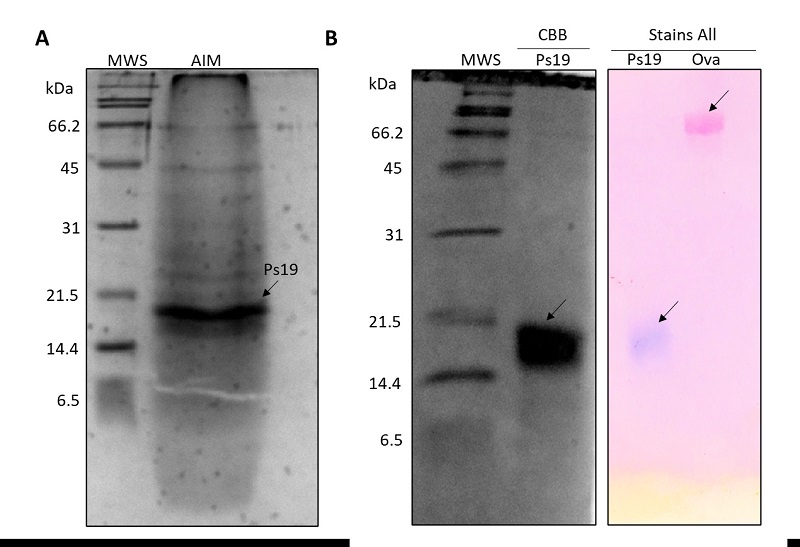
Figura 1: Matriz acética insoluble (AIM) de la concha de Pteria sterna. (A) AIM en un gel de poliacrilamida SDS-PAGE al 16 % teñido con CBB. MWS: estándar de peso molecular (Bio-Rad 1610317), AIM: proteínas de matriz insolubles en ácido acético, la flecha indica la proteína Ps19. (B) Proteína de la concha Ps19 de P. sterna y capacidad de unión a calcio (tinción Stain all). Ova: Ovoalbúmina (control negativo).
The Ps19 protein was purified by preparative electrophoresis (Figure 1B). From the four electrophoreses, a total of 576 μg of Ps19 were purified from 3.4 mg of unpurified protein present in the AIM extracted from the pulverized shell, having a protein (Ps19) with a 17 % yield and 94 % purity (Table 2).
The amount of purified Ps19 was determined by pixel densitometry (Figure 1B). The CBB and Stains-All stains corroborated that the isolated protein was Ps19, which had the expected molecular weight (19 kDa) and presented Ca2+ binding capability (Figure 1B) as described by Arroyo-Loranca et al. (2020).
CaCO3 crystallization in vitro
The modulation of CaCO3 crystal polymorph formation by Ps19 was evaluated by SEM. The Ps19 protein was able to produce calcite crystals when MgCl2 was used as a cofactor at 40 and 100 mM (Figure 2). At 40 mM MgCl2, Ps19 crystallized calcite crystal plates in their typical geometric shape with smooth sides (Figure 2 F-H), which were comparable in size than those observed in the controls (Figure 2E, 3I-K). At 100 mM MgCl2 (Figure 2), only hexagonal vaterite was formed, plates were more structured, and stacked forming platelet interlocks (white arrow, Figure 2B). Increase of Ps19 concentration displayed crystal inhibition at 40 mM when protein was set at 1.2 µg·μL-1, however, this behavior was not observed at 100 mM MgCl2, and CaCO3 crystals presented diamond shape instead of hexagonal, as seen at 0.2 and 1.2 µg·µL-1 Ps19 concentration.
The addition of Ps19 to the solution containing 40 and 100 mM CaCl2 did not generate crystals of calcite or aragonite (Figure 3). At 40 mM rod-like crystals were observed (Figure 3F, G), while high CaCl2 concentration displayed scarce rod-like crystals (Figure 3B) and isolated polycrystalline deposits generating halos or inhibition zones (Figure 3C). The increase of Ps19 concentration at 40 and 100 mM did not produce any significant pattern on crystal deposition.
Figure 2 SEM micrographs of calcium carbonate crystals growth in vitro in the presence of 40 mM CaCl2, Mg2+ ion (40 and 100 mM), 100 mM NaHCO3 and Ps19 at increasing concentrations (µg·µL-1). First panel from left to right (A, E) Negative controls without Ps19; 100 mM Mg2+ ion in presence of (B) 0.2 µg·µL-1, (C) 0.7 µg·µL-1, (D) 1.2 µg·µL-1 Ps19; 40 mM Mg2+ ion in presence of (B) 0.2 µg·µL-1, (C) 0.7 µg·µL-1, (D) 1.2 µg·µL-1 Ps19; (I-K) protein alone without salt. Scale bars are 500 µm. Hexagonal structures are shown in white lines, and arrow indicates platelet interlocks. Each picture is representative of three independent crystallization assays.
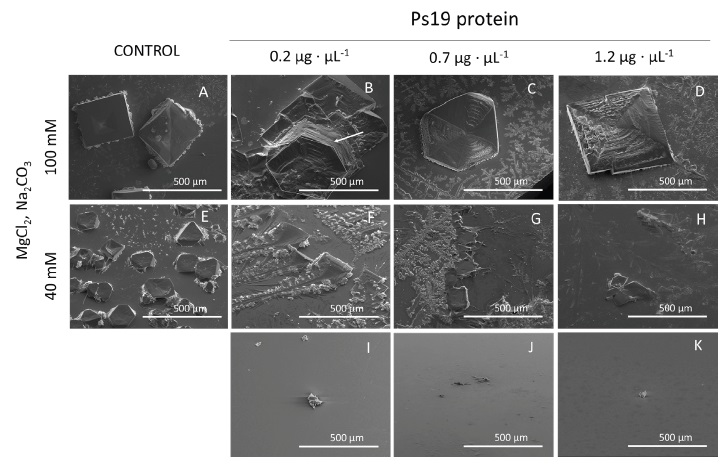
Figura 2: Micrografías SEM del crecimiento de cristales de carbonato de calcio in vitro en presencia de CaCl2 40 mM, iones Mg2+ (40 y 100 mM), NaHCO3 100 mM y Ps19 a concentraciones crecientes (µg·µL-1). Primer panel de izquierda a derecha (A, E) Controles negativos sin Ps19; ion Mg2+ 100 mM en presencia de (B) 0,2 µg·µL-1, (C) 0,7 µg·µL-1, (D) 1,2 µg·µL-1 Ps19; ion Mg2+ 40 mM en presencia de (B) 0,2 µg·µL-1, (C) 0,7 µg·µL-1, (D) 1,2 µg·µL-1 Ps19; (I-K) proteína sola sin sal. Las barras de escala son de 500 µm. Las estructuras hexagonales se muestran en líneas blancas y la flecha indica interbloqueos de plaquetas. Cada imagen es representativa de tres ensayos de cristalización independientes.
Figure 3 SEM micrographs of calcium carbonate crystals growth in vitro in the presence of Ca2+ ion (40 and 100 mM), 100 mM Na2CO3 and Ps19 at increasing concentrations (µg·µL-1). First panel from left to right (A, E) Negative controls without Ps19; 100 mM Ca2+ ion in presence of Ps19 protein (B) 0.2 µg·µL-1, (C) 0.7 µg·µL-1, (D) 1.2 µg·µL-1 Ps19; 40 mM Ca2+ ion in presence of (B) 0.2 µg·µL-1, (C) 0.7 µg·µL-1, (D) 1.2 µg·µL-1 Ps19; (I-K) protein alone without salt. Scale bars are 500 µm. Each picture is representative of three independent crystallization assays.

Figura 3: Micrografías SEM del crecimiento in vitro de cristales de carbonato de calcio en presencia de ion Ca2+ (40 y 100 mM), Na2CO3 100 mM y Ps19 a concentraciones crecientes (µg·µL-1). Primer panel de izquierda a derecha (A, E) Controles negativos sin Ps19; ion Ca2+ 100 mM en presencia de (B) 0,2 µg·µL-1, (C) 0,7 µg·µL-1, (D) 1,2 µg·µL-1 Ps19; ion Ca2+ 40 mM en presencia de (B) 0,2 µg·µL-1, (C) 0,7 µg·µL-1, (D) 1,2 µg·µL-1 Ps19; (I-K) proteína sola sin sal. Las barras de escala son de 500 µm. Cada imagen es representativa de tres ensayos de cristalización independientes.
Saturated solution containing MgSO4 as source of Mg2+ ion resulted in CaCO3 precipitation as ACC (Figure 4). At 40 mM MgSO4, ACC polymorph was predominant in the solution, however small aragonite structures were visible at 0.2 and 0.7 µg·µL-1 Ps19 protein (Figure 4F-H). But, at 100 mM MgSO4 no rhombohedral or even facetted crystals at all were spotted, instead agglomerations of spheres were observed at all Ps19 concentrations tested (Figure 4B-D).
Figure 4 SEM micrographs of calcium carbonate crystals growth in vitro in the presence of 40 mM CaCl2, Mg2+ ion (40 and 100 mM) as MgSO4, 100 mM Na2CO3 and Ps19 at increasing concentrations (µg·µL-1). First panel from left to right (A, E) Negative controls without Ps19; 100 mM Mg2+ ion in presence of (B) 0.2 µg·µL-1, (C) 0.7 µg·µL-1, (D) 1.2 µg·µL-1 Ps19; 40 mM Mg2+ ion in presence of (B) 0.2 µg·µL-1, (C) 0.7 µg·µL-1, (D) 1.2 µg·µL-1 Ps19; (I-K) protein alone without salt. Scale bars are 500 µm, inlets are 50 µm size. Each picture is representative of three independent crystallization assays.

Figura 4: Micrografías SEM del crecimiento de cristales de carbonato de calcio in vitro en presencia de CaCl2 40 mM, iones Mg2+ (40 y 100 mM) como MgSO4, Na2CO3 100 mM y Ps19 a concentraciones crecientes (µg·µL-1). Primer panel de izquierda a derecha (A, E) Controles negativos sin Ps19; ion Mg2+ 100 mM en presencia de (B) 0.2 µg·µL-1, (C) 0.7 µg·µL-1, (D) 1.2 µg·µL-1 Ps19; ion Mg2+ 40 mM en presencia de (B) 0.2 µg·µL-1, (C) 0.7 µg·µL-1, (D) 1.2 µg·µL-1 Ps19; (I-K) proteína sola sin sal. Las barras de escala son de 500 µm, las entradas tienen un tamaño de 50 µm. Cada imagen es representativa de tres ensayos de cristalización independientes.
Finally, when CaCl2/MgCl2 (1:1 ratio) were present in the crystallization assay formed scarce crystal structures and a thin film was present over all crystal preparations (Figure 5). At 40 mM CaCl2/MgCl2, an aragonite tablet was observed at 0.7 µg·µL-1 (Figure 5G), while at 100 mM CaCl2/MgCl2, only aragonite crystals were observed at 0.7 µg·µL-1 and 1.2 µg·µL-1 (Figure 5C, D), which were 100 µm size.
Figure 5 SEM micrographs of calcium carbonate crystals growth in vitro in the presence of CaCl2 and MgCl2 (40 and 100 mM), 100 mM NaHCO3 and Ps19 at increasing concentrations (µg·µL-1). First panel from left to right (A, E) Negative controls without Ps19; 100 mM CaCl2/MgCl2 in presence of (B) 0.2 µg·µL-1, (C) 0.7 µg·µL-1, (D) 1.2 µg·µL-1 Ps19; 40 mM CaCl2/MgCl2 in presence of (B) 0.2 µg·µL-1, (C) 0.7 µg·µL-1, (D) 1.2 µg·µL-1 Ps19; (I-K) protein alone without salt. Scale bars are 500 µm, inlets are 50 µm size. Each picture is representative of three independent crystallization assays.

Figure 5: Micrografías SEM del crecimiento in vitro de cristales de carbonato de calcio en presencia de CaCl2 y MgCl2 (40 y 100 mM), NaHCO3 100 mM y Ps19 a concentraciones crecientes (µg·µL-1). Primer panel de izquierda a derecha (A, E) Controles negativos sin Ps19; CaCl2/MgCl2 100 mM en presencia de (B) 0.2 µg·µL-1, (C) 0.7 µg·µL-1, (D) 1.2 µg·µL-1 Ps19; CaCl2/MgCl2 40 mM en presencia de (B) 0.2 µg·µL-1, (C) 0.7 µg·µL-1, (D) 1.2 µg·µL-1 Ps19; (I-K) proteína sola sin sal. Las barras de escala son de 500 µm, las entradas tienen un tamaño de 50 µm. Cada imagen es representativa de tres ensayos de cristalización independientes.
Raman analysis
Raman measurements confirmed the presence of CaCO3 polymorphs (Figure 6). The spectra show characteristics
peaks belonging to the internal vibration mode of
Figure 6 Raman spectra of calcite and vaterite formation by using Ps19 protein and MgCl2 as a cofactor. Calcium carbonate crystals growth in vitro in the presence of MgCl2 Na2CO3. (A) 100 mM and (B) 40 mM, respectively. Color line indicate salts preparations, red line: salt without protein added, black: salt in the presente of 0.2 µg·µL-1, blue: salt in the presence of 0.7 µg·µL-1, brown: salt in the presence of 1.2 µg·µL-1. Color arrows indicate the peaks for each salt preparation.
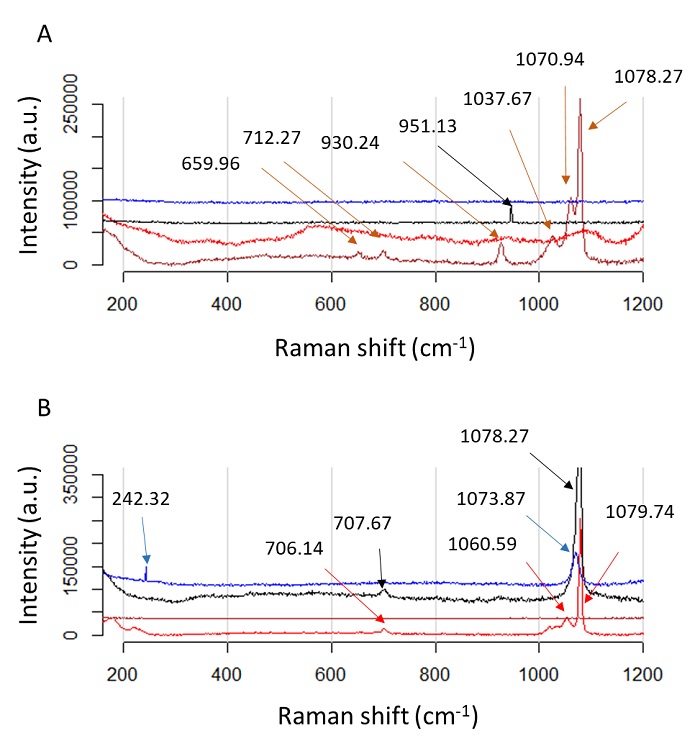
Figura 6: Espectros Raman de formación de calcita y vaterita usando proteína Ps19 y MgCl2 como cofactor. Crecimiento de cristales de carbonato de calcio in vitro en presencia de MgCl2 Na2CO3. (A) 100 mM y (B) 40 mM, respectivamente. La línea de color indica preparaciones de sales, línea roja: sal sin proteína añadida, negra: sal en presencia de 0.2 µg·µL-1, azul: sal en presencia de 0.7 µg·µL-1, marrón: sal en presencia de 1.2 µg·µL-1. Las flechas de color indican los picos para cada preparación de sal.
The Raman spectra of samples using CaCl2 as a cofactor are shown in
Figure 7. Here we observe a single
vibration mode in the region from 1060 to 1080 cm-1corresponding to
Figure 7 Raman spectra of carbonates deposition by using Ps19 protein and CaCl2 as a cofactor. Calcium carbonate crystals growth in vitro in the presente of CaCl2 NaHCO3 (A) 100 mM and (B) 40 mM, respectively. Color line indicate salts preparations, red line: salt without protein added, black: salt in the presente of 0.2 µg·µL-1, blue: salt in the presence of 0.7 µg·µL-1, brown: salt in the presence of 1.2 µg·µL-1. Color arrows indicate the peaks for each salt preparation.
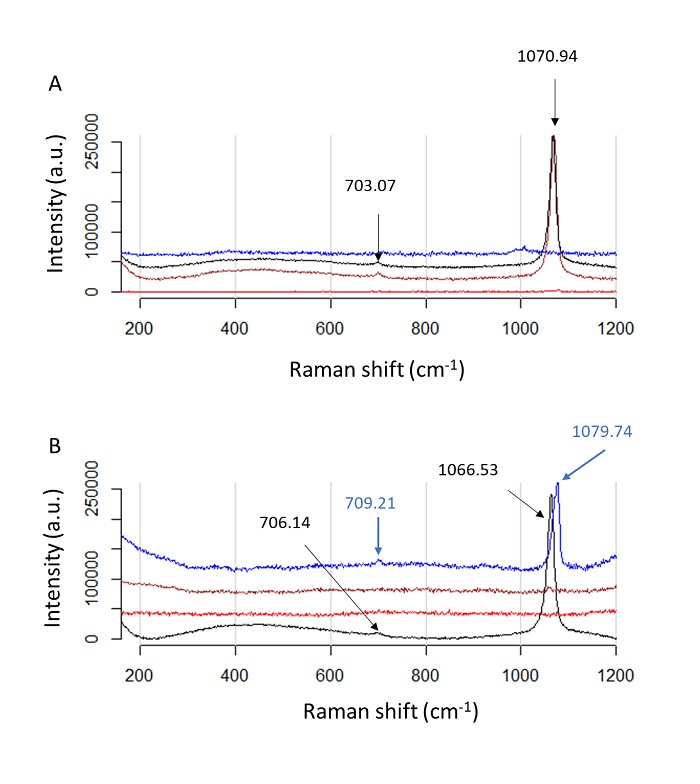
Figura 17: Espectros Raman de deposición de carbonatos usando proteína Ps19 y CaCl2 como cofactor. Crecimiento de cristales de carbonato de calcio in vitro en presencia de CaCl2 NaHCO3 (A) 100 mM y (B) 40 mM, respectivamente. La línea de color indica preparaciones de sales, línea roja: sal sin proteína añadida, negra: sal en presencia de 0.2 µg·µL-1, azul: sal en presencia de 0.7 µg·µL-1, marrón: sal en presencia de 1.2 µg·µL-1. Las flechas de color indican los picos para cada preparación de sal.
Figure 8 Raman spectra of ACC polymorphs by using Ps19 protein and MgSO4 as a cofactor. Calcium carbonate crystals growth in vitro in the presence of A) 100 mM MgSO4 Na2CO3 or B) 40 mM MgSO4 Na2CO3. Color line indicate salts preparations, red line: salt without protein added, black: salt in the presente of 0.2 µg·µL-1, blue: salt in the presence of 0.7 µg·µL-1, brown: salt in the presence of 1.2 µg·µL-1. Color arrows indicate the peaks for each salt preparation.

Figure 8: Espectros Raman de polimorfos de ACC usando proteína Ps19 y MgSO4 como cofactor. Crecimiento de cristales de carbonato de calcio in vitro en presencia de A) 100 mM de MgSO4 Na2CO3, B) 40 mM de MgSO4 Na2CO3 La línea de color indica preparaciones de sales, línea roja: sal sin proteína añadida, negra: sal en presencia de 0.2 µg·µL-1, azul: sal en presencia de 0.7 µg·µL-1, marrón: sal en presencia de 1.2 µg·µL-1. Las flechas de color indican los picos para cada preparación de sal.
Discussion
The shell growth in Mollusk has been described to start with the precipitation of ACC, which is known to surround aragonite platelets in gastropods (e.g. Haliotis laevigata) and bivalves (e.g. Pinctada margaritifera and Atrina rigida) indicating a nascent growing platelet (Nassif et al., 2005; Rousseau et al., 2009). ACC is a highly metastable phase, which is often found hydrated, and after dehydration, transforms to calcite, aragonite, or vaterite (Radha et al., 2010). Divalent ions have been found to affect the crystallization rates and pathways of ACC (Tobler et al., 2015). In Mollusk shells, Mg2+ ion is 2.55 % of their weight (Huang et al., 2018). This ion is known to stabilize ACC forming a complex (Mg-ACC) and reducing their reactivity to form polymorphs or calcite or aragonite, however, aragonite can be detectable since Mg2+ is known to suppress calcite growth (Davis et al., 2000). In our study, the mixture of the Ps19 with the saturated solution containing 40 and 100 mM MgSO4, respectively (Figure 4), resulted in the formation of imperfect spherical morphologies, which has been previously described (Xu et al., 2014). However, this was not observed when MgCl2 was used as a cofactor (Figure 2). This may be explained by the hydration capability of MgSO4 (heptahydrate) compared to MgCl2 (hexahydrated), acting as an inhibitor of calcite by occlusion of Mg inside the platelet, leading a less hydratated form of CaCO3 (Nielsen et al., 2016).
The transition of ACC to calcite and aragonite in Mollusk is modulated by Mg2+ ions and SMPs (Ma and Feng, 2015). In our study, Ps19 was able to induce well-formed aragonite platelets, being the bigger aragonite crystal those found at 100 mM MgCl2 (Figure 2). However, the inhibition observed at 40 mM MgCl2, and the less structured aragonite crystal at 100 mM MgCl2 when 1.2 µg·µL-1 Ps19 was used (Figure 2D, H), suggest that Ps19 can act as an inhibitor of crystal growth besides its role as a crystallization inductor, this dual role may be ion and protein concentration-dependent as has been suggested previously (Arroyo-Loranca et al., 2020). Similar results have been observed in Prismalin-14, Pif97, KRMP-3, and rPNU9 from P. fucata (Suzuki et al., 2004; Bahn et al., 2015; Liang et al., 2016; Kong et al., 2019).
SMPs related to prismatic layer growth of the shell have been found to form strictly calcite crystal at low CaCl2 concentration and to be able to inhibit aragonite platelet growth, e.g. Prisilkin-39 from P. fucata (Kong et al., 2009). However, Ps19 have been proved previously to produce calcite (Arroyo-Loranca et al., 2020) at low CaCl 2 . Interestingly, putative inhibition zones of crystal growth were observed in this study at high CaCl2 concentration and 0.7 µg·µL-1 of Ps19 (Figure 3C), a behavior also been observed in other SMPs such as rPif97 from P. fucata (Bahn et al., 2015), suggesting that calcite crystal deposition may be protein dose-dependent. The capability of Ps19 to produce calcite and aragonite using different ions suggest that Ps19 is associated to the formation of the prismatic and nacre layer in P. sterna, as has been described in other SMPs such as Lys-rich matrix protein family from P. fucata (Liang et al., 2016) and nacrein from the Pacific oyster Crassostrea gigas (Song et al., 2014).
Besides modulation by a single ion, the growth of calcite and aragonite is dependent on the Mg2+ and Ca2+ molar ratio (Loste et al., 2003). An increase in the Mg2+ molar ratio leads to the growth of a hydrated phase of CaCO3 at the surface of the solution, while at the base of the crystallization assay produces calcite and aragonite from 2:1, 3:1 and 4:1 of Mg:Ca (Loste et al., 2003). For Ps19, a molar ratio of 1:1 leads to the production of a thin film and scarce crystal growth with undefined structures at both concentration, 40 and 100 mM, however calcitic and aragonite structures were visible at 0.7 and 1.2 µg·µL-1 of Ps19, these results lead to the hypothesis that Ps19 may react with the salts and inhibit the crystal growth of either calcite or aragonite, and the crystals formed are the result of dehydrated spots in the crystallization assay, however further experiments are required corroborate this hypothesis.
Conclusions
In contrast to other SMPs described in Mollusk, which promote one kind of calcium carbonate polymorph (e.g. aragonite or calcite), Ps19 is a novel protein capable to induce calcite and aragonite crystals in a dose-dependent manner at different ion concentrations, acting as a promoter of aragonite in presence of MgCl2 and as a promoter of calcite in presence of CaCl2, suggesting that Ps19 may play an important role in the prismatic and nacre layer of the shell of P. sterna.