Introduction
The plant cell wall is composed of various polysaccharides, such as cellulose, hemicelluloses, and pectin. Among these, cellulose is the most abundant, constituting 30-50 % of the total cell wall dry mass, while hemicelluloses constitute 20 - 35 % (Pauly and Keegstra, 2008). Based on their main structure, hemicelluloses are grouped into xyloglucans, xylans, and mannans. Galactomannans (GM) are included in the latter group. These are multifunctional macromolecular carbohydrates formed by D-mannopyranose main chain linked by β (1→4) glycosidic bonds with D-galactopyranose branches linked to the mannan main chain by α (1→6) bonds (Figure 1). The mannose/galactose ratio (M/G) varies according to the source and extraction procedures used (Sharma et al., 2022).
Figure 1 Schematic representation of the chemical structure of galactomannans (George et al., 2019).
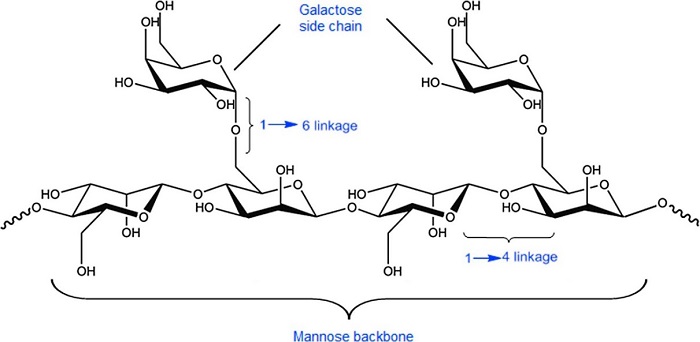
Figura 1: Representación esquemática de la estructura química de los galactomananos (George et al., 2019).
GM are commonly obtained from arboreal legume seeds, for example carob or locust bean (Ceratonia siliqua), tara (Caesalpinia spinosa), guar (Cyamopsis tetragonoloba L.), fenugreek (Trigonella spp) or mesquite seeds (Prosopis sp) (Wielinga, 2009; López-Franco et al., 2013). GM can be distinguished from each other by the M/G ratio which varies between 1.1 to 3.5 approximately. Carob gum has a M/G ratio of 3.5 (Fidan et al., 2020), tara gum 3.0 (Wu et al., 2015), guar and mesquite gum 1.5 (López-Franco et al., 2013; Liyanage et al., 2015), and fenugreek gum with 1.2 (Dhull et al., 2020).
GM are the product of photosynthesis, followed by additional biosynthetic modifications in nature, and have great industrial relevance (Sharma et al., 2022). The structural characteristics of these polysaccharides make them soluble in water at a wide range of temperatures, and chemically and biochemically reactive. Due to their large number of hydroxyl (-OH) groups and the absence of ionic charges in their structure, GM are susceptible to molecular changes (Geronço et al., 2021).
GM derivatives, e.g. obtained by hydrolysis, sulfation, or phosphorylation, provide biological and technological functionalities that are of interest in food, biomedical and other industries (Table 1). This review aims to describe the synthesis and structural analysis of chemically modified GM to improve their physicochemical properties and expand their industrial application.
Tabla 1: Estructura-función de derivados de galactomananos de diferentes fuentes.
Galactomannan source | Derivatized obtaining process | Functional properties | Reference |
Cyamoposis tetragonolobus L. | Hydrolysis | Hepatoprotective | Wu et al., 2019 |
Ceratonia siliqua | Hydrolysis | Immunomodulatory | Chen et al., 2018 |
Sesbania cannabina | Hydrolysis | Antioxidant and immunomodulatory | Tao et al., 2021 |
Caesalpinia pulcherrima | Hydrolysis | Dietary fiber | Buriti et al., 2014 |
Caesalpinia ferrea | Sulfation | Antiviric | Lopes et al., 2013 |
Adenanthera pavonina L., Caesalpinia ferrea Mart., and Dimorphandra gardneriana Tull | Sulfation | Antioxidant and antivirc | Mendes-Marques et al., 2015 |
Cyamoposis tetragonolobus L. | Esterification | Emulsifier | Sarkar and Singhal, 2011 |
Cyamoposis tetragonolobus L. | Esterification | Emulsifier | Cerqueira et al., 2019 |
Sesbania cannabina | Esterification | Film former | Liu et al., 2021 |
Cyamoposis tetragonolobus L. | Carboxymetylation | Binder | Wang et al., 2021 |
Cyamoposis tetragonolobus L. | Carboxymetylation | Thickener | Iqbal et al., 2020 |
Cassia obtusifolia | Carboxymetylation | Gelling agent | Verma et al., 2020 |
Cyamoposis tetragonolobus L. | Carboxymetylation | Gelling agent | Li et al., 2021 |
Cyamoposis tetragonolobus L. | Etherification | Corrosion inhibitor | Biswas et al., 2017 |
Cyamoposis tetragonolobus L. | Etherification | Corrosion inhibitor | Singh et al., 2021 |
Cyamoposis tetragonolobus L. | Phosphorilation | Antioxidant | Wang et al., 2014 |
Structure, physicochemical characteristics and applications of galactomannans
GM is a nonionic polysaccharide conformed by a linear chain of β-(1→4)-D-mannose with branches of α-(1→6)-D-galactose, where the M/G ratio and molecular weight is closely related to its structure-function (Wielinga and Meyhall, 2009). Previously it was thought that these galactose units are linked at regular intervals, that is, alternate in each mannose of the main chain. However, subsequent enzymatic degradation analysis, NMR spectroscopy and computer simulation suggested the irregularity of side chains in such a way that the galactose units are arranged in the form of random doublets and triplets along the main chain (Mudgil et al., 2014; Gupta and Variyar, 2018). Said random substitution of the galactose units leads to regions of high and low substitution in the mannan main chain (Figure 2). In galactose-poor regions, non-covalent interactions between polymer chains could take place, while in galactose-rich regions, this high substitution prevents the formation of more organized structures due to steric hindrance of the side chains, which also results in a high solubility in aqueous solutions (Saurabh et al., 2013).
Figure 2 Schematic representation of galactose (G) highly substituted and low substituted regions in the mannose backbone (M) (Gupta y Variyar, 2018).
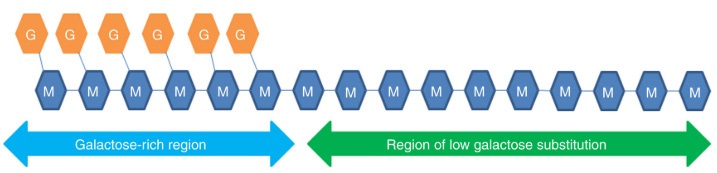
Figura 2: Representación esquemática de las regiones altamente sustituidas y poco sustituidas en galactosa (G) en la cadena principal de manosa (M) (Gupta y Variyar, 2018).
The GM molecular weight one of the highest among natural polysaccharides, it could be up to 2 x 106 Da, measured by size exclusion chromatography with multiangle laser light scattering (López-Franco et al., 2017; Gupta and Variyar, 2018). Due to the native characteristics of GM, it exhibits non-Newtonian behavior in solution and, like most polysaccharides with these characteristics, it has a pseudoplastic, or shear-thinning nature. This shear-thinning behavior of aqueous solutions increases with GM concentration and molecular weight. The apparent viscosity of these solutions demonstrates a decrease with an increasing shear rate. By increasing the shear rate, disruption predominates over formation of new entanglements; molecules align in the direction of the flow and consequently a decrease in viscosity is observed (Hussain et al., 2015). However, at very low and very high shear rates, GM solutions show Newtonian behavior (Whistler and Hymowitz, 1979).
Due to their hydrophilic character, GM have unique functional properties for various food uses. Among these are the water retention capacity, reduced evaporation rate, modification of the formation of ice crystals, regulation of rheological behavior and its emulsifying capacity (Gupta and Variyar, 2018). In this sense, the addition of GM in the preparation of white bread generally results in a greater absorption of water at the time of dough formation. Rodge et al. (2012) reported an 8 % increase in water absorption capacity with the addition of GM at 1 % in wheat flour; likewise, an increase in loan volume of 30 % was observed compared to control. This behavior can be attributed mainly to the hydrophilic nature of the polysaccharide and its capacity to retain water during baking, in such a way that said moisture evaporates through an increase in vapor pressure, resulting in an improvement in bread volume.
On the other hand, the use of GM as an additive for ice cream has been a strategy to prevent the development of crystals and as a texturizing agent during freezing, however, the use of this polysaccharide is only suitable for short-duration ice cream at high temperature. This, mainly because it has a short hydration time, in addition, the GM is thermodynamically incompatible with milk proteins of the food system, given the phase separation between both biopolymers due to hydrophilicity differences of the protein and GM (Mudgil and Barak 2014). Therefore, to avoid phase separation in this type of product, GM is generally added together with other hydrocolloids, such as k-carrageenan (BahramParvar et al., 2013; Javidi et al., 2016). Also, GM have been used in the preparation of low-fat yogurt with a high content of dietary fiber. Lee and Chang (2016) reported a yogurt preparation with improved rheological characteristics by adding GM, which resulted in reduced syneresis and improved texture with acceptable sensory characteristics comparable to control products.
Additionally, GM are part of the most popular and widely used hydrocolloids in the formulation of ketchup-type sauces, to prevent syneresis during freezing and provide stability in heating by cooking. Torbica et al. (2016) have previously reported the preparation of a ketchup-type sauce derived from tomato pomace, with acceptable sensory and textural qualities using GM and xanthan gum as thickeners, managing to reduce whey loss. Likewise, this hydrocolloid has been added in the formulation of mayonnaise and dressings, to reduce the dispersed phase (oil) and increase the water content in the emulsion to create low-fat products. However, due to its hydrophilic characteristics, the use of native GM is restricted the formulation of systems that contain water as a dispersant phase. The uses and potential applications of GM could expand with the knowledge of different synthetic routes of chemical modification applied to obtain changes on the structure-function relationship of polysaccharide.
Chemical modification of galactomannans
Hydrolyzed galactomannans
Hydrolysis, either by chemical methods or enzymatic reactions, is one of the most important and practical strategies for the modification of polysaccharides such as GM (Geronço et al., 2021). In the enzymatic method, the galactose side chains or the mannose main chain, can be hydrolyzed by α-galactosidase and β-mannanase, respectively, it is simple to control, and the reaction conditions are mild compared to chemical hydrolysis which is non-specific and highly aggressive (Ba et al., 2012). The GM functional properties are greatly influenced by their molecular weight, and based on this, the hydrolysis of the mannose main chain and galactose side chain results in a strategy for the modification of its viscoelastic properties (Zhou et al., 2016).
For this purpose, hydrochloric, sulfuric, and trifluoroacetic acids are used, in addition to the application of heat to accelerate the hydrolysis reaction of the side chains by mild conditions (Biermann, 1989). However, this type of hydrolysis results in a difficult elimination of acidic remnants with the probable exception of trifluoroacetic acid, which can be removed by rotary evaporation due to its volatility at not very high temperatures (Liu et al., 2020). Hence, research on obtainment and fractionation of GM hydrolysates has focused mainly on the use of enzymatic catalysis as a less complicated way to obtain mannooligosaccharides.
Wu et al. (2019) evaluated the hepatoprotective effect of GM partially hydrolyzed with β-mannanase from Rhizomucor miehei. The composition of these hydrolysates was 24.9 % (w/w) of mannooligosaccharides with a degree of polymerization of < 7. The average molecular weight of the hydrolyzed GM was 2.5 × 104 Da identified by gel permeation chromatography, the decrease of which was effective by enzymatic treatment. These hydrolyzed GM had a hepatoprotective effect in Kunming mice, by increasing the activity of the superoxide dismutase and glutathione peroxidase antioxidant enzymes. This event, in turn, decreased alcohol-induced lipid peroxidation, and thus the integrity and fluidity of cell membranes.
Similarly, Chen et al. (2018) hydrolyzed GM with 8 U/mL of a Pichia pastoris thermostable β-mannanase at different times of action on the substrate at 50 °C. Native GM had a higher molecular weight than the hydrolyzed ones with a significant decrease from 5.58 × 106 to 3.18 × 103 Da, demonstrating the presence of mannooligosaccharides after 24 h. These hydrolysates exhibited immunomodulatory activity by stimulating the production of TNF-α in RAW 264.7 cells, an important cytokine that intervenes in the inflammation and apoptosis processes.
Recently, hemicellulosic polysaccharides such as GM and their degradation products are gaining more attention due to their excellent in vitro and in vivo antioxidant enhancement and immunomodulatory activities. Tao et al. (2021) hydrolyzed GM from β-endo-mannanase of Trichoderma reesei and evaluated its antioxidant and immunomodulatory effect. GM hydrolysates showed a strong protective effect against induced oxidative stress, using a model of RAW 264.7 cells injured with hydrogen peroxide, as indicated by superoxide dismutase activity and malondialdehyde content.
Structurally, mannooligosaccharides continue to be only the repeating unit of GM in its native form, as its general composition and most of its physicochemical properties are not affected. For example, the FTIR spectrum is very similar to GM and their hydrolysates that show characteristic absorption bands of this polysaccharide as reported in the literature (Cerqueira et al., 2011; Mudgil et al., 2012). Furthermore, the partial hydrolysis of GM leads to a decrease in the polydispersity index by up to one-third, this is due to the general reduction of all molecular weight populations (Buriti et al., 2014). Partially hydrolyzed GM, as it does not suffer significant alterations, seems to be a potential alternative, increasing the availability of new food ingredients, as a source of dietary fiber.
Sulfated galactomannans
A promising derivatized structure of GM is the synthesis of their compounds that contain a sulfonic group (-SO3H) or their salt (-SO3Na). The addition of this functional group in a biopolymer macromolecule changes its physicochemical characteristics, increasing its solubility in water and its biodegradability (Kazachenko et al., 2020). The presence of the -SO3H group in the polysaccharide structure (Figure 3) provides important properties, since the sulfate group has a negative charge in a determined pH range to electrostatically bind to positively charged molecules. However, the synthesis of sulfated polysaccharides is a challenge due to the many stereocenters in their structure, the presence of similar functional groups, and the need to preserve the orientation of the glycosidic bonds. Therefore, from a chemical perspective, sulfated polymeric structures are synthesized by sulfating natural polysaccharides or polymeric analogs (Caputo et al., 2019).
Figure 3 Scheme of sulfation process of galactomannans (Muschin et al., 2016).
There are many challenges associated with polysaccharide sulfation, such as low -OH group reactivity, poor solubility in organic solvents, regioselectivity, degree of sulfation, and isolation of the derivatized polysaccharide. There are scarce solvents in which both the polysaccharide and the sulfated derivatized are soluble, and the reactions are not complete (Richter and Klemm, 2003). On the other hand, identifying and targeting a specific sulfation site is complicated due to the many -OH groups in the polysaccharide. Thus, protecting groups are required which increases the complexity, and decreases yield. Furthermore, sulfating multiple -OH on a single monosaccharide unit is complicated due to electrostatic repulsion between neighboring -SO3H adding a grade of complexity to the derivatization (Al-Horani and Desai, 2010).
Given the structure-function relationship of polysaccharides, the degree of sulfation becomes an important parameter to control, when synthesizing sulfated GM (Caputo et al., 2019). The chlorosulfonic acid and sulfuric acid are currently used in the sulfation process of GM as a sulfating reagent in presence of a strong base (pyridine or piperidine), and its subsequent isolation by converting it into sodium salt. In this sense, the use of chlorosulfonic acid is the most used route to obtain sulfated GM with different sulfation degrees. In contrast to these reagents, sulfamic acid is a stable non-hygroscopic crystalline substance with the reactivity like that of the -SO3 tertiary amine complex that in the presence of urea, which plays a role of the main catalyst, its sulfation occurs (Kuznetsov et al., 2018).
In this sense, Kazachenko et al. (2021) synthesized sulfated GM from sulfamic acid-urea complexes at different reaction times and temperatures. In this study they determined that at 80 °C and for 60 min, the highest possible degree of sulfation (1.67) is obtained by this synthetic route. Thus, from these milder conditions it is possible to have a more effective sulfation process from eco-friendly reagents than from the conventional chlorosulfonic acid route. Qin et al., (2020) evaluated a response surface methodology (RSM) study for the optimization of the sulfation process by varying the chlorosulfonic acid / pyridine ratio, temperature, and reaction time. This study provided optimal sulfation conditions for GM with a 66 % degree of sulfation, which were an acid chlorosulfonic / pyridine ratio of 3:1, reaction time of 4 h and reaction temperature of 40 °C. Two characteristic absorption peaks appeared in FTIR spectra at 1250 cm-1 and 806 cm-1, indicating sulfation of GM. The 13C-CP/MAS NMR spectra confirmed that the substitution position was mainly at C6 in sulfated GM.
Previously, Wang et al. (2014) optimized the sulfation process by varying the same parameters by RSM. In this study, a mathematical model was constructed where the optimal reaction conditions of GM were a chlorosulfonic acid / pyridine ratio of 1.27, reaction time of 120 min, and reaction temperature of 50.8 °C. Among the three reaction parameters studied, reaction time was the most significant factor to have an effect on the sulfation process, based on the slope gradient in the 3D response surface plots (Wang et al., 2014; Qin et al., 2020).
Lopes et al. (2013) sulfated GM with chlorosulfonic acid in pyridine/formamide to study the inhibition of herpes simplex virus (HSV-1) and poliovirus (PV-1). These authors reported an 8.7 % degree of sulfation obtained from three consecutive sulfation procedures. FTIR analysis demonstrated the presence of sulfated polysaccharides with a band at 1255 cm-1 which refers to the vibration of asymmetric stretching of the ester sulfate bond (S=O). These sulfated products inhibited HSV-1 and PV-1 with an IC50 of 405 μg/mL and 1.73 μg/mL, respectively, interfering various stages of viral replication, adsorption, and penetration in both cases.
Similarly, Mendes-Marques et al. (2015) sulfated GM with the same method for the inhibition of dengue virus, in this case they reported a 33 - 39 % degree of sulfation and mentioned the importance of this parameter in its activity. Confirmation of the sulfation process was performed by FTIR spectroscopic analysis with the presence of a band at 1259 cm-1 corresponding to the stretching vibrations of the S=O group mentioned above. Likewise, these derivatized polysaccharides presented a positive correlation between the degree of sulfation and the antiviral activity against the dengue virus DENV-2 and its antioxidant capacity.
Galactomannan fatty acid esters
Chemical modification has conventionally been used as a strategy to change the hydrophilicity of polysaccharides, through the formation of esters. The esterification of GM is carried out regioselectively, this is that the primary alcohol will always be more nucleophilic than the secondary alcohol in the polysaccharide structure (Kanelli and Topakas, 2017). Their respective rate constants for the primary and secondary alcohols do not change during the esterification process, where primary alcohol reacts faster than secondary alcohol, decreasing its concentration as the reaction progresses. Therefore, eventually, the reaction rates will become similar and the regioselectivity will decrease (Fox et al., 2011; Koschella et al., 2006).
Esterification generally involves the reaction of an alcohol with an acylating agent, such as vinyl carboxylates, acyl chlorides, acid anhydrides, as well as the carboxylic acids themselves. On this basis, carboxylic acids have been obtained by Fischer esterification conventionally by strong acid catalysis (concentrated H2SO4), or by in situ activation of the carboxylic group under mild conditions for the acylation of polysaccharides (Cumpstey, 2011). Previously, different synthetic routes have been reported to obtain GM esters, through both chemical and enzymatic routes, either using strong acids or strong bases to react with the acyl donor or using enzymes such as lipase or transferases to catalyze the reaction.
Fujioka et al. (2009) synthesized GM esters through the esterification of the succinate group in the polymer chains. They reacted native GM with succinic anhydride, using DMSO as a solvent and 4-dimethylaminopyridine as a catalyst. FTIR spectroscopy was used to demonstrate the presence of the ester group, showing absent absorption bands at 1735 cm-1 and 1550 cm-1 in the spectrum of the native GM indicating the esterification of the polysaccharide with a degree of substitution of 0.6. In another study, Shenoy and Melo (2010) esterified hydroxypropyl GM using acetic anhydride as donor acyl and pyridine as a catalyst, to be used as filler in unsaturated polyester compounds. The esterification of this polysaccharide leads to an increase in the hydrophobic character of the resulting polymer where the variation of the reaction time results in the formation of esterified GM with degrees of substitution of 0.58, 1.1, 1.8, and 3.0. The FTIR of the products showed an increase in the intensity of the carbonyl band obtained in the region of 1735 - 1750 cm-1 derived from the increase in the degree of substitution of the polymer.
On the other hand, Iqbal et al. (2020a) reported GM esters of aliphatic carboxylic acids of medium and long chain (C5-C18) from acyl chlorides, examining their physical properties concerning the length of the fatty acid chain and degree of substitution. The FTIR spectrum showed typical bands of a structurally native GM for all samples, added to the bands at 1754 cm-1 and 723 cm-1 corresponding to the C=O bond of the ester group and the oscillating vibrations of the -CH2 groups of the aliphatic chain, respectively. However, this type of reaction leads to polymer degradation and not very high degrees of substitution due to the use of aggressive catalysts with respect to pH.
An alternative to this process has been biocatalysts through the use of enzymes to modify the structure of polysaccharides, with the advantage that it is often chemo specific, which allows the synthesis of products with well-defined or stereo-specific structures (Cumpstey, 2011; Cheng and Gu, 2012). Some enzymes have a wide tolerance to the substrate and catalyze reactions in generic structures, hence, esterases, proteases, and lipase have been used to catalyze the formation of esters in polysaccharides, even being an unnatural function of the enzyme. This is because they do not differentiate the -OH groups, but the enzyme will tend to act on the -OH group (esterification) or ester (hydrolysis) that is more sterically accessible, that is, located in primary positions (Cumpstey, 2011). Enzymes normally require at least an amount of water to carry out their action correctly, being structurally unstable in non-aqueous media. However, water is not a good medium for ester synthesis, in these conditions is reversible. When the water is in excess, the chemical equilibrium of the reaction tends towards hydrolysis, so the degree of substitution of the products would be very low (Alissandratos et al., 2010).
On the other hand, polar solvents such as DMSO and DMF can remove essential catalytic water from the surface of enzymes, inactivating them and thus have lower hydrogen bonding capacity leading to higher enzymatic activity (Gu and Cheng, 2005). However, there are no well-established protocols for GM enzymatic esterification, unlike starch and cellulose, which are the most widely used for this chemical modification (Yang and Wang, 2003; Rajan et al., 2008). Most studies are mainly focused on their interaction with hydrophobic compounds and not on the application of GM esters.
In that context, Sarkar and Singhal (2011) elaborated GM succinate and oleate as microencapsulation material in O/W emulsions. The addition of these aliphatic chains leads to an improvement in its emulsifying capacity with low droplet size and viscosity, which makes it an important material for the protection of different food components. In another study, Cerqueira et al. (2019) elaborated GM acetate nanomicelles as carrier material for hydrophobic compounds such as curcumin. These modifications to the GM led to an encapsulation efficiency greater than 99 % and a ζ potential of -18.1 mV, indicating an effective charge of curcumin and repulsion between the nanomicelles to prevent them from agglomerating.
In contrast, in the esterification of GM for the addition of aliphatic chains, hydrophobic films of GM acetate have also been developed as a barrier system and protection against water in food packaging (Liu et al., 2021). In this work, the chemical modification provides a good hydrophobicity to the film with a contact angle of 107 ° and a decrease in its water vapor permeability properties. Thus, the synthesis of these amphiphilic compounds contributes to the development of new compounds for hydrophobic systems. In addition, it will be of vital importance when establishing the synthetic routes to produce GM esters and choose the appropriate compounds for the correct esterification of the polysaccharide.
Other derivatized galactomannans
Carboxymethyl galactomannan
Carboxymethyl galactomannan (CMG) is an anionic GM derivative with a high dissolving rate of viscosity and good stability. Various synthetic routes have been developed to obtain CMG (Figure 4). This is produced by reacting monochloroacetic acid or its sodium salt with deprotonated GM. A strong base, such as sodium hydroxide, is needed to activate the -OH group of GM for nucleophilic substitution (Cumpstey, 2011). Previous studies related to CMG preparation used organic solvents such as acetone, ethanol, isopropanol, and butanol as the medium (Gao and Grady, 2018). GM has been previously dissolved in water, however, the use of excess water in a ratio of approximately 50:1 generally has poor compatibility, because water can hydrolyze the alkylating agent (Dodi et al., 2011).
Figure 4 Synthesis of carboxymethyl galactomannan (CMG) of guar gum (Iqbal et al., 2020).
The synthesis of CMG is widely studied; Wang et al. (2021) obtained CMG as robust water-soluble binder for silicon anodes in lithium-ion batteries, and the 1H NMR spectrum shows that carboxymethyl was attached to position C-6. Additionally, in the FTIR spectrum, two new bands appear at 1602 cm-1 and 1411 cm-1 corresponding to the asymmetric and symmetric stretching vibrations of the COO- group respectively, which further confirms the carboxymethylation of GM. In another study, Iqbal et al. (2020b) synthesized carboxymethylated GM derivatives and their structural characteristics as a synthetic thickener for textile prints. These had a degree of substitution of 0.35, and the FTIR spectrum demonstrates the substitution of carboxymethyl in GM with the bands corresponding to the COO- vibrations that are symmetric or asymmetric at points 1429 cm-1 and 1615 cm-1, respectively.
The potential applications for CMG can be diverse, for example, Verma et al. (2020) evaluated the CMG pharmaceutical applications by making ionotropically gelled beads using sodium diclofenac as a model drug, optimizing its design by RSM. The formulation used was 2.85 % (w/v) CMG and 15 % (w/v) CaCl2 where the beads showed a loading efficiency of 95.41 % and a release of 93.32 % of diclofenac during 24 h. In another study, Li et al. (2021) fabricated polyelectrolytic gels of CMG and quaternary ammonium GM for the removal of dyes in water contaminated with Congo red and methylene blue as study models. These gels were pH and ion sensitive, with maximum adsorption capacities of 1441 and 94.52 mg/g of Congo red and methylene blue, respectively, mediated by electrostatic interactions.
Galactomannan alkyl ethers
Etherification of polysaccharides involves the reaction of the saccharide alcohol groups with an alkylating agent, these include alkyl halides (e.g. bromides, chlorides, and iodides), or less commonly, alkyl sulfonates. Typically, a strong base is used to deprotonate a saccharide alcohol to form an alkoxide ion that attacks the alkyl halide, through an SN2 mechanism to form a polysaccharide alkyl ether. Usually, the alcohol from which the alkoxide is derived can be used as solvent, but it improves the reaction performance if we use aprotic solvents such as DMF or DMSO (Cumpstey, 2011). Currently, derivatized GM alkyl ethers have been reported, such as: methyl, butyl, benzyl, ethyl acrylate and 2-hydroxypropyl tri-methylammonium chloride (HPTAC) GM ethers (Kazachenko et al., 2022a).
The synthesis of methyl and benzyl GM ether has been widely studied, for instance, Mohammed and Ragab (2021) synthesized these GM ethers in a DMSO/paraformaldehyde system using dimethyl sulfate and benzyl chloride as alkylating agents in presence of sodium hydroxide as a catalyst. The correct etherification of the polysaccharide was determined from FTIR shown new peaks at 1114 cm-1 and 1056 cm-1 indicating C-O stretching vibration of functional group of ether for methyl GM and benzyl GM respectively. In another study, Kazachenko et al. (2022b) synthesized GM butyl ether using bromobutane as an alkylating agent in the presence of sodium hydroxide as a catalyst. Etherification was confirmed by FTIR showing absorption bands at 1077, 879, 815 and 796 cm-1, corresponding to the vibrations of the ether bond of the -C-O-C- groups.
The potential applications of GM alkyl ethers have not yet been as extensively studied. The etherification of HPTAC has been used as a strategy for obtaining cationic GM as a corrosion inhibitor (Biswas et al., 2017). Similarly, Singh et al. (2021) synthesized galactomannan ethyl acrylate ether as a corrosion inhibitor on steel under acidic conditions. However, it is necessary to address the possible applications of all types of GM alkyl ethers including those synthesized from uncharged alkyl groups to obtain a more diverse picture in the use of this type of derivatized GM.
Phosphorylated galactomannan
The production of phosphorylated GM is carried out by different synthetic routes: phosphoric acid, phosphoric anhydride, phosphorous trichloride (Figure 5), or dimethyl phosphate (Suflet et al., 2006). Furthermore, H3PO4 and H3PO3 have been usually used as phosphorylating reagents at higher temperatures, generally leading to a low degree of substitution (0.26, phosphate content 4.05 %) and polysaccharide degradation (Wang et al., 2013). The applications for phosphorylated GM have not been so widely studied, being its antioxidant capacity and its relationship with DS one of the few reports found. In this sense, Wang et al. (2014) synthesized phosphorylated GM with POCl3 to evaluate the effect of the degree of substitution with respect to its conformation in solution. The degree of substitution varied from 0.35 to 0.52, and the FTIR spectrum showed new peaks around 1250 cm-1 that describe an asymmetric P=O stretching vibration, a shoulder at 1050 cm-1 corresponding to the P-OH bond, which is attributed to the presence of phosphorylated GM. Finally, the antioxidant capacity was increased to a higher DS of 0.52, which indicates that this derivatized polysaccharide could be effective in scavenging radicals in vitro.
Figure 5 Schematic route for obtaining phosphorylated GM by POCl3 (Wang et al., 2014).

Figura 5: Ruta esquemática para obtener galactomananos fosforilados mediante POCl3 (Wang et al., 2014).
Conclusions
This review highlighted the different chemical modifications and structural characteristics of different derivatized products of GM. Naturally, polysaccharides like these already possess characteristic physicochemical properties such as solubility, swelling, and viscosity inherent in their origin, which are not always desirable for other types of unconventional applications. The chemical modification of GM, on the other hand, leads to structures with new characteristics that can compete with different synthetic excipients already available on the market. This in turn provides excipients that can be less toxic than various synthetic polymers. The future of formulations design using natural polymers and their different chemical modifications is in a strong position. Therefore, natural and derivatized polymers can compete if scientists continue to explore materials and modify them for various formulation designs.