Introduction
Maize (Zea mays L.) belongs to the group of cereals with worldwide importance, due to its applications in animal consumption, including human. In 2018, world maize production reached 1147.62 million tons, the highest amount among the main cereals (FAO, 2020). In Mexico, during 2018, around 7.3 million hectares were cultivated with this cereal, with a total production of 27.1 million tons (SIAP-SADER, 2020). On the other hand, maize production in the main agricultural zones of northwestern Mexico, such as the Yaqui Valley, reached 449,000 tons in the year 2018, while in 2019 its production increased up to 567,000 tons (SIAP-SADER, 2020). However, this global and national food demand has led to the indiscriminate use of agrochemicals, in areas of intensive agriculture such as the Yaqui Valley, where high amounts of nitrogen and phosphate fertilizers have been used, as well as pesticides, to improve the yield of several crops such as wheat and maize (McCullough and Matson, 2016). Nevertheless, the excessive use of agrochemicals contributes to the contamination of soils and underground water supplies, leading to potential health risks and environmental degradation (Meza-Montenegro et al., 2012). Thus, the need fo the development of sustainable strategies for food production, to diminish the damage caused by the use of large quantities of agrochemicals, is evident.
The use of soil microorganisms (mainly bacteria) as inoculants with beneficial traits for plant development and soil health represents an attractive alternative to conventional agriculture, named Plant Growth Promoting Bacteria (PGPB) (Valenzuela-Aragon et al., 2019). PGPB includes a large group of microorganisms that can be found in the rhizosphere and bulk soil, as well as within and on aerial plant tissues (de Souza et al., 2015). PGPB applied to plants, either on soil or seeds, improve plant crops growth and protection against diseases and abiotic stress, by improving the biological nitrogen fixation, solubilization of phosphates, reduction of stress through diverse enzyme production, synthesis of phytohormones, production of siderophores, among others (Glick, 2012; de Souza et al., 2015). Thus, the most studied genera of PGPB are Acetobacter, Acinetobacter, Alcaligenes, Arthrobacter, Azospirillum, Azotobacter, Bacillus, Bradyrhizobium, Burkholderia, Enterobacter, Gluconacetobacter, Klebsiella, Methylobacterium, Ochrobactrum, Paenibacillus, Pantoea, Pseudomonas, Serratia, Stenotrophomonas, and Rhizobium (Glick, 2012; Ahemad and Kibret, 2014). Several studies have demonstrated the ability of these bacterial genera to promote the growth of different crops such as rice, tomato, wheat, maize, chickpea, peanuts, among others, showing significant changes in physiological parameters, i.e., dry weight, chlorophyll content, and plant height (Kumar et al., 2014; Sharon et al., 2016).
PGPB can be classified as native or indigenous when those are endemic to the site where they will be applied and as exogenous when they are isolated from other locations. Several research, mainly related to pollutants biodegradation, have shown that for an exogenous PGPB to properly perform its functions, not only must adapt to the local region edaphoclimatic conditions but also there must be compatibility with the native microorganisms (i.e. to avoid competence for nutrients or a specific niche). For example, Festa et al. (2016) found that the inoculation of exogenous microorganisms caused significant changes in the diversity of the microbial community, which can compromise the subsequent functionality of the soil. On the other hand, Kaur and Reddy (2013) demonstrated that native bacterial strains associated with maize crop have the ability to facilitate microorganism-plant interaction in order to enhance plant growth parameters, such as shoot length, germination, shoot, and root dry weight, as well as an increase in crop yield, by the production of phytohormones, siderophores, and solubilization of phosphates. However, the bacterial inoculant success in the field is not assured, due to the influence of several important factors such as climatic conditions, native microbiota, and its formulation (Dutta and Podile, 2010; de los Santos-Villalobos et al., 2018). The introduction of exogenous PGPB could induce at least a transient perturbation of the equilibrium of soil microbial communities, this may be undesirable if important native species are lost, affecting subsequent crops (Trabelsi and Mhamdi, 2013). However, this effect could be buffered by ecosystem resilience, which is driven by the level of diversity and interactions of the plant-soil-biota (Kennedy, 1999).
At present, research works on native microbiota in the Yaqui Valley are very scarce (de los Santos-Villalobos et al., 2018), even when this is the birthplace of the Green Revolution, and there are no microbial inoculants developed with native strains from this area, which have adapted to the edaphoclimatic conditions (high temperatures, alkaline and low content of organic matter). The aim of this study was to identify and characterize promising native PGPB associated with maize in the Yaqui Valley, as well as to quantify their inoculating impact on morphometric parameters of maize, grown under greenhouse conditions.
Materials and methods
Bacterial strains
The studied bacterial strains (22A67, 13B41, 22B45, and 31B11) were selected according to their ability to promote in vitro maize growth (unpublished data). These strains are cryopreserved in Colección de Microorganismos Edáficos y Endófitos Nativos (COLMENA, http://www.itson.mx/COLMENA) (de los Santos-Villalobos et al., 2018). Glycerol-frozen (-80 °C) bacterial strains were pre-cultured in Petri dishes containing Nutrient Agar (NA) as a culture medium, and incubated for 24 h at 28 °C.
Morphological and molecular characterization
All bacterial strains were cultured in Nutrient Agar (NA) and incubated for 24 h at 28 °C to characterize them by macro (color, shape, margins, and elevation) and microscopical (Gram stains and shape) traits. DNA from each strain axenic bacterial culture was extracted using the UltraClean Microbial DNA Isolation Kit (QIAGEN ®), the DNA integrity analyzed by 1% agarose electrophoresis while the quality and concentration by spectrophotometry (NanoMicro-Spectrophotometer Jenway ®). To amplify the 16S rRNA gene (1500bp) we used the FD1 (5’-CCGAATTCGTCGACAACAGAGTTTGATCCTGGCTCAG-3’) and RD1 (5’-CCCGGGATCCAAGCTTAAGGAGGTGATCCAGCC-3’) primers (Weisburg et al., 1991), in a 50 µL PCR reaction mixture containing 100 ng DNA, 1X PCR buffer, 0.2 µM primers, and 4U MyTaq DNA polymerase (Bioline). The PCR cycle was as follows: 5 min at 95 °C, followed by 30 cycles of 30 s at 95 °C, 40 s at 57 °C, 2 min at 72 °C, and a final elongation step of 5 min at 72 °C. PCR products were verified by electrophoresis gel agarose/TAE (2 %), and the purified amplicons sequenced by the Sanger platform. The edition and analysis of the obtained DNA sequences was with the software FinchTV 1.4.0 (Geospiza, Seattle, WA), CLC Sequence viewer 7 (QIAGEN, Denmark), and BLAST (NCBI, http://www.ncbi.nlm.nih.gov) used to identify the reported sequence with greater similarity to the studied bacterial strains. The phylogenetic analysis was generated with the MEGA software version 7.0 (Kumar et al., 2016) using the neighbor-joining method, the evolutionary distances computed using the Tamura 3-parameter model. The partial sequences of the 16S rRNA gene were deposited in the Genbank database (MG807394, MG807395, MG807396, MG807397 accession numbers).
Plant growth promoting traits by bacterial strain
Indoles production. For quantification of indoles production, 1x103 Colony Forming Units (CFU) of each bacterial strain were inoculated, in triplicate, into 30 mL of nutrient broth (NB), supplemented with 100 ppm of tryptophan, and incubated in a rotary shaker (Yamato R) at 150 rpm and 28 °C for 3 days. After incubation, the bacterial culture was centrifuged at 10,000 rpm for 10 min, and 1 mL of the supernatant was placed in a 1.5 mL Eppendorf tube and centrifuged again at 8000 rpm for 10 min according to de los Santos-Villalobos et al. (2013). Quantification of indoles was performed by the spectrophotometric method using Salkowski reagent according to Glickmann and Dessaux (1995). The concentrations of produced indoles were estimated with a standard curve of indole-3-acetic acid (SigmaR), in a range of 2-50 ppm. The tests were carried out in triplicate.
Phosphate solubilization. Each bacterial strain (1x103 CFU) was inoculated, in triplicate, on Petri dishes containing Pikovskaya (PVK) medium and incubated at 25 °C for 10 days. The PVK medium was composed of 2 solutions (salts and bromophenol blue Stock) according to Onyia and Anyanwu (2013). The ability of the studied strains to solubilize phosphorus (P) was observed by a transparent halo around the bacterial colony. The Phosphate solubilization index (PSI) was obtained according to Sharon et al. (2016), following the Equation 1:
Equation 1. Phosphate solubilization index or Siderophore production index
Siderophore production. To determine the ability of studied bacterial strains to produce siderophores, 1x103 CFU of each strain was inoculated on Petri dishes containing chrome azurol S medium (CAS), and incubated at 28 °C for 10 days, by three independent replicates (García-Meléndez et al., 2017). The CAS medium preparation was following the method described by Alexander and Zuberer (1991). Strains that showed a transparent or colored halo around the bacterial colony were positive for this test. The siderophore production index was obtained following the Equation 1.
1-Aminocyclopropane-1-Carboxylate(ACC) deaminase activity. The ability of strains to produce ACC deaminase was screened in Petri dishes containing minimal medium according to Dworking and Foster (1958). 1x103 CFU of each strain was inoculated, by triplicate, in Petri dishes containing minimal media with ACC as its sole nitrogen source; then incubated at 28 °C for ٣ days. Bacterial growth in the minimal medium was considered positive in ACC deaminase activity (nutrient medium was used as control).
In all the characterization tests mentioned above, Bacillus sp. P41B2 whose plant growth promoting traits are known was used as technical control.
Maize growth promotion by studied PGPB under greenhouse conditions
Bacterial production. A bacterial pre-inoculum was prepared for each strain (1x105 UFC), in tubes with 25 mL of Nutrient Broth (NB), incubated at 120 rpm for 24 h at 28 °C. Then, the pre-inoculum optical density adjusted to OD630=0.5 (Valenzuela-Aragon et al., 2019). For the bacterial inoculum, 1 mL of pre-inoculum was inoculated to 3 L of NB, and incubated for 72 h, at 28 °C with shaking at 120 rpm. The bacterial suspension was pelleted by centrifugation for 15 min at 4000 rpm, washed twice and finally re-suspended in sterile distilled water. The bacterial concentration (CFU/mL) was adjusted to 1x109 CFU/mL.
Greenhouse assay. The greenhouse assay was carried out in 4 L pots under greenhouse conditions at the Instituto Nacional de Investigaciones Forestales, Agrícolas y Pecuarias (INIFAP) located at Cajeme, Sonora (27°N, 110°W). The max/min temperature during assay was 30 °C/٨ °C and 12-hour photoperiod. CARIBU® (ASGROW®) commercial hybrid maize was used. A non-sterile mixture of perlite/agricultural soil/Pro-Mix GTX® substrate (1:3:2) was used as a plant substrate. The soil mixture was classified as clay-loam, with the following characteristics: organic matter = 5.48 %, pH in CaCl2=7.07, electrical conductivity = 2.903 dS/m, total salts = 1858 ppm, N = 217 Kg/Ha, P = 15 ppm. In each pot, 300 mL (1x109 CFU/mL) of each bacterial strain were applied separately to each plant, 10 replicates per treatment and an un-inoculated control were established. Plants were kept well-watered, and no chemical fertilizers were applied during the assay (Parra-Cota et al., 2014).
Measurement of maize growth parameters. Data collection was weekly over 6 weeks and at the end of the assay (42 days after sowing). The first measurement was 14 days after sowing between stages V3 and V4 of maize growth. Plant height, leaf chlorophyll level (SPAD 502 Minolta®), and leaves number were measured, and at the end, height, plant and root dry weight, and stem thickness.
Statistical analysis
A completely randomized design was applied in all experimental assays with three replicates per treatment, in the greenhouse assay a replicate was constituted by 10. Data analysis was by one-way analysis of variance (ANOVA) test and Tukey-Kramer test (p=0.05), using Statgraphics Centurion XVI.II (Statgraphics Technologies, Inc., Virginia).
Results and discussion
Morphological and molecular characterization of studied bacterial strains
The macroscopic traits of all strains were similar in shape (circular), margin (entire), and elevation (flat) but different colors. Cell shape varied from coccus for strain 22A67 to rod-shaped for the other strains. Similarly, Gram stain was different between the bacterial strains (Table 1). Based on the 16S rRNA gene sequence phylogenetic analysis (Figure 1), the identifications were as follows: strain 22A67 as Advenella incenata (MG807395, accession number), strain 22B45 as Pantoea dispersa (MG807397), strain 31B11 as Rhizobium pusense (MG807394), and the strain 13B41 (MG807396) as Bacillus sp. In the latter, it was not possible to determine the species due to the sequences high similarity between different species of the genus Bacillus.
Table 1 Morphological characteristics of studied PGPB.
Tabla 1. Caracteristicas morfologicas de las BPCV
estudiadas.
Strain | Microscopic traits | Microscopic traits | ||
Macroscopic traits | Color | Cell shape | Gram reaction | |
13B41 | Circular with entire margin and flat | Brown | Rod-shaped | Positive |
22A67 | Circular with entire margin and flat | Light Brown | Coccus | Negative |
22B45 | Circular with entire margin and flat | Yellow | Rod-shaped | Negative |
31B11 | Circular with entire margin and flat | Brown | Rod-shaped | Negative |
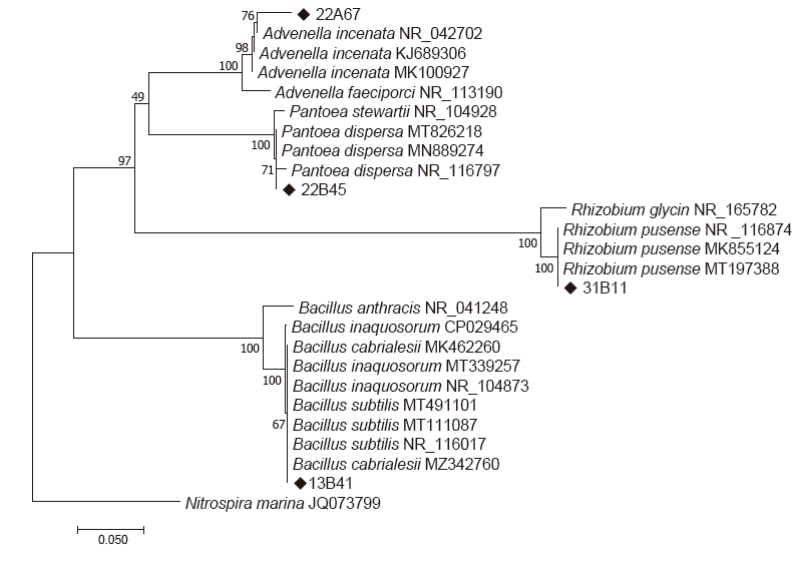
Figure 1 Phylogenetic Tree inferred using the Neighbor-Joining method. Numbers at nodes
indicate values of bootstrap support based on an análisis of 1,000
resampled data sets. Nitrospira marina was used as
an outgroup sequence. The scale bar indicates the number of base
substitutions per site.
Figura 1. Arbol filogenetico inferido mediante el
metodo de Neighbor- Joining. Los numeros en los
nodos indican valores de soporte de Bootstrap basados en un analisis
de 1000 conjuntos de datos remuestreados. Se utilizo
Nitrospira marina como secuencia externa. La
barra de escala indica el numero de sustituciones de bases por
sitio.
The four genera to which the studied bacterial strains belong have been previously described as PGPB. The Bacillus genus was first reported by Cohn in 1872 and currently includes more than 377 species, which are widely distributed in very diverse ecosystems such as aquatic, terrestrial, and those with extreme conditions (Villarreal-Delgado et al., 2018; de los Santos Villalobos et al., 2019). Several Bacillus species have been identified as plant growth-promoting bacteria since they suppress pathogens and/or improve plant development (Sansinenea, 2019). Bacteria of the Rhizobium genus have been extensively studied for their ability to fix nitrogen in symbiosis with legumes, but Rhizobium is also a very good root colonizer of non-legumes and can produce plant hormones, siderophores, solubilize phosphorus, and exhibits adverse effects to plant pathogens (Qureshi et al., 2013). At present, many research works have demonstrated the beneficial effects of Rhizobium inoculation to cereals, like maize, in terms of improved growth and yield (Mehboob et al., 2012; Hussain et al., 2016).
Pantoea is a relatively recent genus, proposed by Gavini et al. (1989). Pantoea spp. have been isolated from plants surfaces, within plant tissues, seeds, fruits, rhizosphere, water, soil, from humans and other animals; they are rarely considered as pathogens (Chauhan et al., 2015). Various studies have reported strains of Pantoea as biological control agents and as growth promoter in crops such as corn, wheat, apple, cotton, barley, and potato (Montañez et al., 2012; Kaur and Reddy, 2013; Chauhan et al., 2015). Whereas the genera mentioned above has been widely studied, mainly Bacillus and Rhizobium, Advenella is a poorly studied genus, and reports of their role as PGPB are scarce, but these coincide in their potential role as phosphate solubilizers in soils with crops such as maize and wheat (Espinosa-Victoria et al., 2009; Singh et al., 2014; Wang et al., 2020).
Plant growth promoting traits by studied bacterial strains
In order to have a better understanding of plant-microorganism interactions, it is important to study the PGPB potential mechanisms of action. The four studied strains were able to produce indoles, i.e., Pantoea dispersa 22B45 showed the higher production of indoles (11 ppm), followed by Bacillus sp. 13B41 (4.0 ppm), Rhizobium pusense 31B11 (3.8 ppm), and Advenella incenata 22A67 (2.8 ppm) (Table 2). Indole acetic acid (IAA) is generally considered as the most important native auxin (Glick, 2012); several species of bacteria are capable to produce this class of phytohormones, where their production vary depending on growing conditions, stage of development, availability of nutrients, among others (Duca et al., 2014). The bacteria indoles production can affect the hormonal balance in plants and, therefore, can influence their growth, mainly increasing the total root surface, which leads to an enhanced mineral uptake from the soil (Spaepen and Vanderleyden, 2011). For example, in different studies, two native Pantoea strains associated with maize showed the ability to synthesize indoles in 121.6 ppm and 93 ppm, respectively (Montañez et al., 2012; Kaur and Reddy, 2013). Similarly, Naveed et al. (2014) demonstrated that indoles-producing bacteria application can positively affect different growth parameters of maize, such as root dry weight and plant height, with increments of 43% and 8%, respectively, compared to un-inoculated control.
Table 2 Plant growth promotion traits by studied PGPB, under in vitro conditions.
Tabla 2. Rasgos de promocion del crecimiento vegetal de
las PGPB estudiadas, en condiciones in
vitro.
Strain | Indoles production (ppm) | Phosphate solubilization Index (PSI) | Siderophore production Index (SPI) | ACC deaminase activity |
---|---|---|---|---|
Bacillus sp. 13B41 | 4.0 b | 1.25 ab | 1.88 b | - |
Advenella incenata 22A67 | 2.8 a | 1.58 b | 1.40 a | - |
Pantoea dispersa 22B45 | 11.4 c | 1.42 ab | 1.29 a | + |
Rhizobium pusense 31B11 | 3.8 b | 1.15 a | 1.30 a | + |
Bacillus sp. P41B2* | 3.1ab | 1.28ab | 1.37a | - |
On the other hand, Advenella incenata 22A67 showed a high level of phosphate solubilization in PVK medium, similar to an Advenella strain with phytase activity reported by Singh (2014). Pantoea dispersa 22B45, Bacillus 13B41, and Rhizobium pusense 31B11, also showed the ability to solubilize phosphates, but at a lower level (Table 2). P is an important nutrient of plants due to it is involved in different functions, such as photosynthesis, respiration, and nutrient movement (Viruel et al., 2014). There is enough P in soil, but only a small amount is available for plants, which limits their growth (Viruel et al., 2014). The P availability depends on its solubility, which is influenced by the activity of roots and microorganisms in the soil, mainly through the production of organic acids and alternate mechanisms as the production of chelating compounds and secretion of phytase enzymes (Pande et al., 2017). Thus, the studied strains can be classified as phosphate-solubilizing bacteria capable of solubilizing phosphate from an insoluble tricalcium source, which has shown a positive impact on biomass weights of tomato and maize crops, compared to un-inoculated plants (Montañez et al., 2012; Sharon et al., 2016).
Another important micronutrient for plants is iron (Fe), which influences several important biological processes such as photosynthesis, respiration, and chlorophyll synthesis (Kobayashi and Nishizawa, 2012). However, like phosphorus, this micronutrient has low availability in soils. Soil bacteria can overcome this Fe limitation by chelating molecules called siderophores (Villarreal-Delgado et al., 2018), which production capacity provides an advantage against soil-borne pathogens (de los Santos-Villalobos et al., 2012). Every studied strains showed the ability to produce siderophores, observing the higher value (SPI=1.88) for Bacillus sp. 13B41, significantly different compared to the rest of the strains (Table 2). In addition, bacterial genera like Pantoea, Advenella, Bacillus, and Rhizobium have shown this biochemical trait, also related to the growth promotion effect in maize and wheat cultivars (Kaur and Reddy, 2013; Kumar et al., 2014; Singh et al., 2014).
The activity of the enzyme 1-aminocyclopropane-1-carboxylate (ACC) deaminase, is of a plant growth promoter mechanism by microorganisms, which regulate plant levels of ethylene (Glick, 2012). Ethylene is produced endogenously by plants and participates in the regulation of all processes for their growth, however, under environmental stress, its production is accelerated, negatively affecting root growth, and therefore, the development of the whole plant (de Souza et al., 2015). Here, Pantoea dispersa 22B45 and Rhizobium pusense 31B11 showed ACC activity, like that reported by Shahzad et al. (2008), where strains of the genus Pantoea were identified with ACC activity and used to increase the yield of chickpea of up to 54 % compared to the un-inoculated control. Hussain et al. (2016) showed that by the inoculation of Rhizobium in maize, plants grew under drought stress conditions, obtaining an increase of 16 % and 30 % in dry biomass of root and shoot, respectively, compared to un-inoculated plants.
Maize growth promotion by isolated bacterial strains, under greenhouse conditions
In this study, all inoculated treatments showed a significant difference (p≤0.05) in plant chlorophyll level compared to the un-inoculated treatment, increased up to 40 % by Bacillus sp., 47 % by Rhizobium pusense, 41 % by Pantoea dispersa, and 39% by Advenella incenata at 42 days after sowing (Figure 1). However, there was no significant difference between plants inoculated with the studied strains. At 14 days after sowing, plant chlorophyll levels increased from 13 % to 20 % for all inoculated plants compared to the un-inoculated treatment (Figure 2). Increased chlorophyll content by the application of PGPB might be attributed to the enhanced root size of plants by phytohormones production, as well as the solubilization and mobilization of nutrients (Qasim et al., 2017). Furthermore, the leaf chlorophyll level is markedly related to the photosynthetic capacity, which implicates a better nutrient cycling, indicating an improvement of plant growth (Croft et al., 2016).
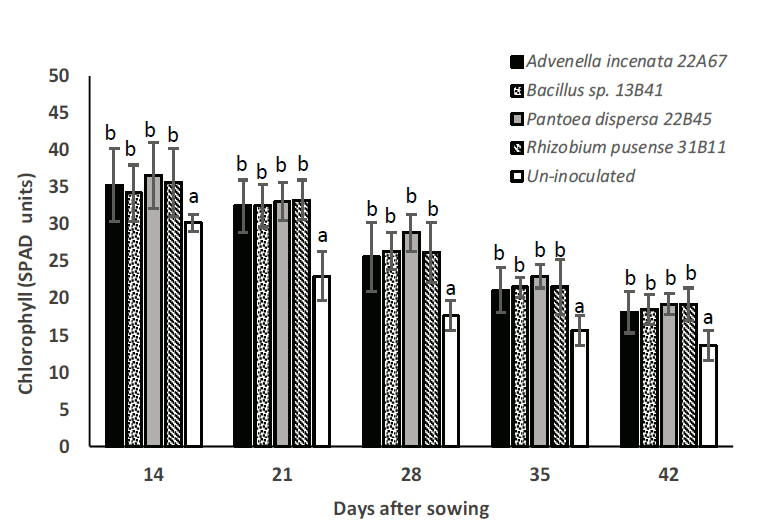
Figure 2 Chlorophyll level of maize plants inoculated by each studied strain and un-inoculated.
Distinct letters indicate significant differences (p<0.05, Tukey
test), n=10.
Figura 2. Nivel de clorofila de plantas de maiz no
inoculadas e inoculadas por cada cepa estudiada. Las letras
distintas indican diferencias significativas (p <0.05, prueba de
Tukey), n=10.
Height of inoculated plants showed significant differences at 21 days after sowing, where strain Advenella incenata 22A67 showed a height of 37 cm, being different to the rest of the treatments [30.2 cm, 30.9 cm, 31.5 cm, and 28.2 cm for Pantoea dispersa 22B45, Rhizobium pusense 31B11, Bacillus sp. 13B41, and un-inoculated control, respectively]. The plant height on days 28, 35, and 42 after sowing, showed significant increases for all treatments (40, 51, and 35 %, respectively), compared to the un-inoculated treatment, and there was no difference between plants inoculated with the studied strains, as indicated in Figure 3.
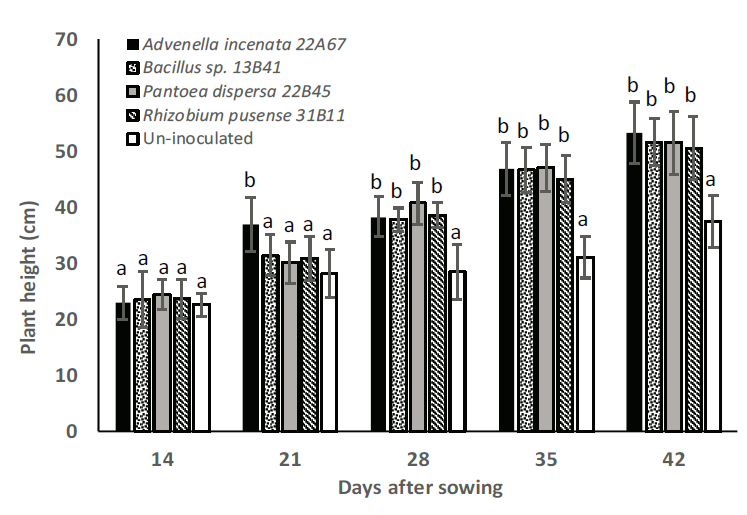
Figure 3 Height of maize plants inoculated with each strain. Distinct letters indicate
significant differences (p<0.05, Tukey Test), n=10.
Figura 3. Altura de las plantas de maiz inoculadas con
cada cepa. Las letras distintas indican diferencias significativas
(p <0.05, prueba de Tukey), n=10.
In addition, the stem thickness showed a significant increment from 63% to 73% by studied bacterial strains (Table 3), compared to the un-inoculated treatment. This increment may relate to the accumulation of reserves in the stem during the vegetative stages, which could later increase the production of grains (Setter and Meller 1984; Seyed Sharifi et al., 2017). On the other hand, the maize inoculation of the studied strains increased de number of leaves, but Pantoea dispersa 22B45 increased the number of leaves by 33 %, presenting at least one more leaf per plant than the rest of the strains and un-inoculated control (Table 3). Agbodjato et al. (2016) and Abiala et al. (2015) also reported an increase in the number of leaves in maize plants inoculated with PGPB under greenhouse conditions; in the first study the effect was observed by the inoculation of P. fluorescens; while in the second, the individual inoculation of Bacillus, Lysinibacillus, Citrobacter and Enterobacter caused this effect in plants. The increase in the number of leaves is clear evidence of the growth promoting effect generated by the PGPB.
Table 3 Effect of studied PGPB on maize growth under greenhouse conditions, at vegetative
stage V8.
Tabla 3. Efecto de las PGPB estudiadas sobre el
crecimiento del maiz en condiciones de invernadero, en la etapa
vegetativa V8.
PGPB | Stem thickness (mm) | Number of leaves | Root dry weight (g) | Shoot dry weight (g) | ||||
Advenella incenata 22A67 | 13.7 ± | 2.50b | 8 ± | 0.79b | 5.45 ± | 1.88b | 7.90 ± | 3.21b |
Bacillus sp. 13B41 | 13.6 ± | 1.71b | 8 ± | 0.74b | 5.99 ± | 2.10b | 7.61 ± | 2.13b |
Pantoea dispersa 22B45 | 13.6 ± | 1.51b | 9 ± | 0.52c | 6.34 ± | 1.85b | 7.89 ± | 2.52b |
Rhizobium pusense 31B11 | 12.9 ± | 2.38b | 8 ± | 0.82b | 5.32 ± | 1.94b | 6.99 ± | 3.11b |
Un-inoculated | 7.9 ± | 1.37a | 6 ± | 0.48a | 2.67 ± | 0.81a | 2.03 ± | 0.76a |
Maize plants inoculated with Pantoea dispersa 22B45 also showed the highest root dry weight, 6.34 g, followed by maize inoculated with Bacillus sp. 13B41, Rhizobium pusense 31B11, Advenella incenata 22A67, and un-inoculated plants, as shown in Table 3. All inoculated plants showed a higher shoot dry weight and root dry weight compared to the un-inoculated treatment, with increments from 244 to 289 %, and 99 to 137 %, respectively (Table 3). Silva et al. (2016) reported the capacity of Pantoea and Bacillus to increase shoot and root dry matter by 28 % and 18 %, respectively, in maize plants at 40 days after emergence compared to un-inoculated control. Qureshi et al. (2013) showed that the genus Rhizobium is an effective plant growth promoter in maize cultivars, due to its ability to improve the photosynthetic rate, up to 21 %. In addition, an increase in plant height and dry matter production was observed, these traits were attributed to the ability of Rhizobium strains to produce phytohormones. On the other hand, although there are few reports about maize growth promotion by Advenella (Espinoza-Victoria et al., 2009; Singh et al., 2014), this study shows that it can improve maize plant height from the first stages of development (Figure 4), besides increasing the dry matter content of maize.
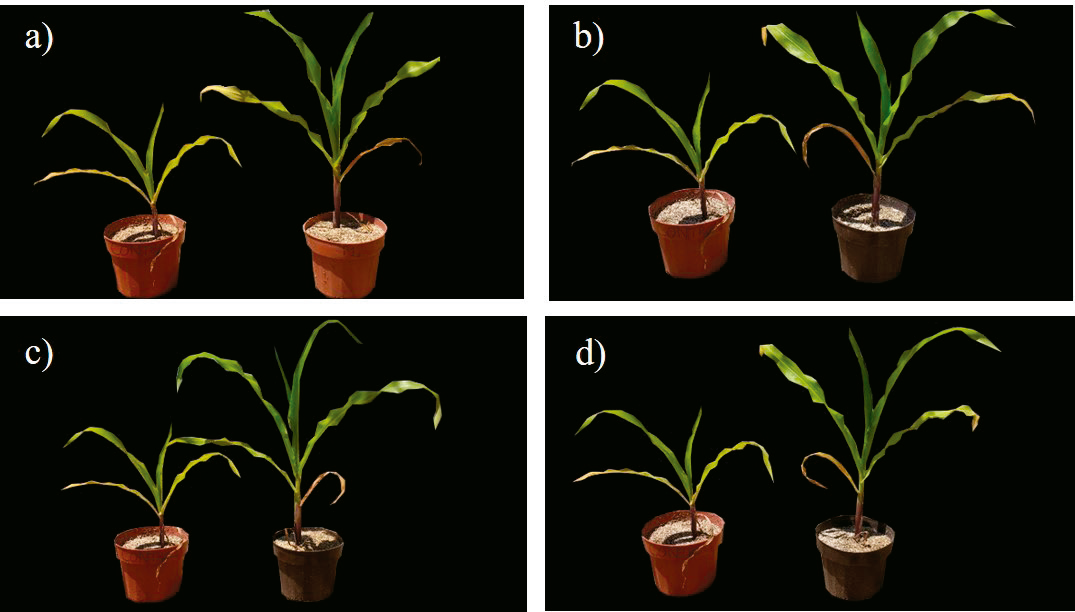
Figure 4 Effect of PGPB inoculation (right) on maize plant growth compared with un-inoculated
plants (left). a) Advenella
incenata 22A67, b) Bacillus
sp. 13B41, c) Rhizobium pusense
31B11, d) Pantoea dispersa 22B45.
Figura 4. Efecto de la inoculacion de PGPB (derecha)
sobre el crecimiento de la planta de maiz en comparacion con plantas
no inoculadas (izquierda). a) Advenella
incenata 22A67, b)
Bacillus sp. 13B41, c)
Rhizobium pusense 31B11,
d) Pantoea dispersa 22B45.
Conclusion
Plant growth-promoting bacterial strains studied here showed significant plant growth promoting traits in maize plants (root weight, plant height, number of leaves, stem thickness), under greenhouse conditions compared to the un-inoculated plants; however, these beneficial effects were similar among strains. Thus, Rhizobium pusense 31B11, Pantoea dispersa 22B45, Bacillus sp. 13B41, and Advenella incenata 22A67, isolated from the Yaqui Valley, are promising bacterial strains to be evaluated in field trials in this area or in others with similar edaphoclimatic conditions, to test their ability to improve grain yield in maize, so that they can be used as native microbial inoculants for sustainable agriculture.