Introduction
Tumors of the central nervous system (CNS) are the most common solid tumors during childhood and are among the top two causes of cancer-related death in children in Mexico and worldwide1-3. These brain tumors are classified according to histology and tumor location. Gliomas arise from glial precursor cells present in the brain and spinal cord and are named according to their clinicopathologic and histologic subtype: astrocytomas originate from astrocytes, oligodendroglial tumors from oligodendrocytes, and mixed gliomas from a mix of oligodendrocytes, astrocytes, and ependymal cells. Significant experimental evidence shows that neural stem cells, glial progenitor cells, oligodendrocyte progenitor cells, and possibly differentiated astrocytes can serve as cells of origin for astrocytoma4,5. This type of tumor is the most commonly diagnosed type of glioma in children4. In Mexico, 32% of the diagnosed brain tumors correspond to astrocytoma1-3.
In 2007, the World Health Organization (WHO) published a series of guidelines to grade the CNS tumors6. Thus, histopathological characteristics observed in hematoxylin- and eosin-stained sections, immunohistochemistry of lineage-associated proteins, and ultrastructural characteristics have been used for grading the pediatric astrocytomas into four grades (Table 1), leading to a lot of confusion and doubts among pathologists as the differentiation level varies between grades7.
Table 1 The WHO classification of astrocytoma (2016)a
Astrocytic tumors | Histologic grade | 2016 WHO classification | ||||
---|---|---|---|---|---|---|
I | II | III | IV | |||
Subependymal giant cell astrocytoma | X | Other astrocytic tumors | ||||
Pilocytic astrocytoma | X | |||||
Pilomyxoid astrocytomab | X | |||||
Pleomorphic xanthoastrocytoma | X | |||||
Diffuse astrocytoma | X | Diffuse astrocytoma, IDH-mutant | Diffuse astrocytic tumors | |||
Anaplastic astrocytoma | X | Anaplastic astrocytoma, IDH-mutant | ||||
Glioblastoma | X | Glioblastoma, IDH–wild-type | ||||
Giant cell glioblastoma | X | Glioblastoma, IDH-mutant Diffuse midline glioma, H3 K27M-mutant | ||||
Gliosarcoma | X |
WHO, World Health Organization; IDH, isocitrate dehydrogenase; H3 K27M, histone H3 K27M.
aAdapted from Louis et al., 2007 and 20166,10. This integrative classification includes the grading system used by the 2007 guidelines and the genetic driving mutation incorporated in 2016. The children specific H3 K27M entity is added in the current classification system.
bIn 2016, the WHO suggested not grading the pilomyxoid variant until further studies clarify its behavior.
Recently, the WHO published an updated grading system that considers the genetic basis of tumorigenesis and histological features6,8. In this classification, diffuse astrocytoma, anaplastic astrocytoma, and glioblastoma are clustered in the diffuse astrocytic tumor category. Within this grading system, genetic driver mutations are included in these categories. Therefore, diffuse astrocytic tumors are further classified by the presence or absence of mutations in the isocitrate dehydrogenase 1/2 (IDH1/IDH2) (isocitrate dehydrogenase [IDH] and nicotinamide adenine dinucleotide phosphate [NADP+] 1 and 2, respectively) and H3.3 histone A (H3F3A) genes. Meanwhile, low-grade astrocytomas are grouped into the other astrocytic tumor categories (Table 1)7,9,10; thus, genotyping is not required as low-grade astrocytomas do not harbor these driver mutations.
The IDH1 and IDH2 proteins in the cytosol and mitochondria generate reduced NADPH from NADP+ by catalyzing the oxidative decarboxylation of isocitrate into alpha-ketoglutarate (a-KG) outside of the Krebs cycle. IDH gene mutations in one allele (heterozygous mutation) produce a switch from arginine to histidine at amino acid residue 132 (R132H) that changes the function of the enzymes, which, in turn, produce 2-hydroxyglutarate, a possible oncometabolite, instead of NADPH. IDH1 mutations occur more commonly in glioma than IDH2 mutations, which undergo a similar missense mutation at arginine 172 (R172H)11-14.
H3F3A may bear mutations that change lysine to methionine at position 27 (K27M) or glycine to valine or arginine at position 34 (G34R/V). These two mutations, detected by whole-genome sequencing, are seen in 30% of pediatric glioblastoma. However, the incidence of these mutations increases to 95% in high-grade glioblastoma from the midline location (80 and 15% of high-grade glioblastoma bearing K27M and G34R mutation, respectively)15,16.
This upgraded classification system elicited a layered diagnosis with histological classification. The WHO grades with molecular information are reported as integrated diagnosis, improving diagnostic accuracy, patient management, and treatment response due to targeted therapies. However, it will also impose some technological constrains as not all the diagnosis centers may have access to genetic diagnostic tools (i.e., deoxyribonucleic acid [DNA] sequencing)7,8. Hence, it is essential to establish simple, cost-effective, and sensitive mutation scanning methods in clinical laboratories.
Sanger DNA sequencing has been widely used as the gold standard for clinical DNA analyses. However, it presents a low sensitivity (15-20% limit of mutant allele detection), emphasizing the need for a simple, cost-effective, and sensitive methods for mutation scanning in clinical laboratories as a routine practice for tumor sample analyses. The DNA melting analysis (DMA) of TaqMan probes has been claimed to fulfill such criteria. It has been used by Botezatu et al. and Huang et al. for the identification of mutations in the phosphatidylinositol-4,5-bisphosphate 3-kinase catalytic subunit alpha (PIK3CA), KRAS (KRAS proto-oncogene GTPase), NRAS (NRAS proto-oncogene GTPase), and BRAF (B-Raf proto-oncogene serine/threonine kinase) genes17-19. The theoretical basis of the method is the dependence of the melting temperature of the double DNA helix on its length, base composition (GC content), sequence, and the degree of complementarity of the strands. An increase in the fluorescence of a self-quenching fluorescence probe, like TaqMan probes, has been shown not only when it is cleaved by Taq DNA polymerase in the polymerase chain reaction (PCR) but also when it is hybridized with the DNA used as a template (in both cases, the fluorophore and the quencher became spatially separated)18,20. Therefore, the TaqMan probe dissociation from a non-mutated (KO) or WT DNA will produce a specific melting temperature that can be scored by the drop of fluorescence (as the fluorophore and the quencher become more prone to contact each other upon dissociation from the template DNA). Meanwhile, the presence of a single mutation in the template DNA will produce a shift of this melting temperature.
Therefore, the analysis of the melting temperature of a TaqMan probe can be used to identify the WT or KO status of the genes of interest. In addition, this assay allows using TaqMan probes in a closed-tube format, first performing asymmetric PCR by adding more concentration either of the forward or reverse primer (to accumulate single-stranded DNA) and then the analysis of the melting temperature of the TaqMan probes17-19.
To incorporate a molecular classification system that enables a more precise tumor classification as suggested by the WHO in 2016, we have evaluated the genotyping of pediatric astrocytomas with the DMA of TaqMan probes coupled to asymmetric PCR.
Methods
DNA isolation
Astrocytoma biopsies were obtained from the Neurosurgery Department of the Hospital Infantil de Mexico Federico Gomez under the informed consent of patients and their parents. All the experimental procedures have been approved by the scientific, ethical, and biosafety committees of the hospital (project numbers HIM/2017/104/SSA-1420 and HIM/2016/096/SSA-1289). Template DNA was isolated from brain tumor samples described in table 2 using the AllPrep DNA/RNA/miRNA Universal Kit (QIAGEN). DNA concentration and integrity were evaluated with the NanoDrop system (Thermo Scientific). Only DNA samples with high quality were used for both the DMA-TaqMan method and Sanger sequencing.
Table 2 Classification of diffuse astrocytic tumors by DNA Sanger sequencing
Astrocytoma-grade (local ID) | R132 in IDH1 | R172 in IDH2 | K27 and G34 in H3F3A |
---|---|---|---|
Pilomixoid astrocytoma-II (M17) | Wild type | Wild type | NA |
Diffuse astrocytoma-II (CM15) | Wild type | Wild type | NA |
Diffuse astrocytoma-II (CM39) | Wild type | Wild type | NA |
Pilomixoid astrocytoma-II (CM75) | Wild type | Wild type | NA |
Anaplastic astrocytoma-III (M29) | Wild type | Wild type | NA |
Anaplastic astrocytoma-III (CM54) | Wild type | Wild type | NA |
Anaplastic astrocytoma-III (M69) | Wild type | Wild type | NA |
Glioblastoma-IV (M9) | Wild type | Wild type | Wild type |
Glioblastoma-IV (M10) | Wild type | Wild type | Wild type |
Glioblastoma-IV (M49) | Wild type | Wild type | Wild type |
Glioblastoma-IV (CM8) | Wild type | Wild type | Wild type |
Tumor biopsies were classified by the Pathology Department of the Hospital Infantil de México Federico Gómez using genotyping by Sanger sequencing. The codons used for genotyping of IDH1, IDH2, and H3F3A and their genetic status in the tumor biopsies are displayed in single table columns.
ID, identification; NA, not applicable (as the genetic status is not used for classification); IDH1, isocitrate dehydrogenase 1; IDH2, isocitrate dehydrogenase 2; H3F3A, H3.3 histone A; DNA, deoxyribonucleic acid.
Primer and TaqMan probe design
To design primers pairs and TaqMan probes, we followed previous reports17-19. Briefly, three primer pairs for the IDH1, IDH2, and H3F3A genes (from the Human genes hg38 assembly, in the UCSC Genome Browser21) were designed using the Primer-Blast tool22. In addition, a TaqMan probe for each amplified gene segment was designed to have the nucleotide matching the possible mutation in the middle of the probe (Table 3). The same primers were used for the DMA of the TaqMan probes method and Sanger sequencing.
Table 3 Primers and probes used for mutation scanning by dissociation curve
Genomic sequence to be amplified | Codon with a mutation to be detected | Forward (F) and reverse (R) primers (Tm and CG%) Sense (s) or antisense (as) Taqman probes | Sequence |
---|---|---|---|
180 bp in exon 2 and intron 2 in IDH1 | R132 | IDH1-F (59ºC, 55%) | 5’ atattctgggtggcacggtc 3’ |
IDH1-R (58ºC, 41%) | 5’ aagttggaaatttctgggccat 3’ | ||
Probe IDH1 (as) | [FAM]ccataagcatgacgacctatgatgat[BHQ-1] | ||
152 bp in exon 4 and intron 4 in IDH2 | R172 | IDH2-F (59ºC, 50%) | 5’ tctgcaaaaacatcccacgc 3’ |
IDH2-R (59ºC, 60%) | 5’ caagaggatggctaggcgag 3’ | ||
Probe IDH2 (s) | [TAMRA]atcaccattggcaggcacgcccatgg[BHQ-2] | ||
180 bp in exon 1 in H3F3A | K27 and G34 | H3F3A-F (59ºC, 50%) | 5’ tggctcgtacaaagcagact 3’ |
H3F3A-R (55ºC, 36%) | 5’ tgtggatacatacaagagagacttt 3’ | ||
Probe H3F3A,K27 (as) | [Cy5]agggcgcactcttgcgagcggctttt[BHQ-3] | ||
Probe H3F3A,G34 (s) | [HEX]gcgccctctactggaggggtgaagaa[BHQ-1] |
Specific primers were designed to amplify three genomic regions (in IDH1, IDH2, and H3F3A genes) that bear possible mutations, as well as long TaqMan probes for each amplicon. Each TaqMan has different fluorophores plus their specific signal quenchers. The same primers were used for the DNA melting analysis (DMA)-TaqMan method and Sanger sequencing.
Tm, melting temperature; GC%, guanine-cytosine content; bp, base pairs; IDH1, isocitrate dehydrogenase 1; IDH2, isocitrate dehydrogenase 2; H3F3A, H3.3 histone A; FAM: carboxyfluorescein; BHQ: black hole quencher™ family; TAMRA: carboxytetramethylrhodamine; Cy5: cyanine-5; HEX: hexachlorofluorescein phosphoramidite
PCR
To set up PCR conditions for specific amplification of the desired regions, we performed PCRs with magnesium chloride and hybridization temperature gradients. We detected specific amplification parameters of the three amplicons under 1 mM MgCl2 and 61°C (Fig. 1A).

Figure 1 A: PCR standardization with magnesium chloride and hybridization temperature gradients. The PCR parameters of 1 mM MgCl2 and 61°C displayed specific amplification of the desired amplicons in IDH1, IDH2, and H3F3A genes (asterisks). B: The specificity of the PCR primers when using DNA as a template, as negative control reactions (with no DNA) were included for each amplicon. MWM: molecular weight marker; PCR: polymerase chain reaction; IDH1: isocitrate dehydrogenase 1; IDH2: isocitrate dehydrogenase 2; H3F3A: H3.3 histone A; DNA: deoxyribonucleic acid.
The specificity of the PCR primers was verified with the next reactions and settings: each 20 ml volume reaction contained 1x amplification buffer, 10 mM dNTPs, 1 mM MgCl2, 1 U Taq DNA polymerase (Thermo Scientific), a primer pair (0.5 mM each one), and 50 ng of DNA template. The PCR protocol started with a denaturation step for 3 min at 95°C, followed by 45 cycles at 95°C for 30 s, 61°C for 30 s, and 72°C for 20 s, with a final cycle at 72°C for 10 min. PCR reactions with no DNA were included for each amplicon as negative controls (Fig. 1B).
DMA of TaqMan probes in asymmetric PCR
Each 25 ml volume reaction contained 1x reaction buffer, 200 mM dNTPs, 1 mM MgCl2, 1 U Taq DNA polymerase (Thermo Scientific), 0.2 mM TaqMan probe, 500 ng of DNA template, and pair of specific primers with a 10-fold concentration difference of one of the primers (0.04 mM/0.4 mM). Real-time PCR protocol started with a denaturation step for 3 min at 95°C, followed by 55 cycles at 95°C for 30 s, 61°C for 30 s, and 72°C for 20 s, and fluorescence acquisition at 62°C. The MxPro-Mx3000P software (version 4) was used to collect and analyze amplification and melting data from the Stratagene Mx3005P real-time PCR detection system (Agilent Technologies).
Sanger sequencing and alignment of sequences
DNA regions to be sequenced were amplified using the same primers described in table 3. PCR reactions with specific amplification of the desired amplicons were purified with the MinElute PCR Purification Kit (QIAGEN). We used 20 ml of purified PCR reactions and 5 ml of the specific primer (10 mM) for Sanger sequencing at the Sequencing Core Facility (INMEGEN, Mexico). Sequencing results were delivered after 2 days. All the sequenced samples displayed enough quality for subsequent alignment (Table 2). To detect KO or WT DNA sequences, we performed a pairwise sequence alignment23 between the respective reference DNA (Table 3) and the sequence retrieved from Sanger sequencing.
Results
Genotyping of IDH1, IDH2, and H3K27M/G34 by Sanger sequencing
With the primers described in table 3, we amplified and purified the regions of interest and sequenced them by Sanger technology. Four diffuse astrocytomas (Grade II), three anaplastic astrocytomas (Grade III), and four glioblastomas (Grade IV) were sequenced, and all of them displayed the WT sequence for all the tested genes (Table 2).
Evaluation of DMA of the IDH1 TaqMan probe on IDH1-WT samples
We evaluated the melting temperature of the IDH1-TaqMan probe to identify the gene status of a bona fide WT template from pilocytic astrocytoma. A criterion that must be fulfilled is that the amplification of the template must be successful in order to do the melting temperature value more reliable19. Thus, we focused on melting temperature curves from PCR reactions that displayed amplification signals during the respective cycles of the real-time PCR. Under this scenario, only 14% of the PCR reactions (3/28) displayed amplification plots (Figs. 2A, C, E, G, and Table 4). The predicted melting temperature for a WT template is 63.7°C. However, the observed melting temperatures were 69.7, 92.2, and 92.2°C, respectively (Figs. 2B, D, F, H, 3A and B and Table 4). Furthermore, to test the method with another WT template, we evaluated the IDH1-TaqMan probe with a template from a diffuse astrocytoma IDH1-WT and observed that the melting temperature curve peaked at 65.6°C (Figs. 2H and 3A and Table 4).
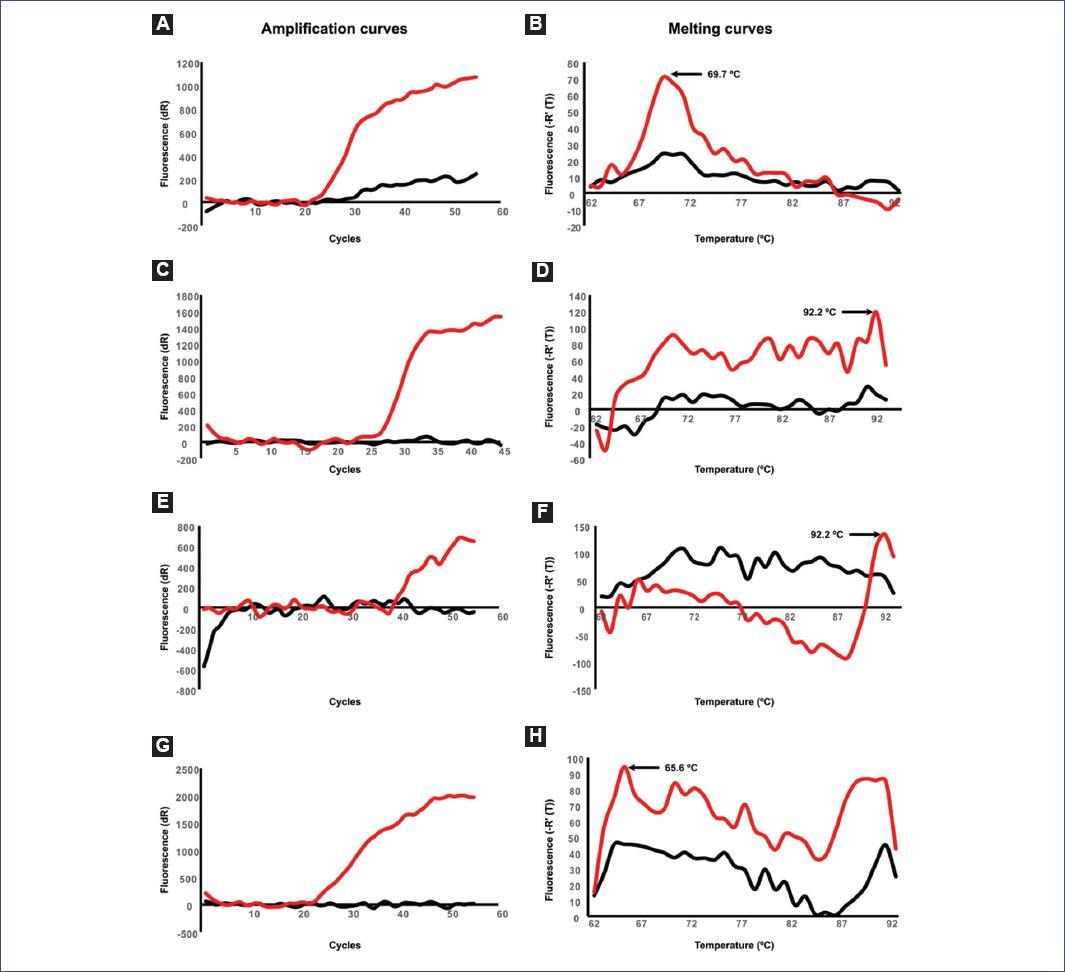
Figure 2 A, C, E, G: Amplification curves. B, D, F, H: Negative derivative melting curves for four different asymmetric PCRs. Specific primers and TaqMan probe for IDH1 (displayed in Table 2) were used to evaluate the melting curves of four different wild-type samples. The red lines indicate the fluorescence of the TaqMan probe during amplification and melting curve assessment. Peaks and values of the melting curves are displayed in each one of the respective panels. NTCs are shown in black lines. PCR: polymerase chain reaction; IDH1: isocitrate dehydrogenase 1; NTC: non-template controls.
Table 4 DNA melting analysis (DMA) of TaqMan probes with asymmetric PCR
Tested codon | Predicted T (°C) | Observed Tm (°C) | Success ratio for asymmetric PCR (success/trials) |
---|---|---|---|
R132 in IDH1 | WT: 63.7 | 69.7* | 14% (4/29) |
92.2* | |||
92.2* | |||
65.6** | |||
KO: 61.9 | NA | NA | |
R172 in IDH2 | WT: 74.5 | NO | 0% (0/7) |
KO: 72.4 | NA | NA | |
K27 and G34 in H3F3A | K27-WT: 76 | NO | 0% (0/7) |
K27-KO: 76.1 | NA | NA | |
G34-WT: 72.4 | NO | 0% (0/5) | |
G34-KO: 70.4 | NA | NA |
Predicted and observed melting temperatures of the gene-specific TaqMan probes for IDH1, IDH2, and H3F3A genes. Information on biological replicates and the success ratio is also displayed. NO, not observed (no data was obtained); NA, not assessed; IDH1, isocitrate dehydrogenase 1; IDH2, isocitrate dehydrogenase 2; H3F3A, H3.3 histone A; PCR, polymerase chain reaction; Tm, melting temperature; WT, wild type; KO, mutated.The values correspond to the melting curve when using DNA from a pilocytic astrocytoma* or a diffuse astrocytoma**. KO biopsies have not been assessed (NA) as the variation of melting temperatures in WT samples exceeds the shift that a single mutation would produce. Predicted melting temperatures were calculated with the melting temperature (Tm) calculation Tool available at the Biophp.org project (http://www.biophp.org/).
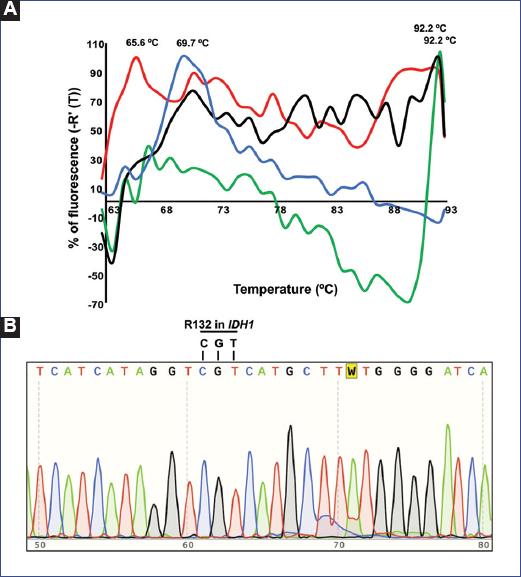
Figure 3 A: Overlapped negative derivative melting curves for four different asymmetric PCRs for the specific primers and TaqMan probe for IDH1. The negative derivate fluorescence is expressed as a percentage to make it comparable across samples. B: Chromatogram form Sanger sequencing showing the sequence of a diffuse astrocytoma IDH1-WT and its alignment to the reference sequence in the codon arginine 132 (R132). PCR: polymerase chain reaction; IDH1: isocitrate dehydrogenase 1; WT: wild type.
Evaluation of melting temperature for IDH2 and H3K27/G34 TaqMan probe
We tested the specific primers and TaqMan probes for IDH2 and H3K27/G34 using DNA obtained from a diffuse astrocytoma (II) – IDH1-WT, IDH2-WT, and H3K27M/G34-WT. Despite having obtained a good amplification efficiency and high-quality Sanger sequencing with the specific primers, the asymmetric real-time PCR with the TaqMan probes did not display any amplification plots nor melting curves (Table 4).
Discussion
The DMA of TaqMan probes in asymmetric PCR has been used to identify mutations in the PIK3CA, KRAS, NRAS, and BRAF genes in lung cancer, colon cancer, and melanoma17-19. Here, we tried to set up this melting analysis for the genotyping of pediatric astrocytomas by identifying specific mutations in the IDH1, IDH2, and H3F3A genes. As proof of principle, we used the IDH1-TaqMan probe to identify the WT status of IDH1 in two different WT DNA templates (pilocytic and diffuse astrocytoma). We obtained four melting temperature values that ranged from 65.6°C to 92.2°C. This broad variation in temperature is higher than the expected shift of 2-3°C if the mutation was present. Besides, only four out of 29 reactions displayed amplification of the DNA template, and amplification was observed only in one of the two technical duplicates of these four asymmetric PCR reactions (data not shown). As mentioned above, the successful amplification of the DNA template is an important parameter to do the melting temperature value more reliable19. Therefore, we conclude that the broadened values of the dissociation temperatures of the IDH1-TaqMan probe cannot be used as a reliable method for identifying a WT scenario. Albeit testing this TaqMan probe using DNA templates that harbor the mutations is still necessary, we speculate than the KO status of IDH1 will not be identified by this analysis as the broad range of melting temperatures will mask the temperature shift induced by the presence of the mutation on the template.
Finally, when we used the TaqMan probes for IDH2 and K27/G34 in H3F3A, amplification plots were not obtained. Although more standardizations are required for these genes, we concluded that a significant variation in melting curves and long-time consumption makes this genotyping assay method not reliable nor efficient, at least for IDH1. Meanwhile, Sanger sequencing was faster and more reliable to detect the gene status in all the sequenced samples.
Here, we show that the DMA of TaqMan probes in asymmetric PCR was not reproducible nor reliable. We conclude that Sanger sequencing or alternative next-generation sequencing will continue being the gold standard genotyping method.