Introduction
Exercise is one of the main factors by which a balance is maintained in the body, generating physiological and emotional health. This practice has been related _I to the reduction of morbidity and mortality rates in chronic degenerative diseases. Indicating that exercising reduces the risk of developing heart disease, diabetes, colon cancer, high blood pressure, among others (Colberg et al., 2016; Figueroa, Wong, Jaime & Gonzales, 2017). Each type of exercise requires a certain level of resistance, strength, speed and mobility depending on the importance of achieving the results that are intended to be obtained in competition. High-performance endurance sports include cardiovascular or aerobic exercise such as running, cross-country skiing, cycling, or swimming; of which are carried out over a long period of time (Joyner & Coyle, 2008). High-performance athletes must maintain a great effort for as long as possible, generating an increase in cardiac, pulmonary and circulatory capacity. These processes require increasing oxygenation and nutrients at the muscle level. During this process, athletes overcome their own resistance and increase the degree of intensity of exercise, generating systemic damage affecting the intestinal tract. Reflecting in damage to the epithelium that involves intestinal permeability, modifications in microbiota, oxidative stress and an increase in pro-inflammatory processes. The intake of natural products with a high content of dietary fiber and polyphenols over time has been a beneficial alternative to promote correct gastrointestinal and systemic homeostasis. Dietary fiber, in addition to reducing digestive symptoms such as constipation and diarrhea, improves the intestinal microbiome and serves as an energy substrate for the same intestinal microbiota, favoring the production of healthy metabolites that have a prebiotic effect, favoring intestinal symbiosis. Furthermore, metabolites such as polyphenols have been associated with a bidirectional relationship with the intestinal microbiota and are related to anti-inflammatory and antioxidant effects (Fraga, Croft, Kennedy & Tomás-Barberán, 2019; So et al., 2018). Increasing the consumption of these natural compounds during high resistance training can serve as an alternative to reduce the damage caused by exercise and promote the regeneration of intestinal tissue, as well as maintain systemic homeostasis.
Gastrointestinal symptoms caused by resistance training
An endurance athlete who practices high-performance exercise is a person who requires a high work level and development of their morphological, functional, biochemical and psychological capacities. This type of athletes is more prone to suffer gastrointestinal disorders and systemic modifications due the training that they perform is exhaustive (García-Naveira, 2010). The prevalence of diarrhea (44%), abdominal pain (44%), vomiting (22%) and nausea (89%) have been observed in high-performance athletes being these symptoms a cause of gastrointestinal discomforts (Figure 1) (Stuempfle, Hoffman & Hew, 2013). The modifications that occur at systemic level are changes of the gastrointestinal blood circulation caused by a splanchnic hypoperfusion resulting mainly damage to the intestinal tissue, erosions in the intestinal mucosa and an increase in intestinal permeability, triggering inflammatory and oxidative processes as well as intestinal microbiota modifications (Lamprecht & Frauwallner, 2012).
Modifications of the intestinal barrier generated by resistance training
The intestinal barrier it is represented by a monocellular layer of epithelial cells where the plasma membrane of these cells restricts transcellular transport of negative molecules. Within this monolayer, specialized cells, such as globet and Paneth cells provide additional components of the intestinal barrier by producing mucus and antibacterial proteins, respectively. Paracellular transport between epithelial cells is also restricted by intricate spatial arrangements of tight junction proteins including claudins, zonula occludens (ZO-1 and ZO-2) and occludens (Ghosh, Wang, Yannie & Ghosh, 2020). A defective intestinal barrier may result in increased intestinal permeability which promotes the exposition to luminal content and triggers intestinal imbalance. Inflammatory bowel diseases display several defects in the many components of the intestinal barrier. These alterations may represent a primary dysfunction in Crohn's disease and colon cancer. On the other hand, it has been described that the high-resistance training also impacts the intestinal barrier integrity (JanssenDuijghuijsen et al., 2016; Lee, 2015). Different in vivo studies have been carried out to establish how the type and exercise intensity training generate intestinal barrier modification. Mice that were subjected to exercise training at 80% VO2max until exhaustion, showed an increase in intestinal permeability after the training and the damage remained even after five hours post-exercise (Gutekunst, Krüger, August, Diener & Mooren, 2014). Human studies have also been carried out where it was observed that due to intensity and type of exercise that was considering of high-resistance, the athletes presented gastrointestinal symptoms emphasizing nausea, diarrhea or abdominal pain just after running a 10 km for 90 minutes. At the end of the race, the athletes had presented alterations in the gastrointestinal barrier with a specially increase of intestinal permeability (Karhu et al., 2017). In contrast with the modifications of the intestinal barrier caused by exercise, body temperature is another variable that modifies the intestinal barrier which can lead to presented intestinal permeability causing also systemic damage (Pires et al., 2017).
Microbiota modification generated by resistance training
The intestinal microbiota is the community of microorganisms that reside in the digestive tract. The main phyla included in the human microbiome are Firmicutes and Bacteroidetes represented the 90% of the total intestinal microbiota, however, there are other phyla in lesser quantity that perform various metabolic activities such as Actinobacteria, Verrucomicrobia and Proteobacteria (Greenhalgh, Meyer, Aagaard & Wilmes, 2016). The intestinal microbiota promotes digestion and absorption of nutrients from food to generate energy, as well as, protecting against infections, regulating the epithelium and balancing oxidative stress, promoting intestinal barrier function and protection against pathogen colonization and stimulation of the immunological system (Thomas, Parker, Divekar, Pin & Watson, 2018). Physical exercise is one of the causes of the modification of the intestinal microbiota, generating beneficial or negative effects on human health (Figure 2). Athlete microbiomes have been reported to contain a high abundance of Veillonellaceae, Bacteroidetes, Prevotella, Methanobrevibacter, andAkkermansia (Scheiman et al., 2019). The observational study by Clark & Mach, 2016 showed significant changes between the microbiota of the players and the control groups specifically in the relationships of Faecalibacterium prausnitzii and Akkermansia muciniphila. This bacterias have been suggested to be probiotics and both show a crucial role in prebiotic fermentation (Bu et al., 2020; Ferreira, Sousa & Andrade, 2017). In animal training models, a higher abundance of Firmicutes has been found compared to another bacterial phylum. This phylum is composed of more than 200 different genera such as Lactobacillus, Bacillus, Clostridium, Enterococcus and Ruminococus, strains related to positive effects on the intestinal microbiota (Rinninella et al., 2019). Additionally, members of the Bacteroidetes phylum in particular have been associated with human metabolic diseases and, a decrease in the B. phylum has been observed in the analysis of the microbiome of endurance athletes comparing this type of athletes with the sedentary control group (Kulecka et al., 2020). On the other hand, studies in professional cyclists show an increase in genera and species related to carbohydrate metabolism and energy generation emphasizing Prevotella and Methanobrevibacter smithii (Petersen et al., 2017). High intensity exercise, without adequate training, can be a stress factor for the body and this tendence has a negative effect on the intestinal microbiota (Ticinesi et al., 2019). In human trials, it was determined that an intense military training generated an increase in intestinal permeability and inflammation markers, these results were related to a greater abundance of Bacteroides (Karl et al., 2017). As part of the gut microbiota metabolism, the short chain fatty acids (SCFAs) are the main metabolites produced by the microbiota. SCFAs have been reported to increase after exercise. Butyrate is the most relevant SCFA as a marker of intestinal health and it is generated by Faecalibacterium prausnitzii, Roseburia hominis and Akkermansia muciniphila, and the genus Coprococcus. Specifically, the butyrate produced by Roseburia hominis and Faecalibacterium prausnitzii also has a positive impact on lipid metabolism in athletes (Machiels et al., 2014). With the mentioned above, it is clear that the microbiota and the metabolism products of them may be an intestinal barrier protection and at the same time dependent of the training intensity.
Impact of high-resistance training on oxidative stress
The human body naturally produces free radicals and reactive species as part of metabolic processes such as superoxide anion (O2•), hydrogen peroxide (H2O2) and hydroxyl radical (•OH), nitric oxide (NO) and reactive oxygen and nitrogen species (RONS) respectability (Radak et al., 2017). These molecules generate beneficial effects, among them, the activation of the immune system, allowing to counteract various pathologies. However, they can generate adverse effects like cytotoxicity, genotoxicity, development of neurodegenerative and cardiovascular diseases, inflammatory processes, cancer and systemic modification in situations of physical activity training and exercise by damaging several tissue and cellular components including oxidation of biomolecules (lipids, proteins and DNA) (Boccatonda, Tripaldi, Daví & Santilli, 2016; Sies, 2015). The organism has antioxidant defense mechanisms that neutralize the action of the different reactive species: superoxide dismutase (SOD), catalase (CAT), and glutathione peroxidase (GPx) as enzymatic mechanisms and non-enzymatic antioxidant mechanisms (ascorbic acid, α, β, γ isomers, tocopherols, tocotrienols, carotenes and flavonoids). Therefore, an imbalance between production of reactive oxygen species and an adequate antioxidant defense generates an oxidative stress state and possibly tissue damage (Figure 3). The exercise generates beneficial health effects by reducing oxidative stress. This effect depends on the type of exercise, the intensity and the duration with which athletes usually train (Pingitore et al., 2015). During high intensity exercise, an exacerbated state of oxidative stress is generated by different processes: a) oxygen metabolism, b) ischemia and reperfusion phenomena, c) autoxidation process of hemoglobin and myoglobin, d) increase in body temperature, e) catecholamines release) f) lactate production, (Duncker & Bache, 2008; Kruk, Kotarska & Aboul-Enein, 2020; McAnulty et al., 2005; Morris, Nevill, Boobis, Macdonald & Williams, 2005; Starkov, 2008). In response to these processes related with oxidative stress and exercise, it is activated the redox signaling pathway implicated in skeletal muscle contraction force, mitochondrial biogenesis and antioxidant enzyme induction (Mason, Morrison, McConell & Wadley, 2016). Different studies indicate that skeletal muscle cell mitochondria were the likely generators of high concentrations of hydrogen peroxide. However, NADPH oxidase and xanthine oxidase enzymes may contribute to the relative increase of oxidative stress in whole body (Jackson, Vasilaki & McArdle, 2016). Studies indicate the use of the pharmacological agent Allopurinol inhibits xanthine oxidase, a free radical-generating enzyme, this compound is involved in tissue damage produced during exhaustive exercise (Sanchis et al., 2015). In this sense, a greater amount ofRONS regulates negatively muscle metabolism by inactivating the adenosine monophosphate-activated kinase (AMPK) pathway and mitochondrial biogenesis (Morales-Alamo & Calbet, 2016). There are reports that indicate the continuous production of free radicals generated during high-resistance training promote a physiological adaptation to oxidative stress through the action of endogenous antioxidants after a certain periodic time, this is due to the increase in antioxidant enzymes such as glutathione reductase and superoxide dismutase. All these mechanisms depend of the intensity and exercise type promoting benefits or harm at systemic level (Boccatonda et al., 2016).
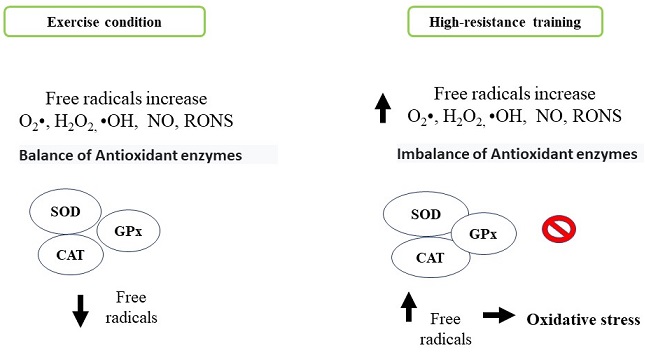
Figure 3 Comparison of cell antioxidant system activation. Antioxidant process generated by antioxidant enzymes under normal exercise execution conditions compared to oxidative stress generated by overporduction reactive oxygen species generated during exercise of can resistance. Personal elaboration.
Inflammatory process generated by high-intensity training in athletes
The inflammatory process is one of the basic defense mechanisms against external aggressions. Activation of the immune response appears to play a key role in endurance exercise performance. This is related according to the type and intensity of training, generating mild or severe inflammatory processes. Moderate exercise allows an adaptation and improvement of the immune system compared to resistance exercise that can increase the concentration of reactive oxygen species and, consequently, induction of an inflammatory reaction (Pedersen, 2017). The inflammatory mechanism of resistance exercise has been described, this includes a reduction in the function of immune cells (Natural killer cells, neutrophils, T and B lymphocytes), increase of proinflammatory (TNF-α, IL-1β e IL-6) and antiinflammatory cytokines (IL-10), chemokines (IL-8) and the expression of macrophages of the major histocompatibility complex (Passos et al., 2019). It has been described that the secretion of interleukins is related to the course of time after the execution of the exercise. IL-6 is synthesized immediately after the exercise. This is secreted at muscular level and it is concentrations usually have a fifty-fold increase compared with normal concentrations (Pedersen & Hoffman-Goetz, 2000). IL-1 has been detected five days after training, it was related with prolonged muscle damage. Whereas IL-10 is secreted after thirty minutes of high-intensity training (Cabral-Santos et al., 2019). Increased secretion of IL-10 has been correlated to the protection of other systemic inflammatory processes including intestinal inflammation (Gleeson et al., 2011). High-intensity performance exercise can generate an alternate inflammation process by increasing the permeability of the intestinal epithelial wall and modifications in the mucosal layer (Marlicz & Loniewski, 2015). Therefore, it implies loss of the selectivity of the intestinal wall, allowing the entry of large molecules into the bloodstream, generating an immune response. Pathogens or their endotoxins can be transported through the damaged intestinal wall, generating the recognition and activation of specific toll-like receptors (TLR), these compounds activate signaling cascades, generating the transcription process of proinflammatory proteins to the gastrointestinal tract. In this sense, the real inflammatory damage is going to be as a consequence of exercise type and the complications at systemic level, as it happens at intestinal level (Trevisi et al., 2008).
Effect of natural sources intake as a therapeutic alternative on high- resistance athletes
High-resistance athletes have specific nutritional needs to maintain adequate energy and nutrient consumption and then be able to performance periods of long-lasting resistance training, avoiding fatigue and health conditions. For this reason, high-resistance athletes and any kind of athletes should consume different types of products gels, bars, drinks, protein, vitamins, minerals, ergogenic and herbal supplements (Table I). Currently, the athletes consume natural products with the aim of improving performance and frequently there are found it on the market, such as extracts obtained from different parts of plants and fruits. This type of extracts contains a large number of bioactive compounds (phenolic compounds, alkaloids, carotenoids, and others) which are used as supplements to increase mental agility, stimulate energy metabolism and improve muscle performance. Likewise, athletes usually consume different products that contain dietary fiber most of the time to maintain weight control and intestinal homeostasis.
Table I Type of natural products consumed by athletes.
Product | Systemic effect |
---|---|
Carbohydrates | |
Energy drinks, energy bars, gells, and concentrate powders. | Improvement of exercise performance and increased of the ergogenic effect in central nervous system (Redondo et al., 2019). |
Proteins | |
Eggs, milk, meat and products like casein or whey. | Activation of metabolic pathways related with muscle hypertrophy and prevention of protein catabolism. Also, increased muscle strength (Redondo et al., 2019). |
Amino acids | |
Arginine or branched-chain amino acid mixtures. | Blood flow increase, oxygen and nutrients supply to skeletal muscle (Brooks et al., 2016). |
Polyunsaturated fatty acids | |
Capsules and food. | Improvement of protein synthesis and muscle function, less reduction of damage induced by inflammation process during exercise (Lewis, Radonic, Wolever & Wells, 2015). |
Natural sources of nitrates | |
Beet juice as nitrate sources in presentations in forms of powder, juice concentrates and shots. | Reduction of oxygen consumption and improvement of exhaustion time, cardiorespiratory performance and maximum VO2 (Vitale & Getzin, 2019). |
Antioxidants | |
Vitamins such as C and E. | Inhibition of signaling pathways in oxidative stress process (Gomez-Cabrera et al., 2008; Passos et al., 2019). |
Dietary fiber intake as a natural alternative
There are a large number of natural products that can be studied, such as fruits, vegetables or grains, that have been reported to be high contain in fiber. Dietary fiber is a carbohydrate polymer with three or more monomeric units that are resistant to endogenous digestive enzymes, therefore are not hydrolyzed or absorbed in the small intestine. This is classified into insoluble and soluble fiber. The fiber is available in the form of insoluble or partially fermentable fiber, which generally has cellulose as an essential component, followed by lignin and hemicelluloses. This type of fiber is poorly degraded by the action of intestinal and colonic bacteria, being practically entirely excreted through the feces, exerting an effect on the volume and fecal weight. The soluble fiber is responsible for delaying gastric emptying and intestinal transit, its solubility in water conditions the formation of gels in the intestine, which makes this type of fiber more fermentable and contributes to the production of different beneficial metabolites and includes gums, mucilages, inulin, fructooligosaccharides, galactoolysaccharides, pectins, and some hemicelluloses (Barber, Kabisch, Pfeiffer & Weickert, 2020).
The adequate fiber intake is an option to improve gastrointestinal symptoms regardless of condition. The recommendations for fiber intake in healthy people based on their sex and age, as mentioned by the Institute of Medicine, indicated a recommended dose of fiber between 19-38 g/day (Institute of Medicine, Food and Nutrition Board, 2005). Currently, research on the benefit of fiber consumption in high-performance athletes is limited. However, it has been established that the consumption of fermentable fiber generates a prebiotic effect by increasing different bacterial genera in the colon, mainly Bifidobacterium (Cantu & Hamaker, 2020). Sometimes, gastrointestinal diseases in endurance athletes are often related to inadequate fiber intake experiencing abdominal cramps, diarrhea or symptoms such as bloating during the training process (Jeukendrup, 2017). Currently has been established a relation between fiber consumption and increased physical performance in older population generating an improvement in physical performance by measuring gait speed and grip strength (Wu et al., 2013). At the fiber fermentation process, short chain fatty acids are generated, these generate beneficial effects in the colon. In vitro model studies have shown that butyrate exerts specific anti-inflammatory actions, modulating the transcription factor NF-kB and therefore decreasing pro-inflammatory cytokines (TNF-α) (Parada-Venegas et al., 2019). Likewise, it has been reported that the production of propionate, one of the most important short-chain fatty acids produced as a substrate by bacterial fermentation during the consumption of dietary fiber, increases training performance by improving muscular endurance (Scheiman et al., 2019). In this sense, it has been shown that the controlled consumption of dietary fiber in the diet has anti-inflammatory effects, decreasing the serum level of inflammatory mediators and a decrease in the oxidative process in the body (Tierrablanca, Luna, Guzmán, Ramírez & Aguilar, 2019). Therefore, the consumption of fiber by high resistance training athletes with intestinal injury can help to regenerate the intestinal barrier, promoting a symbiotic effect on the gut microbiota, reducing oxidative stress and inflammatory processes, and therefore, reducing gastrointestinal symptoms.
Polyphenols, an alternative for high-resistance training
Polyphenols are a large group of bioactive compounds derived from plants. These are classified into two different main classes: phenolic acid and flavonoids. Its chemical structure is conformed of a C6-C3-C6 base skeleton. At flavonoids, the two aromatic rings that conformed it, differ in their oxidation degree and at the same time in different subclasses: flavones, flavonols, isoflavones, flavanones, anthocyanins and flavanols. Polyphenols are also found within food sources as large structures, composed of oligomers and polymers forming tannins, which are divided into hydrolysable tannins (gallic and ellagic acids) and condensed tannins (procyanidins) (Amiot, Riva & Vinet, 2016). These compounds are metabolized in the intestine through digestive enzymes, however not all polyphenols are metabolized at this level since large compounds such as hydrolysable tannins, condensed tannins, and the polyphenols that are bounded to dietary fiber will be go to large intestine where they will be finish being metabolized by the gut microbiota (Crozier, Del Río & Clifford, 2010). In athletes the benefits are related to the reduction of severe oxidative damage, muscle weakness, reduction of inflammatory processes and intestinal health (Espín, González-Sarrías & Tomás-Barberán, 2017). For this reason, athletes currently consume sports nutritional supplements that contain antioxidants specifically polyphenolic compounds such as flavanols, (epi)-catechin, catechin and procyanidins, being consumed naturally as part of supplements. Contrary, some of the supplements that athletes consume usually have some source of polyphenols in their composition, but it is usually in small doses (Daneshvar et al., 2013; Gholami & Ardestani, 2018). The polyphenols consumption becomes relevant to increase high-resistance training over time and the impact that these compounds can have on the intestinal epithelium regeneration reducing the symptoms that afflict high-resistance athletes has been observed (Bowtell & Kelly, 2019). Polyphenols such as hydroxycinnamic acids, flavanols, flavanols and hydroxybenzoic acids, have a beneficial effect due to their prebiotic, antioxidant and anti-inflammatory properties that are related to improving systemic and intestinal discomfort at the gastrointestinal tract level in athletes (Singh, Yau, Leung, El-Nezami & Lee, 2020). It should be described that athletes usually consume products that contain polyphenols in order to reduce a muscular injury generated by high impact or resistance exercise since during this process, the muscle fibers are exposed to high mechanical forces causing muscle damage. Currently studies have determinate that supplementation of a 1200 mg/day dose of polyphenols during 3 days prior to exercise and recovery has been reported to improve muscle function (Bowtell & Kelly, 2019). On the other hand, the consumption of a commercial supplement based on polyphenols extract managed to demonstrate its effect on metabolic homeostasis and exercise performance during high-intensity training under anaerobic conditions (Cases et al., 2017). Another type of effects generated by polyphenols consumption are the effects related to the intestinal barrier regeneration, as well as, systemic homeostasis what leads to a decrease of gastrointestinal symptoms in high-resistance training (Zhu, 2018). Likewise, it has been established that the polyphenols consumption modifies the intestinal microbiota promoting a prebiotic effect (Most, Penders, Lucchesi, Goossens & Blaak, 2017). Flavonoids can modify the composition of gut intestinal exerting prebiotic and bactericide properties (Etxeberria et al., 2015). In vitro and in vivo studies have shown that polyphenolic compounds promote the growth of Lactobacillus, Bifidobacterium, Akkermansia muciniphila, Faecalibacterium prausnitzii, and Roseburia (Anhê et al., 2015). Like it is mentioned previously, the growth of these strains at intestinal level can promote beneficial effects for the athlete. Therefore, the intake of natural sources by high resistance training athletes with intestinal injury can help to regenerate the intestinal barrier, promoting a symbiotic effect on the gut microbiota, reducing oxidative stress and inflammatory processes due to can promote a real decrease in gastrointestinal discomfort.
Conclusions
The consumption of natural sources rich in fiber and polyphenolic compounds are excellent candidates for the treatment of symptoms presented by high-resistance athletes. These compounds can prevent or treat conditions generated at the gastrointestinal level. This effect is generated by reducing the processes of disruption of the intestinal barrier, generating a homeostasis of the intestinal microbiota, reducing oxidative and inflammatory processes. Making them excellent sources for constant consumption in athletes without affecting the physical performance demanded by the athlete. However, it is necessary to study in depth the mechanisms of the ingestion of natural sources in in vivo models of high resistance exercise as well as to establish adequate doses and types of polyphenols that exert a beneficial effect especially in this particular population to reach precise conclusions.