Introduction
Due to the simplicity of construction and versatility of the steel fiber reinforced concrete (SFRC), its use has increased significantly in recent years. SFRC is currently used in many fields of the civil engineering such as airport pavements, industrial floors, hydraulic structures, beams and walls for buildings, supporting underground tunnels, coatings with shotcrete or sprayed concrete (Abbas et al., 2014; Mahmud et al., 2013; Caballero et al., 2013; Johnston, 1982; Ramzi y Omer, 1999).
The corrosion of the rebar by the action of the chloride ion is one of the main causes of the premature deterioration in concrete structures by oxidation of the rebar (Granju y Balounch, 2005). This deterioration is due to the urban and industrial development in coastal zones across the whole world, where the sea salt is one of the principal adverse factors. The chloride ions are found in a small quantity in the environment, and therefore, they may be present in products used in the concrete mix such a cement, coarse and fine aggregates and mixing water. The quantities of these products are low in comparison with the mass or the volume of other materials in the mix. By making use of such products, small amounts of chlorides ion are adding to the mix, especially when additives are used (Pereira de Oliveira et al., 2013). The threshold or concentration of chlorides necessary to break the passive layer of the steel is related to certain parameters such as contents of tricalcium aluminate (C3A), tetracalcium of aluminoferrite (C4AF), pH and W/C ratio (Ramzi & Omer, 1999). Due to the chloride ions, the medium resistivity decreased when the ionic concentration of the matrix pores increase, which increases the electric conductivity as well as the corrosion velocity.
The aim of this work is to assess the electrochemical behavior of the concrete reinforced with Dramix RC-65-35-BN low carbon steel fibers at a short term (phase of corrosion initiation). The mixes of SFRC were exposed to penetration of aggressive agents (water, 3.5% NaCl solution), and then the durability of the steel fibers because such exposure was evaluated.
Experimental program
Type I Portland cement was used as cementitious material, including fly ash replacement of 10%. The aggregates used for the concrete mix correspond to a fine gravel of grays tones having maximum size of 10 mm, specific gravity of 2.64, and absorption of 3.07%. Two kinds of sand were used; one from river and one from crag with specific surface area of 2470 kg/m3 (3.02 and 1.49 fineness modules, respectively) and absorption of 0.77% and 0.79% respectively. A water/cement ratio of 0.58 and two different dosages of steel fibers were used for the concrete mixes. In addition, two plasticizer additives were used: Plastiment AD 20 (SIKA, 2013), and Sika Viscocrete 2100 (SIKA, 2012). The composition of the mix is summarized in Table 1.
The experimental tests were performed on specimens with steel fiber reinforced concrete (SFRC). Cylinders and beams having standard dimensions of 150x300 mm and 150x150x600 mm, respectively, were cast. The specimens were cured by applying a curing membrane on their surfaces. The curing membrane was based on aqueous emulsion of paraffin, which guarantees the development of the concrete strength.
Hooked-end steel fibers having 0.55 mm of diameter and 35 mm of length, aspect ratio of 65 and low carbon content, were used (Bekaert, 2010). Fiber dosages (D f) of 30 kg/m3 and 60 kg/m3, corresponding to fiber volume fraction (Vf) of 0.38% and 0.76%, respectively, were used in this study. The steel of the fibers was corrugated and complied with the parameters of standard test method NTC 5214-10. The characteristics of the fibers are shown in Table 2. In the table, effective work area represents the area of all fibers embedded into each specimen considered for the electrochemical measurements.
Specimens with steel fibers were subjected to corrosion environments for monitoring the corrosion during 56 days, that is, they were immersed in potable water (A1) and in a solution having 3.5% of analytical sodium chloride Panreac PA131655.1211 NaCl 99.0% (A2). Description and quantity of electrochemical tests are shown in Table 3. Tests were aimed at measuring the corrosion potential, current density and polarization resistance of SFRC specimens. After 28 days of curing of specimens, electrochemical measurements were recorded every 14 days, during 56 days: only at each measurement date, the SFRC specimens were removed from their environments and tested under immersion in a solution of distilled water. This study was aimed at starting the corrosion process through the chloride ion (phase of corrosion initiation), and analyzing the degradation of steel fiber reinforced concrete in terms of the time. For such purpose, SFRC was considered as a conductive material due to saturation of the pore network of the material, which leads conductivity between fibers. Furthermore, X Ray Diffraction (XRD) test was carried out on the steel fibers to determine corrosion products associated to the composition of fibers.
The electrochemical characterization was performed using a Gamry PCI-4 potentiostat/galvanostat, which uses the rest potential technique. Current density and linear polarization resistance (LPR) tests were performed using a cell composed by a counter-electrode of stainless steel, a reference electrode of Cu/CuSO4 and the Dramix RC-65-35-BN steel fibers embedded in the concrete as a working electrode (assuming steel fibers embedded in the matrix were not interconnected). The linear polarization resistance tests were performed applying a low external potential (±20mV) around the open circuit potential, where a resistance drop correction was first applied verifying that drop values were lower than 75 Ω. The measurements of the open circuit potential were taken in a time of 12000 seconds until stabilization of itself was reached. Figure 1 shows the setup of both current density and linear polarization resistance tests. For the corrosion potential tests, the setup was adapted from the study of Mangat and Gurusamy (1987) (Figure 2). Mangat and Gurusamy (1987) measured the half-cell potential of fibers exposed at the fractured face after flexure tests and applying 2000 cycles of wetting and drying. During such test, the electrical reference and half-cell were fixed on the concrete surface (negative terminal), and the electrical connection to the steel fiber was then moved to the selected fibers (positive terminal) and finally half-cell potential of each fiber was recorded (Tran et al., 2015). As a working electrode, in this study, the SFRC specimens were first saw in the mid-high and then, the connection to fibers in the fractured face was made. Hence, mapping of measurements on selected fibers was conducted and the results were computed as the average from these embedded fibers in three SRFC specimens (for both cylinders and beams) that were considered at each measurement date. This average was considered due to the similarity between the measured values.
It was also identified the corrosion products generated on the steel fibers surfaces by X-ray diffraction (XRD). The experimental setup of the X-ray diffraction test comprises a Goniometer having reference PW3050/60 (θ/θ), which is managed under a XPERT-PRO system using a monochromatic radiation of Cu Kα 1.54 Å. The Goniometer is operated at 40 kV and 40 mA under temperature conditions of 25°C. Scanning on the surface was performed from 2θ = 20.01° to 2θ = 99.99° with a step of 2θ = 0.02° at a scanning time of 1 second. The crystalline phases of the steel fibers surface was identified by an X-ray diffractometer having reference XPERT-PRO, and using the diffraction database of the equipment. In addition, it was used the MAUD software which is an analysis program based on Rietveld method. Such method consists in adjusting a theoretical diagram that coincide entirely with the observed diagram. The theoretical diagram is based on the crystalline structures and on series of crystalline parameters. The steel fibers were pull out of the concrete matrix and analyzed after 56 days of exposure to chloride ion (A2) and potable water (A1). For evaluating the reliability of the results obtained in the tests, statistical parameters such as arithmetic mean (X) and the coefficient of variation (CV) were utilized. Such parameters determines the average and the dispersion of the electrochemical measurements, respectively.
Results and discussion
Corrosion potential
The results of corrosion potential of SFRC specimens are shown in Table 4 and the mean values are plotted in Figure 3. Two trends regarding the low carbon steel fibers in the concrete are shown in Figure 3. One trend is related to the corrosion potential variation in function of the fiber dosage (30 and 60 kg/m3), and other related to effect of chloride ion in the mixture at early ages. The obtained corrosion potentials of the concretes are shown in Figures 3a and 3b using graphics of corrosion potential versus evaluated time. This evaluation consisted in periodic measurements of corrosion potential (56 days). The criterion used for this evaluation was that specified by the ASTM C 876-09 standard test method. Corrosion monitoring was performed having the specimens immersed in water and simultaneously specimens exposed to chloride ion by immersion in a synthetic solution that simulated the seawater (3.5% NaCl).
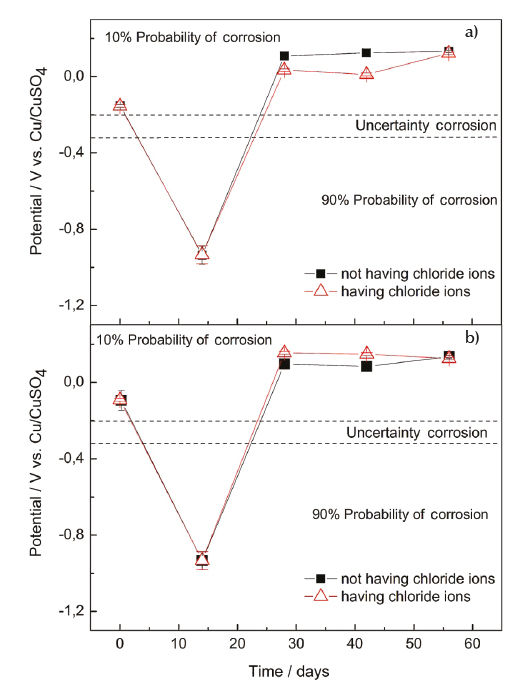
Figure 3 Corrosion potential for concretes with and without exposition to chloride ion, a) dosage of 30 kg/m3, b) dosage of 60 kg/m3
Using the concept specified by ASTM C 876-91, it can be observed that for the first level evaluated, corresponding to 28 days of curing (0 days in graph), and both fiber dosages of 30 kg/m3 and 60 kg/m3, has a potential that coincides with the zone of passivation. Measurements during the first 14 days showed that the specimens exposed to water (A1) and to chloride ion (A2) suffered a decrease of potential and passed to the appropriate area of possible corrosion. This is because at the end of this measurement, the potential are found in a region having 90% of probability of corrosion (also known as active corrosion region). For the third level of exposition (28 days), the specimens subjected to both environments showed an increment of the corrosion potential. Therefore, the values of corrosion potential moves towards a cathodic zone, which indicates that, the material search a better performance of the corrosion in these environments. Subsequently, for the fourth and fifth level of exposition (42 days and 56 days, respectively), the potential keeps steady for both fiber dosages and for the two evaluated environments (A1, A2), that is, the potential remains in a passive region. In addition, the effect of the chloride ion observed in the corrosion potential begins to oscillate in a range of ± 10 mV from the third level of exposition. This behavior is not an indication of corrosion that could be generated by the depassivation.
Polarization resistance and current density
The results of polarization resistance of SFRC specimens are shown in Table 5 and the mean values are plotted in Figure 4a. Figure 4a shows the values of polarization resistance measured using the LPR technique. It is observed that the measured values are related to the concrete having fiber dosages of 30 kg/m3 and 60 kg/m3, and subjected to a solution having and not having chloride ion at all levels of evaluation. Overall, this polarization resistance value decreased gradually in each evaluated level. For both dosages, the SFRC specimens in presence of potable water (A1) exhibited a better behavior and experienced higher resistance values from the beginning of the evaluation, because this parameter was kept high until the last evaluated levels. Hence, it indicates that SFRC exhibited a higher value of resistance when it is not in contact with chloride ion. In contrasts, for both dosages, this parameter decrease in function of time for the concrete subject to chloride ion, that is, in the environment A2. Nonetheless as shown in Figure 4a, the most efficient concrete mix was that having less quantity of fibers in the mix (Bartos, 1981). Such trend is associated to the lower exposure area of the steel fibers, which leads high values of polarization resistance in SFRC specimens.
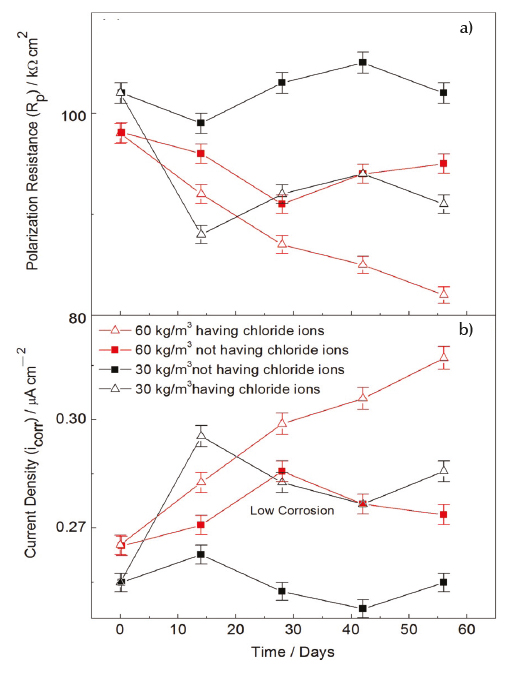
Figure 4 Polarization resistance and current density of the steel fibers embedded in Portland concretes with and without exposition to chloride ion
The results of current density of SFRC specimens are shown in Table 6 and the mean values are plotted in Figure 4b. The current corrosion is an inverse function of polarization resistance in accordance with the established protection criterion for plain concrete. However, the specimens subjected to chloride ion showed low corrosion current values (Figure 3). Following the adopted criterion, SFRC specimens with exposition to chloride ion are in a level of low values of corrosion. SFRC specimens having Df = 30 kg/m3 and Df = 60 kg/m3, and subjected to a chloride ion showed a high corrosion density that increases from the beginning of exposure. For the time evaluated in this study, this increment keeps constant in the cathodic zone, which generates a low corrosion condition (Aperador et al., 2012).
XRD analysis
The spectrum corresponding to steel fibers having and not having chloride ion is included in Figure 5. The figure shows the different phases that were obtained in the XRD spectrum. These phases are related to RC-65-35-BN steel fibers exposed to the chloride ion, and are labeles as C: calcite, G: goethite, AN: andradite, H: halite, MG: magnetite, W: wüstite, L: lepidocrocite, HM: hematite and Q: quartz. Since one type of fiber was only used for SFRC, both specimens having Df = 30 kg/m3 and Df = 60kg/m3, showed a similar behavior. The Q phase shows high intensity. Such intensity is related to the concrete matrix (cementitious materials and aggregates). The oxides found on the steel surface are MG and W. It is observed that, excluding the quartz, MG and W phases show the greater intensity when comparing to the others phases and hydroxides belong to the G, L, and HM phases. Both the oxides and hydroxides registered in the spectrum are presented in the majority of the corrosion processes of low carbon steel fibers (Choi et al., 2014). Overall, as shown in Figure 5, the spectrum shows the same phases for both fibers having and not having chloride ion. However, the spectrum of fibers having chloride ions has lower intensity.
When comparing the steel fibers having and not having exposition to chloride ion, it is observed that steel fibers not subjected to chloride ion have the highest peak corresponding to the quartz. Such trend is related to the adhesion of the metal surface with the concrete. In contrast, the steel fibers subject to chloride ion show the lowest peak due to the loss of adherence between matrix and the fibers. For both dosages, the generated corrosion products are linked basically to the degradation process of the steel.
Conclusions
The behavior of SFRC subjected to an environment having and not having exposure of chloride ion at a short term (phase of corrosion initiation) was assessed in this paper. The results measured in this study revealed the following conclusions:
When concrete specimens reinforced with steel fibers using dosages of 30 and 60 kg/m3 are subjected to an environment having and not having content of chloride ion, they experienced a passive behavior. This process is maintained until the end of the time evaluated (56 days). The analysis performed with the polarization resistance technique indicated that exposure of SRFC specimens to the chloride ion generates a decrease of the corrosion resistance as the dosage of fibers increase in the concrete mix. This trend is associated to greater exposure area when having higher fiber dosage. It is worth mentioning that despite the corrosion resistance values decrease, corrosion potential values for dosages of 30 and 60 kg/m3 were similar because these concrete mixes were exposed at the same exposure medium. Therefore, the most efficient concrete mix was that having less quantity of fibers in the mix, because at higher fiber content, the SFRC specimens will be more prone to corrosion.
The results obtained with the XRD technique showed that the proportion of goethite hydroxide is higher than the other products such as magnetite and wüstite. Such trend indicates that magnetite and goethite are products with higher intensity compared to the other phases found. However, it was also found lepidocrocite as other corrosion product. Comparing these results with the electrochemical measurements, it can be state that for the phase of corrosion initiation, the analyzed specimens generate the same content of corrosion products.
For both dosages of fibers analyzed, SFRC specimens subjected to chloride ion showed high corrosion density values. Nevertheless, these specimens remain in a passive region where a low probability of corrosion exists. Therefore, for the phase of corrosion initiation evaluated in this study, the SFRC subject to chloride ion showed a behavior, which does not affect the durability of the concrete specimens.