Servicios Personalizados
Revista
Articulo
Indicadores
-
Citado por SciELO
-
Accesos
Links relacionados
-
Similares en SciELO
Compartir
Agrociencia
versión On-line ISSN 2521-9766versión impresa ISSN 1405-3195
Agrociencia vol.51 no.5 Texcoco jul./ago. 2017
Water-Soils-Climate
Effects of the aerator roller and fire on the physical and hydrological properties of the soil in the Scrublands of Coahuila, México
1Facultad de Ciencias Forestales, Universidad Autónoma de Nuevo León. Carretera Nacional 145. 67700. Linares, Nuevo LeónMéxico,. (medina.romelia.10@gmail.com).
2Agriculture Faculty, Kyushu University. Hakozaki, Higashi-ku, Fukuoka, 812-8581. Japan. (kubot@agr.kyushu-u.ac.jp).
3Facultad de Economía, Universidad Autónoma de Nuevo León. Avenida Lázaro Cárdenas 4600 Oriente. 64930. Fraccionamiento Residencial Las Torres, Monterrey, Nuevo León. (marcovgmeza@hotmail.com).
Since the XVI century, the Chihuahuan desert has been a grazing area. This area has ecological and economical significance for the country; however, overgrazing has caused changes in the physical and hydrological properties of the soil. These changes were evaluated on microphyllus desert scrublands (MDM) and rosette desert scrublands (MDR) in Coahuila, Mexico. The rehabilitation techniques were an aerator roller during 2004, 2008 and 2011, and natural fires. The hypothesis was that both techniques promote the increase of the soil’s organic matter (MOS), water infiltration, and decrease soil compaction. A bifactorial experimental design was used which consisted of 10 treatments: 1) control (MDMt and MDRt); 2) aerator roller, applied in 2004 (MDMRA04 and MDRRA04); 3) 2008 (MDMRA08 and MDRRA08); 4) 2011 (MDMRA11 and MDRRA11); and, 5) burned area, 2011 (MDMIN11 and MDRIN11). The following variables were analyzed: useful water (AU), coefficient of permeability, electrical conductivity, apparent density, water infiltration on the soil, soil organic matter, soil reaction, mechanical resistance to penetration, and soil texture. Five treatments were applied on both types of vegetation, and the analysis of variance showed significant differences in all the variables, except for the AU percentage. The RA11 and IN11 treatments’ MOS content doubled on both types of vegetation, with regard to the content observed on the control treatments. Permeability decreased by 5, 82, 45, 48, and 12 %, on the MDMRA04, MDMRA08, MDMRA11, MDRRA08, and MDRIN11 treatments, respectively, and increased by 6 and 2 % on the MDMIN11 and MDRRA04 treatments, in relation to control. During the first 3 years, the aerator roller increased MOS and decreased soil compaction. Therefore, using the aerator roller is a periodic management option depending on the soil variables’ state, in relation with its use.
Key words: soil organic matter; microphyllus and rosette desert scrublands; soil compaction; permeability; fire
El desierto Chihuahuense es un área pastoreada desde el siglo XVI, con importancia ecológica y económica en el país, donde el sobrepastoreo ha provocado cambios en las propiedades físicas e hidrológicas del suelo. Estos cambios se evaluaron en ecosistemas de matorral desértico micrófilo (MDM) y rosetófilo (MDR) en Coahuila, México. Las técnicas fueron de rehabilitación con rodillo aireador en 2004, 2008 y 2011 y de un incendio natural. La hipótesis fue que ambas promueven el incremento de la materia orgánica del suelo (MOS), la infiltración de agua y disminuyen la compactación de suelo. El diseño experimental fue bifactorial con 10 tratamientos: 1) testigos (MDMt y MDRt), 2) rodillo aireador aplicado en 2004 (MDMRA04 y MDRRA04), 3) 2008 (MDMRA08 y MDRRA08), 4) 2011 (MDMRA11 y MDRRA11) y, 5) área incendiada 2011 (MDMIN11 y MDRIN11). Las variables analizadas fueron: agua útil (AU), coeficiente de permeabilidad, conductividad eléctrica, densidad aparente, infiltración de agua en el suelo, materia orgánica del suelo, reacción de suelo, resistencia mecánica a la penetración y textura de suelo. El análisis de varianza mostró diferencias significativas en todas las variables, excepto el porcentaje de AU, entre los cinco tratamientos en ambos tipos de vegetación. El contenido de MOS de los tratamientos RA11 e IN11 se duplicó en ambos tipos de vegetación respecto al observado en los tratamientos testigo. La permeabilidad disminuyó en los tratamientos MDMRA04, MDMRA08, MDMRA11, MDRRA08 y MDRIN11 (5, 82, 45, 48 y 12 %, respectivamente) y aumentó en el tratamiento MDMIN11 y MDRRA04, respecto al testigo en 6 y 2 %. El rodillo aireador durante los primeros tres años aumentó la MOS y redujo la compactación del suelo, por lo cual el uso de rodillo es una opción de manejo periódico en función del estado de las variables del suelo relacionadas con su uso.
Palabras clave: materia orgánica del suelo; matorral micrófilo y rosetófilo; compactación de suelo; permeabilidad; fuego
Introduction
The soil properties are susceptible to changes in land use and are indicators of the soil quality (Wang et al., 2012). The soil physical qualities such as density, pore distribution and size, water retention capacity, water content in soil, infiltration, and aggregation capacity, are easily altered (Spedding et al., 2004; Bhattacharyya et al., 2008; Cjpek et al., 2012). In addition, the organic matter is highly susceptible to agricultural overexploitation and fire (Darwish et al., 1995; Certini, 2005; Swędryńska et al., 2013).
Changes in land use can dramatically alter the vegetation, even for brief periods (Huang et al., 2006), because the vegetation enhances the soil’s physical, chemical, and microbial properties, through the decrease of apparent density, and the increase of nutrients, infiltration rates, water retention, and plant biomass (Jia et al., 2011). As a result of erosion, the reduction of the soil capacity to store available water is the factor that most contributes to soil productivity loss (Rasouzadeh and Yaghoubi, 2014). The edaphic factors, such as pH, nutrient levels, quality and quantity of the organic matter (MOS), change with depth (Rumpel and Kögel-Knabner, 2011; Eilers et al., 2012). Soil MOS is one of the main factors that affect other soil properties (Murray et al., 2014) and its functions, including water retention (Carter, 2002), air and water infiltration (Hillel, 2004; Li et al., 2007), and stability of aggregates (Six et al., 2004); it also modifies the porosity and available water capacity which can enhance root development, stimulates plant growth and crop yield, directly or indirectly replacing nutrients (Darwish et al., 1995), and reduces erosion (Li et al., 2007).
The disturbed ecosystems tend to decompose (Montaño-Arias et al., 2006); this affects the ecosystem operation, due to the alteration of its nutrients circulation dynamic, primary productivity, and water flow and retention (Maass, 1998). Fire causes significant physical and chemical changes -such as loss of vegetation cover, soil hydrophobicity development, and permeability loss (Cydzik and Hougue, 2009). These effects on the soil properties depend on its intensity and duration (Certini, 2005). Fire has the highest impact on MOS, from a light volatilization of minor components, up to carbonization or complete oxidation (Certini, 2005).
For decades, researchers have carried out experiments and developed management systems of shrub species in order to return pastures to their climax condition and to improve the productivity of the rangelands, (Anaya and Barral, 1995; Molinar-Holguín et al., 1998; Casanova et al., 2007). Sierra Maderas del Carmen, located in northern Coahuila, has a long history of cattle grazing (INE-SEMARNAT, 1997), which has favored soil compaction and changes in the vegetation structure of the microphyllus and rosette desert scrublands. In this zone, enhancement projects were implemented through mechanical treatments; however, these projects focused on the evaluation of the benefits for vegetation (Casas and Manzano, 2009; Ayala et al., 2014; Medina et al., 2016), and not for other ecosystem components. The main objective of our study was to evaluate the changes on some hydrological and edaphic properties in areas where wildfires took place, as well as the areas were mechanical treatments were carried out -using an aerator roller-, in order to find out the effects that each treatment has on the soil. The hypothesis was that the effect of fire and of the aerator roller will increase MOS and permeability, as well as decrease apparent density.
Materials and Methods
The study was carried out in the desert lands of Sierra Maderas del Carmen, in northern Coahuila, within Los Pilares -a private conservation area- (McKinney et al., 2006) (Figure 1), between 29° 22.45’ and 28° 42.21’ N, 102° 56.23’ and 102° 21.08’ W, at an altitude of 1182 m. The annual average precipitation is 237.4 mm and the annual average temperature is 21.5 °C. The predominant soils are calcium kastanozems, rendzinas, chromic vertisols, and calcareous litosols and reghosols (SPP 1982a; 1982b; 1983). The vegetation types include: oak (Quercus), pine (Pinus), and oyamel (Abies) woodland; submontane scrublands, zacatales, and the Chihuahuan desert scrublands, which include the microphyllus and rosette desert scrublands, gypsophila and halophilous communities (INE-SEMARNAT, 1997). The experimental plots were located in microphyllus and rosette desert scrublands.
In the spring of 2014, five treatments were defined within the microphyllus desert scrublands and five within the rosette desert scrublands. They all had the same soil type (calcareous reghosol), and a slope lower than 5°. A bifactorial experimental design was used which consisted of 10 treatments: 1) control (MDMt); 2) aerator roller, applied in 2004 (RA04); 3) roller, applied in 2008 (RA08); 4) roller, applied in 2011 (RA11); and, 5) burned area, 2011 (IN11). Samples were taken from a 1024 m2 (32 m×32 m) georeferenced plot which was establish for each treatment (Estrada-Castillón et al., 2014; Medina et al., 2016). Four 1.5 kgs samples were obtained from each plot, at a 0-30 cm depth; they were subsequently analyzed in the lab, to find out their physicochemical properties (DOF, 2002). We also collected four undisturbed samples, in order to determine the soil’s coefficient of permeability (K), using the JIS method (Das, 1997). In addition, we took soil samples to determine its apparent density (DA), using the cylinder method (Pennock et al., 2008). Samples were taken at two different depths -0 to 10 cm and 10 to 20 cm- to carry out a permeability and DA analysis.
The following analysis were carried out in the laboratory: organic matter (MOS) content, using the AS-07-NOM-021-REC-NAT-2000 method, Walkley/Black (DOF, 2002); soil reaction (pH), using the AS-23-NOM-021-RECNAT-2000 method (DOF, 2002); electrical conductivity (CE) from the saturation extract, using the AS-18 NOM-021 RECNAT-2000 method (DOF, 2002); soil texture, using the AS-09 NOM-021 REC-NAT-2000 method, Bouyouscos densimeter (DOF, 2002); soil infiltration, using the double ring infiltrometer; mechanical resistance to penetration (RMP), using the Yamanaka soil hardness tester (Carrasco et al., 2012); field capacity and the permanent wilting point, using the pressure cooker method (López-Ritas, 1967). These methods were used to determine the useful water percentage.
The statistical analysis was carried out using the SPSS® program, standard version 22 for Windows (SPSS Inc. Chicago, IL.). In order to prove the variances’ normality and homogeneity assumptions for the analyzed variables, the data was studied using the Lilliefors modification of the Kolmogorov-Smirnov test (Gómez et al., 2003). The variables were transformed to induce a normal distribution: the CE values were transformed into the inverse square; the MOS percentage was transformed into an arcsine square root of the proportion; the K values were transformed into an inverse square root; the pH values were raised to the power of ten, while the cumulative infiltration (IAc) and the RMP values were transformed into logarithms (McCune and Grace, 2002).
We determined the Spearman’s correlation coefficient to find out the relation between variables (Lahura, 2003); we used ANOVA to detect significant differences between treatments (Ott, 2001); and we compared means using the Tukey test (p≤0.05), to support the error rate credibility (Walpole et al., 1999).
Results and Discussion
For all treatments, soil texture was classified as clay loam soil, with 60-80 % clay, 20-45 % sand, and 20-50 % silt.
Spearman’s coefficient showed a significant negative correlation between apparent density, CE (-0.476), and MOS (-0.537), and a significant positive correlation between apparent density and pH (0.409). CE showed a significant positive correlation with MOS (0.701) and useful water (0.305), but it showed a negative correlation with pH (-0.401). MOS showed a significant negative correlation with pH (-0.292) and a positive correlation with useful water (0.235) (Table 1).
Table 1 Spearman’s correlation coefficients for the variables under study.

†Lower triangular and bilateral p values in the upper triangular (n=80). ¶DA: apparent density; CE: electrical conductivity; MOS: soil organic matter; pH: soil reaction; K: permeability; AU: useful water.
For the treatments’ average, the Spearman’s coefficient showed that DA had significant positive correlation with pH (0.636) and mechanical resistance to penetration (0.661), but it had a negative correlation with CE (-0.891) and MOS (-0.867). CE showed significant positive correlation with MOS (0.794) and negative correlation with RMP (-0.867), while pH showed a significant correlation with AU (-0.770) (Table 2).
Table 2 Spearman’s correlation coefficients for the treatments’ averages of the variables under study.

†Lower triangular and bilateral p values in the upper triangular (n=10). ¶Significance.
The analysis of variance (Table 3) indicated that with regard to model and treatment all variables showed significant differences, except AU percentage (p=0.108). With regard to the vegetation factor (FAa), the following variables showed significant differences: CE (p=0.006), MOS (p=0.007), and pH (p=0.001); meanwhile, with regard to the roller and fire treatment factors (FBb), all variables showed significant differences. The vegetation-treatment interaction (FA*FB) was significant for MOS (p=0.005), permeability (p=0.012), pH (p=0.031), and cumulative infiltration (p=0.001), while depth only showed significant differences for MOS (p=0.049) and pH (p=0.031).
Table 3 Analysis of variance for the two-criteria classification model (Treatment and Depth or Treatment and Season).

†DA: apparent density; CE: electrical conductivity; MOS: organic matter; K: permeability; pH: soil reaction; AU: useful water; IAc: cumulative infiltration; RMP: mechanical resistance to penetration.
The habitat enhancement methods (RA and IN) implemented in the rosette desert scrublands diminished soil apparent density (12-20 % in relation to control, with RA11 and IN11 treatments). When mycrophyllus desert scrublands were treated with RA11, apparent density diminished 6 % in relation to control, which matches the findings of Anriquez et al. (2005) about the DA reduction when a roller treatment was implemented in semi-arid ecosystems at Western Chaco, Argentina. These results are similar to those of Nandapure et al. (2011), who evaluated the long-term effects of integrated nutrient management on the physical properties of vertisol in Ankola, India; as well as to a Chinese study (Pei et al., 2008) about the changes in soil and vegetation properties in grazing and exclusion areas, in which DA was susceptible to management changes in the area.
The results indicate that during the first three years of the aerator roller treatment MOS and CE increase, while RMP, IAc, and pH decrease. Although MOS, K, pH, and CE values showed significant differences between treatments -according to the soil classification included in the Official Mexican Standard NOM-021-SEMARNAT-2000-, the values belong to the same category; therefore, we must accept that there are no significant differences as a result of the treatments. MOS and DA were the only variables that showed statistical differences, and -according to the Standard- they belong to another classification (Table 4). According to NOM-021-SEMARNAT-2000, pH and CE variables do not show category changes; these results match those obtained by Silva-Arrendondo et al. (2013) who analyzed the changes in the chemical properties of soil that has been impacted by intensive farming in northeastern Mexico; they also emphasized the importance of taking classifications into consideration, in order to detect changes that might be relevant for management purposes.
Table 4 NOM-021-SEMARNAT-2000 Classification.
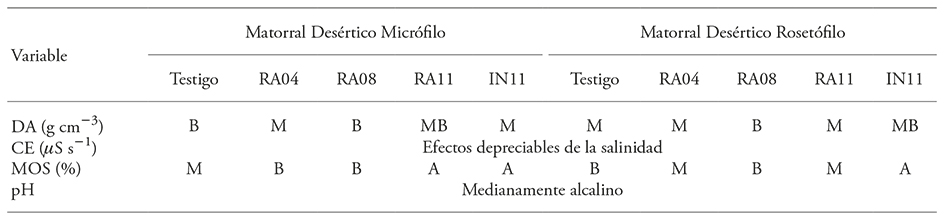
†MOS and DA classification: MB: very low; B: low; M: medium; A: high.
Rusan et al. (2007) evaluated the long-term effects on soil and plant soil quality variables in the irrigation of crops with wastewater, and found out that pH, Cu, Zn, Fe, and Mn concentrations did not change. Our study is similar, since pH did not change enough to involve two different categories in the standard used in the laboratory. Our results show that using a mechanical treatment (i.e., the aerator roller) is a feasible short-term alternative that increases permeability and organic matter in the soil, since the former shows a significant increase in the variable’s value in the rosette desert scrublands. However, after 8-10 years (2004 and 2008 treatments), MOS and IAc diminished, while DA and pH increased.
According to the Tukey test (p≤0.05) applied to the soil apparent density, there are two groups and one subgroup (Table 5). The MDMRA11 (1.00 g cm-3) and MDRIN11 (1.01 g cm-3) treatments are included in the group with the lowest soil compaction (group a), which has a very low classification. The MDRt (1.26 g cm-3), MDRRA04 (1.25 g cm-3), MDRRA08 (1.20 g cm-3), and MDMRA08 (1.19 g cm-3) treatments are included in the group with the highest soil compaction (group b). The MDMRA11 treatment showed the lowest apparent density, which may be the result of the “recent” soil removal caused by the blades of the aerator roller and the cover that it induces (Medina et al., 2016). This result is similar to the one obtained by Lozano et al. (2010) -who studied the effects of cover crops and grazing on the physicial properties of savannah soil-: lower soil densities occurred in induced covers, which may be the result of a greater soil cover.
Table 5 Means by treatment and results of Tukey test (p≤0.05).
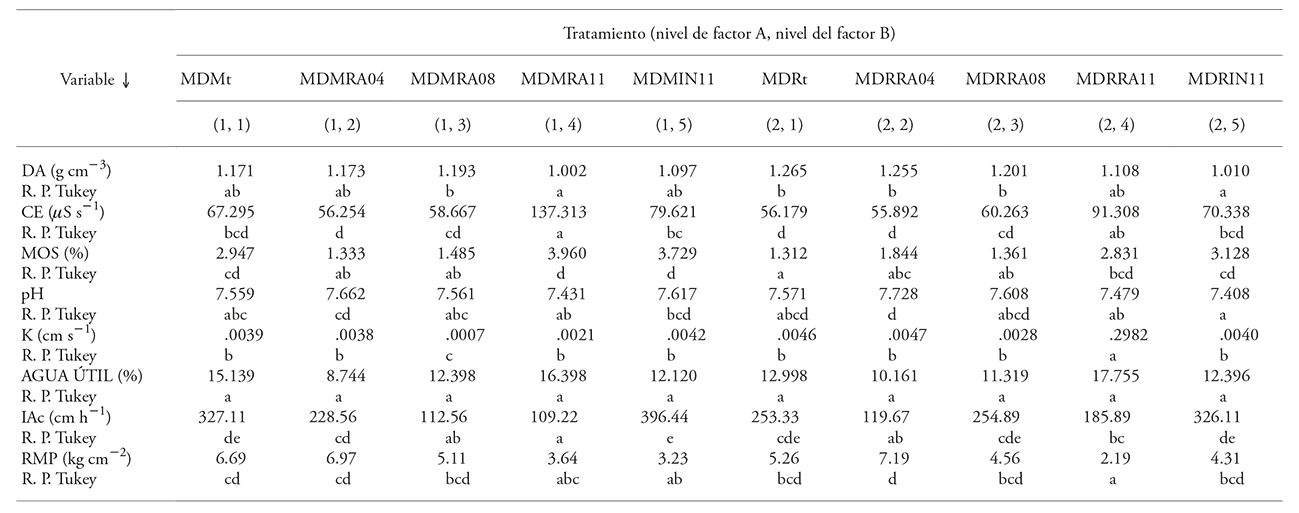
†Averages with different letters in a row are statistically different (Tukey p≤0.059).
CE showed two clearly defined groups and four subgroups: group a included the MDMRA11 treatment (137.3 µS s-1) with the highest CE, and group d included the MDMRA04 (56.3 µS s-1), MDRt (56.2 µS s-1), and MDRRA04 (55.9 µS s-1) treatments with the lowest CE. MOS showed two clearly defined groups and four subgroups: group a included the MDRt treatment (1.31 %) with the lowest MOS content, and group d included the MDMRA11 (3.96 %) and MDMIN11 (3.73 %) treatments with the highest MOS percentage. Soil management practices affect soil quality, nutrient quality, and chemical properties (Lauber et al., 2008; Zhang et al., 2013; Zhang et al., 2014). Additionally, Velasquez et al. (2007) studied soil quality indicators in Colombia and found out that MOS is a variable that indicates the quality and use given to the carbon that has been sequestered in the soil, since the amount of MOS tends to decrease, depending on soil use practices. The highest MOS percentage was observed in the MDRRA11 and MDRIN11 treatments, perhaps owing to the contribution of vegetable matter to the soil, as a result of the use of the roller and not from the vegetation cover and bush density. This result is similar to those of Demuner-Molina et al. (2014) who evaluated the effect of farming and soil enhancers in the humidity and radicular development and showed a greater crop output as a result of the enhancers that act as organic fertilizers. In contrast, Montaño-Arias et al. (2006) determined that low MOS contents are the result of the limited presence of bush vegetation.
Larrea tridentata favours fertile islands and accumulates under its canopy a greater concentration of nutrients, water holding capacity, and microbial activity (Celaya and Castellanos, 2011), which might explain the results obtained from RA11 and IN11 treatments in the mycrophyllous desert scrublands, where L. tridentata is one of the dominant species (Medina et al., 2016), and where the highest MOS content can be found; this is the result of the recent biomass contribution of this species to the soil, after the roller was used. In contrast, Kabzems and Haeussler (2005) did not find significant differences when they analyzed soil properties and vegetation response after MOS was removed. In our study, using the aerator roller did not have a positive short-term effect, because it increased MOS, but the increase was not sustained; in contrast, an agro-forestal system increases MOS content as times goes by (Murray et al., 2014). According to Martínez et al. (2008), MOS is responsible for soil properties, such as compaction, fertility, water retention, and structural stability. The results of our study are similar with regard to the relation between MOS and DA for all treatments; and MOS with K and IAc, in which there was a positive relationship between those variables for treatments RA04 MDM and IN11 treatments for both types of vegetation. pH showed two clearly defined groups and five subgroups: group a included the MDRIN11 treatment with the lowest pH, while group d included the MDRRA04 treatment with the highest pH.
The soil K value showed three clearly defined groups: group a included the MDRRA11 (0.298 cm s-1) treatment; group b included the MDMt (0.004 cm s-1), MDMRA04 (0.004 cm s-1), MDMRA11 (0.002 cm s-1), MDMIN11 (0.004 cm s-1), MDRt (0.005 cm s-1), MDRRA04 (0.004 cm s-1), MDRRA08 (0.003 cm s-1), and MDRIN11 (0.004 cm s-1) treatments; and group c included the MDMRA08 (0.007 cm s-1) treatment.
IAc showed two clearly defined groups and five subgroups: the MDMRA11 (109.22 cm h-1) treatment had the least cumulative infiltration and was included in group a; group e included the MDMIN11 (396.44 cm h-1) treatment which had the highest IAc. In both vegetation types, using a roller diminished IAc in the three treatments, in relation to the control; however, the MDMIN11 treatment showed the highest IAc in all treatments. These results differ from the ones obtained by Velásquez et al. (2011) with three treatments (continuous grazing, grazing exclusions, and aerator roller), but there were no differences in infiltration across sites or treatments.
Two groups and four subgroups were obtained for RMP: MD-RRA11 (2.19 kg cm-2) and MD-RRA04 (7.19 kg cm-2) were the treatments that showed less resistance to penetration, for group d and group a, respectively. The 2004 treatments (MDMRA04 and MDRRA04) caused an increase in RMP: 4 % in mycrophyllous scrublands, and 37 % in rosette scrublands, in relation to the controls. In contrast, in the 2008 roller treatments, both kinds of vegetation underwent a 24 % RMP reduction; the resistance was 50 % less in relation to control, when the RA11 treatment was used. In the IN11 treatment in a mycrophyllous scrubland, soil was 51 % less resistant to penetration, while resistance was 18 % lower with a rosette treatment, in relation to the control. Likewise, in relation to the control, using a roller diminished RMP up to 50 % in the mycrophyllous desert scrublands, and up to 59 % when the MDRRA11 treatment was used. These results match those of Ohep et al. (1998) who analyzed the effect of farming on the soil physical properties and found that there were significant differences with regard to DA, macroporosity, microporosity, and resistance to penetration for farming treatments. The similarity in results may be the consequence of ploughing the first soil stratum during farming and using the aerator roller.
Conclusions
In both desert scrublands, using an aerator roller is an efficient alternative method to manage the soil that increases the organic matter and reduces soil compaction during the first three years of the treatment. Additionally, during the first three years after the roller is used, water infiltration increases and mechanical resistance to soil penetration diminishes in rosette scrublands.
Fire is a feasible alternative that increases organic soil matter in both types of vegetation: cumulative infiltration increases in mycrophyllous desert scrublands, while soil compaction diminishes in rosette desert scrublands. However, fire has short-term benefits
Literatura Citada
Anaya, N. E., y H. Barral. 1995. La ganadería y su manejo en relación con los recursos agua y pastizal en la zona semi-árida de México. Aplicación al caso de la Hacienda Atotonilco y de la comunidad La Virgen, Durango. Manejo y uso del agua en las cuencas hidrográficas del norte de México. CENID-RASPA, ORSTOM Folleto Científico 5: 1-78. [ Links ]
Anriquez, A., A. Albanesi, C. Kunst, R. Ledesma, C. López, A. Rodríguez, R. Torresi, y J. Godoy. 2005. Rolado de fachinales y calidad de suelo en el Chaco occidental, Argentina. Cienc. Suelo 23: 145-157. [ Links ]
Ayala, F. A., B. F. G. Denogean, M. S. Moreno, A. Durán, B. Martínez, L. Barrera y E. Gerlach. 2014. Rehabilitación y Mejoramiento de Hábitat para la fauna silvestre. INVURNUS 9: 18-22. [ Links ]
Bhattacharyya, R., S. Kundu, S. C. Pandey, K. P. Singh, and H. S. Gupta. 2008. Tillage and irrigation effects on crop yields and soil properties under the rice-wheat system in the Indian Himalayas. Agric. Water Manage. 95: 993-1002. [ Links ]
Carrasco, S. J., N. F. Squella, S. J. Riquelme, C. J. Hirzel, y C. H. Uribe. 2012. Técnicas de conservación de suelos, agua y vegetación. Investigaciones Agropecuarias, Centro Regional de Investigación Rayentué, Rengo, Chile. Serie Actas 48: 1-210. [ Links ]
Carter, M. R. 2002. Soil quality for sustainable land management. Agron J.. 94: 38-47. [ Links ]
Casanova, F., L. Ramírez, y F. Solorio. 2007. Interacciones radiculares en sistemas agroforestales: mecanismos y opciones de manejo. AIA 11: 41-52. [ Links ]
Casas, N., y M. Manzan. 2009. Evaluation of the use of roller aerator for the rehabilitation of grazing lands and content of carbon in arid areas of northeastern Mexico. In: VI Simposio Internacional de Pastizales 4 al 7 de Nov. Universidad Autónoma de Nuevo León - Instituto Tecnológico de Estudios Superiores de Monterrey. Monterrey, N. L. s/p. [ Links ]
Celaya, M. H., y A. E. Castellanos V. 2011. Mineralización de nitrógeno en el suelo de zonas áridas y semiáridas. Terra Latinoam. 29: 343-356. [ Links ]
Certini, G. 2005. Effects of fire on properties of forest soils: a review. Oecología 143: 1-10. [ Links ]
Cjpek, J., V. Kuráž, and J. Fourz. 2012. Hydrological properties of soils in reclaimed and unreclaimed sites after Brown-Coal mining. Polish J. Environ. Studies 22: 645-652. [ Links ]
Cydzik, K., and T. S. Hougue. 2009. Modeling postfire response and recovery using the hydrologic engineering center hydrologic modeling system (HEC-HMS). J. Am. Water Resour. Assoc. 45: 702-714. [ Links ]
Darwish O. H., N. Persaud, and D. C. Martens. 1995. Effect of long-term application of animal manure on physical properties of three soil. Plant Soil 176: 289-295. [ Links ]
Das, B. M. 1997. Soil Mechanics Laboratory Manual, Fifth Edition. Engineering Press, Austin, Texas. 78720-0129. pp: 5-23. [ Links ]
Demuner-Molina, G., M. Cadena-Zapata, S. G. Campos-Magaña, A. Zermeño-González, y F. J. Sánchez-Pérez. 2014. Efecto de labranza y mejoradores de suelo en humedad y desarrollo radicular. Tecnología y Ciencias del Agua. V: 123-130. [ Links ]
DOF, 2002. Diario Oficial de la Federación. Norma Oficial Mexicana NOM-021-RECNAT-2000. Martes 31 de Diciembre. 85 p. [ Links ]
Eilers, K. G., S. Debenport, S. Anderson, and N. Fierer. 2012. Digging deeper to find unique microbial communities: The strong effect of depth on the structure of bacterial and archaeal communities in soil. Soil Biol. Biochem. 50: 58-65. [ Links ]
Estrada-Castillón, E., J. R. Arévalo, J. A. Villarreal-Quintanilla, M. M. Salinas-Rodríguez, J. A. Encina-Domínguez, H. González-Rodríguez, and C. M. Cantú-Ayala. 2014. Classification and ordination of main plant communities along an altitudinal gradient in the arid and temperate climates of northeastern Mexico. Sci. Nature 102: 58-68 [ Links ]
Gómez, G. M., C.Danglot B., y L. Vega F. 2003. Sinopsis de pruebas estadísticas no paramétricas. Cuándo usarlas. Rev. Mex. Pediatr. 70: 91-99. [ Links ]
Hillel, D. 2004. Encyclopedia of Soils in the Environment: London, Elsevier. pp: 295-303. [ Links ]
Huang, Z. L., L. D. Chen, B. J. Fu, Y. H. Lu, Y. L. Huang, and J. Gong. 2006. The relative efficiency of four representative cropland conversions in reducing water erosion: evidence from long-term plots in the loess Hilly Area, China. Land Degrad. Dev. 17: 615-627. [ Links ]
INE-SEMARNAT, 1997. Programa de Manejo del Área de Protección de Flora y Fauna “Maderas del Carmen”, México. 1ª. Ed. 127 p. [ Links ]
Jia, X. X., M. A. Shao, X. R. Wei, R. Horton, and X. Z. Li. 2011. Estimating total net primary productivity of managed grasslands by a state-space approach in a small catchment on the Loess Plateau, China. Geoderma 160: 281-291. [ Links ]
Kabzems, R., and S. Haeussler. 2005. Soil properties, aspen, and white spruce responses 5 years after organic matter removal and compactation treatments Can. J. Forest Res. 35: 2045-2055. [ Links ]
Lahura, E. 2003. El coeficiente de correlación y correlaciones espúreas. Documento de trabajo 218. http://departamento.pucp.edu.pe/economia/documento/el-coeficiente-de-correlacion-y-correlaciones-espureas/ (Consulta: enero 2014). [ Links ]
Lauber, C. L., M. S. Strickland, M. A. Bradford, andN. Fierer . 2008. The influence of soil properties on the structure of bacterial and fungal communities across land-use types. Soil Biol. Biochem . 40: 2407-2415. [ Links ]
Li, X., F. Li, R. Zed, Z. Zhan, and B. Singh. 2007. Soil physical properties and their relations to organic carbon pools as affected by land use in an alpine pastureland. Renew. Agr. Food Syst. 21: 26-35. [ Links ]
López-Ritas, J. 1967. El Diagnóstico de Suelos y Plantas: Métodos de Campo y Laboratorio. Madrid. Mundi - Prensa. 333 p. [ Links ]
Lozano, Z., H. Romero, y C. Bravo. 2010. Influencia de los cultivos de cobertura y el pastoreo sobre las propiedades físicas de un suelo de sabana. Agrociencia 44: 135-146. [ Links ]
Maass, J. M. 1998. La erosión de suelos en México: una consecuencia de la transformación del hábitat y uno de los problemas más serios de la degradación ambiental. In: Toledo, G., y M. Leal (eds). Destrucción del Hábitat. PUMA, Universidad Nacional Autónoma de México. México, D.F. pp: 271-285. [ Links ]
Martínez, E.H., J.P.Fuentes E., y E.Acevedo H. 2008. Carbono orgánico y propiedades del suelo. J. Soil Sc. Plant Nutr. 8: 68-96. [ Links ]
McCune, B., and J. A. B. Grace. 2002. Analysis of Ecological Communities. 2ª. Ed. MjM Software Design, Oregon, USA. 304 p. [ Links ]
McKinney, B. R., P.Robles Gil, R. Skiles, V. J. Delgadillo, y J. Rojo. 2006. El Carmen-Big Bend: Un innovador modelo de alianzas públicas y privadas para la conservación transfronteriza. In: Mittermeier, R., C.F. Kormos, C.G. Mittermeier, P.Robles Gil, T.Sandwith, y C.Besaçon (eds). Conservación Transfronteriza: Una Nueva Visión para Áreas Protegidas. CEMEX, Monterrey, México. pp: 101-112. [ Links ]
Medina, G.R., I.Cantú S., E.Estrada C ., H.González R., y J.A.Delgadillo V. 2016. Cambios en la vegetación del matorral desértico micrófilo en un área bajo manejo. Rev. Mex. Cien. For. 6: 37-48. [ Links ]
Molinar-Holguín, F., H. Souza-Gomes, J. Holechek L., y R. Valdez. 1998. México, Macro-economía y manejo de pastizales. Rangelands 20: 16-24. [ Links ]
Montaño-Arias, N. M., R. García S., G. Ochoa R., y A. Monroy A. 2006. Relación de la vegetación arbustiva, el mezquite y el suelo de un ecosistema semiárido en México. Terra Latinoam . 24: 193-205. [ Links ]
Murray, R. M., M. G. Orozco, A. Hernández, C. Lemus, y O. Nájera. 2014. El sistema agroforestal modifica el contenido de materia orgánica y las propiedades físicas del suelo. Avances Inv. Agropec. 18: 23-31. [ Links ]
Nandapure, S. P., B. A. Sonune, V. V. Gabhane, R. N. Katkar, and R. T. Patil. 2011. Long term effects of integrated nutrient management on soil physical properties and crop productivity in sorghum-wheat cropping sequence in a vertisol. Indian J. Agric. Res. 45: 336-340. [ Links ]
Ohep, C., F. Marcano, y O. Sivira. 1998. Efecto de la labranza sobre las propiedades físicas del suelo y el rendimiento del frijol (Vigna unguiculata L. Walp) en el Yaracuy medio. Bioagro 10: 68-75. [ Links ]
Ott, R. L. 2001. An Introduction to Statistical Methods and Data Analysis. 5a Ed. Duxbury Thomson Learning. Michael Longnecker. Texas A & M University. U.S.A. 1152 p. [ Links ]
Pei, S., H. Fu, and C. Wan. 2008. Changes in soil properties and vegetation following exclosure and grazing in degraded Alxa desert steppe of Inner Mongolia, China. Agric. Ecosyst. Environ. 124: 33-39. [ Links ]
Pennock, D., T. Yates, and J. Braidek. 2008. Soil sampling designs. In: Carter, M. R., and E. G. Gregorich (eds). Soil Sampling and Methods of Analysis. 2nd. Ed. Taylor & Francis Group. LLC. 25-39 p. [ Links ]
Rasoulzadeh, A., and A. Yaghoubi. 2014. Inverse modeling approach for determining soil hydraulic properties as affected by application of cattle manure. Int. J. Agric. Biol. Eng. 7: 27-35. [ Links ]
Rumpel, C., and I. Kögel-Knabner. 2011. Deep soil organic matter a key but poorly understood component of terrestrial C cycle. Plant Soil 338: 143-158. [ Links ]
Rusan, M. J. M., S. Hinnawi, and L. Rousan. 2007. Long term effect of wastewater irrigation of forage crops on soil and plant quality parameters. Desalination 215: 143-152. [ Links ]
Silva-Arredondo, F., M. Pando-Moreno, H. Gonzalez-Rodriguez, and L. Scott-Morales. 2013. Changes in the chemical properties of a soil impacted by intensive agriculture, North-eastern Mexico. Int. J. Bio-resource Stress Manage. 4: 126-131. [ Links ]
Six, J., H. Bossuyt, S. D. Degryze, and K. Denef. 2004. A history of research on the link between (micro) aggregates, soil biota, and soil organic matter dynamics. Soil Tillage Res. 79: 7-31. [ Links ]
Spedding, T. A., C. Hamel, G. R. Mehuys, and C. A. Madramootoo. 2004. Soil microbial dynamics in maize-growing soil under different tillage and residue management systems. Soil Biol. Biochem . 36: 499-512. [ Links ]
SPP. Secretaría de Programación y Presupuesto. 1982a. Carta Estatal de Edafología. Dirección General de Geografía del Territorio Nacional. Cartas H 13-9 y H13-12. Esc: 1: 250,000. [ Links ]
SPP. Secretaría de Programación y Presupuesto. 1982b. Carta Estatal de Geología. Dirección general de Geografía del Territorio Nacional. Cartas H 13-9 y 13-12. Esc: 1: 250,000. [ Links ]
SPP. Secretaría de Programación y Presupuesto. 1983. Síntesis Geográfica de Coahuila y anexo cartográfico. s/p. [ Links ]
Swędrzyńska, D., I. Malecka, A. Blecharezyk, A. Swędrzyński, and J. Starzyk. 2013. Effects of various long-term tillage systems on some chemical and biological properties of soil. Polish J. Environ. Studies 22: 1835-1844. [ Links ]
Velasquez E., P. Lavelle, and M. Andrade. 2007. GISQ, a multifunctional indicator of soil quality. Soil Biol. Biochem . 39: 3066-3080. [ Links ]
Velásquez, V. M. A., R. Gutiérrez L., and A. Muñoz. 2011. Vegetation management and their impact on the soil hydrological response in a semiarid rangeland. Ing. Agríc. Biosist. 3: 17-23. [ Links ]
Walpole, R. E., H. R. Myers, y L. S. Myers. 1999. Probabilidad y Estadística para Ingenieros. 6ª Ed. Prentice Hall Hispanoamericana. México. 752 p. [ Links ]
Wang, L., Y. Mu, Q. Zhang, and Z. I. Jia. 2012. Effects of vegetation restoration on soil physical properties in the wind-water erosion region of the Northern Loess Plateau of China. Clean-Soil Air Water 40: 7-15. [ Links ]
Zhang, Z. H., X. Y. Li, Z. Y. Jiang, H. Y. Peng, L. Li, and G. Q. Zhao. 2013. Changes in some soil properties induced by re-conversion of cropland into grassland in the semiarid steppe zone of Inner Mongolia, China. Plant Soil 373: 89-106. [ Links ]
Zhang, P., T. Wei, Z. Jia, Q. Han, X. Ren, and Y. Li. 2014. Effects of Straw incorporation on soil organic matter and soil water-stable aggregates content in semiarid regions of northwest China. PLoS ONE 9: 1-11. [ Links ]
Received: November 2016; Accepted: February 2017