Serviços Personalizados
Journal
Artigo
Indicadores
-
Citado por SciELO
-
Acessos
Links relacionados
-
Similares em SciELO
Compartilhar
Agrociencia
versão On-line ISSN 2521-9766versão impressa ISSN 1405-3195
Agrociencia vol.51 no.1 Texcoco Jan./Fev. 2017
Plant Protection
Trichoderma spp. native strains molecular identification and in vitro antagonistic evaluation of root phitopathogenic fungus of the common bean (Phaseolus vulgaris L.) cv. Montcalm
1Instituto Tecnológico de Roque. Km 8 Carretera Celaya-Juventino Rosas. 38110. Celaya, Guanajuato, Mexico.
2Colegio de Postgraduados, Instituto de Fitosanidad Campus Montecillo, Km. 36.5 Carretera México-Texcoco. 56230. Montecillo, Texcoco, Estado de México, Mexico.
3Universidad Politécnica de Guanajuato, Laboratorio de Fitopatología. Avenida Universidad Sur 1001. 38483. Localidad Juan Alonso, Cortazar, Guanajuato. Mexico.
4Instituto Nacional de Investigaciones Forestales, Agrícolas y Pecuarias, Región Noreste, Campo Experimental General Terán. Km 31 Carretera Montemorelos-China. 67400. General Terán, Nuevo León, Mexico.
Trichoderma spp. is a fungus of the Ascomycota division with great potential to control diseases in economically important crops. This antagonistic fungus inhibits the growth of phytopathogenic microorganisms, including those responsible for root diseases, such as Rhizoctonia spp. and Fusarium spp. The objective of this study was to identify Trichoderma species and to describe their in vitro antagonistic activity in pathogenic strains of Rhizoctonia solani, F. oxysporum, F. verticillioides and F. solani, with different virulence determined based on pathogenicity tests in bean plants (Phaseolus vulgaris L.) cv. Montcalm. The hypothesis was that the identification and antagonistic description of Trichoderma species against pathogenic fungi defines their biological control efficiency. The experimental design was completely random, with at least three repetitions. The molecular identification of Trichoderma spp. was done through ITS1 and ITS2 regions, separated by the 5.8S ribosomal RNA gene (ITS, Internal Transcribed Spacer). The antagonism was evaluated using the modified cellophane method and dual cultures to estimate the percentage of radial growth inhibition (PRGI). Seven Trichoderma strains identified using molecular techniques showed 95-100 % identity to T. harzianum and T. asperellum. Trichoderma strains showed high antagonistic capacity with 73.1 to 76.4 mean PRGI values in pathogenic strains, suggesting an antibiosis effect (77.6 PRGI) and different hyphal interaction types. Antagonistic strains were statistically similar for pathogen growth and development inhibition; however, T. asperellum’s antagonistic values were higher than T. harzianum’s with regard to all pathogenic strains, even R. solani, which was the most aggressive pathogen for cv. Montcalm (mean value: 7.0). The antagonistic effect of Trichoderma strains on phytopathogenic fungi was determined with in vitro tests and corroborated with in vivo bioassays with similar inhibition percentages.
Key words: Trichoderma spp.; Rhizoctonia solani; Fusariumox ysporum; F. verticillioides; F. solani; antagonism; mycoparasitism
Trichoderma spp. es un hongo de la división Ascomycota con gran potencial para controlar enfermedades en los cultivos con importancia económica. Este hongo antagonista inhibe el crecimiento de microorganismos fitopatógenos, entre los que destacan los causantes de enfermedades radiculares, como Rhizoctonia spp. y Fusarium spp. El objetivo de este estudio fue identificar especies de Trichoderma y caracterizar su actividad antagónica in vitro en cepas patogénicas de Rhizoctonia solani, F. oxysporum, F. verticillioides y F. solani con virulencia diferente, determinada a partir de pruebas de patogenicidad en plantas de frijol (Phaseolus vulgaris L.) cv. Montcalm. La hipótesis fue que la identificación y caracterización antagónica de especies de Trichoderma contra hongos patógenos define su eficiencia en el control biológico. El diseño experimental fue completamente al azar, con al menos tres repeticiones. La identificación molecular de Trichoderma spp. se realizó a través de regiones ITS1 e ITS2 separadas por el gen 5.8S del rRNA ribosomal (ITS, Internal Transcribed Spacer). El antagonismo se evaluó con la prueba de papel celofán modificada y cultivos duales para estimar el porcentaje de inhibición de crecimiento radial (PICR). Siete cepas de Trichoderma identificadas por técnicas moleculares mostraron 95 a 100 % de identidad a T. harzianum y T. asperellum. Las cepas de Trichoderma expresaron capacidad antagónica alta con valores medios de PICR de 73.1 a 76.4 en las cepas patogénicas, lo que sugiere efecto de antibiosis (77.6 PICR) y diferentes tipos de interacción hifal. Las cepas antagonistas fueron estadísticamente similares en la inhibición del crecimiento y desarrollo de los patógenos, pero T. asperellum obtuvo valores de antagonismo superiores a T. harzianum con todas las cepas patogénicas, incluso R. solani que fue el patógeno más agresivo para el cv. Montcalm (valor medio 7.0). El efecto antagónico de las cepas de Trichoderma en los hongos fitopatógenos pudo determinarse con pruebas in vitro y corroborado en bioensayos in vivo, con porcentajes de inhibición similares.
Palabras clave: Trichoderma spp.; Rhizoctonia solani; Fusariumox ysporum; F. verticillioides; F. solani; antagonismo; micoparasitismo
Introduction
Methods for disease control include the use of resistant genotypes, cultural practices, volatile compounds, and plant extracts, as well as chemical and biological control (Nashwa et al., 2008). Chemical control is one of the most used methods and, in most cases, pollutes the environment, it is expensive, and its control is not selective; this affects the survival of antagonistic microorganisms. Biological control is an efficient and economical complementary alternative for the control of foliar diseases and those caused by soil pathogens (Infante et al., 2009). The Trichoderma genus includes several species that serve as biological control agents, which stimulates crops and induces systemic resistance to different pathogens in plants (Harman, 2004). The identification and description of this species are crucial for its proper application in the biological control of specific pathogens in agricultural crops. The morphological identification methods of Trichoderma species have been reinforced with molecular techniques, such as the identification of the ITS1 and ITS2 regions separated by the 5.8S ribosomal RNA gene (ITS, Internal Transcribed Spacer), resulting in the strain reclassification of this fungus (White et al., 1990; Kullnig et al., 2001; Druzhinina et al., 2006). T. harzianum strains were relocated in species such as T. asperellum, T. atroviride, and T. longibrachiatum (Lieckfeldt et al., 1999; Hermosa et al., 2000; Kullnig et al., 2001), while T. atroviride or T. viride were relocated as T. asperellum (Watanabe et al., 2005).
Trichoderma is as an antagonistic fungus, with growth and disease control mechanisms that affect pathogens: direct competition for space and nutrients; production of metabolites and antibiotics; and direct parasitism in fungi (mycoparasitism) (Ezziyyani et al., 2004a). These fungal species are used all over the world to control fungous diseases such as Trichoderma harzianum, T. asperellum, T. atroviride, T. gamsii, and T. polysporum (Samuels et al., 2010; Schuster and Schmoll, 2010), because they are easily isolated and they grow quickly in different substrates (Howell, 2003; Ezziyyani et al., 2004a; González et al., 2012). However, antagonistic fungi (such as Trichoderma spp.) have a limited control over pathogenic fungi that cause root rot, because the antagonist-pathogen interactions have not been described. Phytopathogenic fungi that cause root rot in economically important crops are part of a consortium made up of the Fusarium spp., Rhizoctonia sp., Sclerotium sp. and Pythium sp. genera (Bolkan, 1980). Fusarium spp. species adapt and develop in a wide variety of conditions; therefore, their elimination or control can be a great challenge (Mitra and Lele, 1981).
Our hypothesis was that the identification and antagonistic description of Trichoderma spp. in pathogenic fungi, which cause root rot, defines its biological control efficiency. This study had three objectives: 1) to identify the species of the Trichoderma strains through the amplification of the ITS1 and ITS2 regions, separated by 5.8S ribosomal RNA gene (ITS, Internal Transcribed Spacer); 2) to identify the amplicon sequencing and to describe the strains based on their antagonistic activity in pathogenic strains of bean crops (Phaseoulus vulgaris L.), such as R. solani, Fusarium oxysporum, F. verticillioides, and F. solani with different aggressiveness; and, 3) to provide information to determine if the Trichoderma antangonistic strains efficiently control the pathogenic fungi of this crop’s root.
Materials and Methods
Obtaining fungal isolates from Fusarium spp., Rhizoctonia solani, and Trichoderma spp.
Soil sampling was carried out in a field cultivated with corn and bean in the spring-summer 2013 cycle, at the Bajío Experimental Field (CEBAJ) of the Instituto Nacional de Investigaciones Forestales, Agrícolas y Pecuarias (INIFAP), Celaya, Guanajuato (20° 34’ 33.44” N, 100° 4’ 19.24” W, and 1775 masl).
Sampling (90 samples of approximately 250 g) was performed using the zigzag method, with soil sample taken at 5, 10, and 15 cm deep. The samples were diluted 1:1000 in double distilled water and 0.25 mL of that dilution were placed in sterile Petri dishes (Star®Dish) with a water-agar medium (2%, Bioxon®) (WA). The dishes were kept under natural light and darkness conditions, at 23-25 °C. They were analyzed after 48 and 72 h with a compound microscope, with 40X-400X magnification lenses (Leica CME®), in order to observe spore germination or fungus colonies formation. A fragment of the colony or hyphal tip was extracted from the developed fungus, and transferred to Petri dishes with a potato-dextrose-agar medium (PDA, Bioxon®), in order to purify, identify, and preserve them.
Strain purification, identification, and conservation
The isolates were purified using a monoconidial culture and a conidial suspension in WA medium. After 24 h, we observed the germination of conidium. Using a compound microscope, a germinated conidium was extracted and cultured in a PDA medium. The morphology of the Rhizoctonia, Fusarium, and Trichoderma genera was identified with Barnett and Hunter’s morphological dichotomous keys (1988). The species of the Fusarium genus strains were identified with the keys of Nelson et al. (1983). Only the seven Trichoderma strains were identified with molecular techniques, amplyfing the ITS1 and ITS2 regions, separated by 5.8S ribosomal RNA gene (ITS, Internal Transcribed Spacer) (White et al., 1990; Kullnig et al., 2001; Druzhinina et al., 2006). PCR amplification was performed with ITS4 and ITS5 primers (Bellemain et al., 2010). The amplified fragments were sequenced at the Integral Phytosanitary Diagnostic Laboratory of the College of Postgraduates (Montecillos Campus, Texcoco, Estado de Mexico).
Strains of Fusarium spp., R. solani, and Trichoderma spp. were preserved placing colony discs, in 15 mL Falcon® tubes, with 100% mineral oil, at 4 °C. The seven Trichoderma strains and one R. solani strain were kept in an active state in Petri dishes with PDA and five Fusarium strains were kept in a synthetic low-nutrient agar culture medium (SNA: Spezieller Nährstoffarmer Agar) (0.2 g L-1 glucose, 0.2 g L-1 sucrose, 1.0 g L-1 KH2PO4, 1.0 g L-1 KNO3, 0.5 g L-1 MgSO4 ∙7H2O, 0.5 g L-1 KCl, 0.6 mL L-1 1 N NaOH, 23.0 g L-1 agar) (Nirenberg, 1976).
Pathogenic and antagonistic strains
Five strains of the Fusarium genus (Fsol-1, Fvert-4, Fsol-9, Foxy-23, Foxy-43) and one of R. solani were analyzed. The selection of pathogenic strains was established by means of pathogenicity tests in cv. Montcalm bean plants, which are susceptible to root rot (Schneider and Kelly, 2000; Cichy et al., 2007; Michcrop.com, 2016). In a greenhouse, cv. Montcalm seeds were germinated in trays with peat (Sunhine 3 Mix®). Fifteen days after they were sowed, the plants were removed from the trays, the substrate was removed with water, and a third of the root was cut off. Ten plants of each Fusarium spp. strain were immersed in an inoculum with 25 mL-1 conidia (Schneider y Kelly, 2000) during 4 minutes (Castellanos et al., 2011). The plants were transplanted into 1 L pots with peat. Wheat grains infested with the R. solani strain were used as inoculum. Fifteen days after germination, five grains were applied as colony-forming units (CFU), for each of the 10 bean plants (Mora, 1996). The inoculated plants were kept in a greenhouse, at 28-32 °C, with natural light and they were watered every 3 days. The control plants were non-inoculated cv. Montcalm. Plant evaluation for both pathogens was performed 28 days after inoculation, using the CIAT scale (plants with values from 1 to 3 were considered resistant, 4 to 6 moderately susceptible, and 7 to 9 susceptible) (CIAT, 1987).
Seven Trichoderma strains were tested (Tri-1, Tri-2, Tri-3, Tri-4, Tri-5, Tri-6, and Tri-7). Six of them were obtained from the sampled soil (Tri-1, Tri-2, Tri-3, Tri-4, Tri-6, and Tri-7), and one (Tri-5) was provided by CINVESTAV (Irapuato Unit).
Antibiosis of Trichoderma spp.
The preselection of Trichoderma strains’ capacity to inhibit phytopathogenic fungi was carried out using the modified cellophane method (Dennis and Webster, 1971), with a random design and three repetitions (each one in a separate Petri dish). The test determines Trichoderma’s ability to inhibit the mycelium growth of the Fusarium spp. and R. solani strains. Petri dish-size (90 mm diameter), autoclave-sterilized (121 °C for 15 min) circles of Whatman® filter paper, were placed in PDA culture media under aseptic conditions. A 7-mm diameter disc of each Trichoderma strain was placed in the centre of the dish. The dishes were kept at 22-25 °C for 48 h. Afterwards, the paper circle was carefully removed. The center of the same Petri dish was inoculated with another disc with a phytopathogenic fungus, the dishes were kept at the same temperature and for the same period. Radial growth (mm) was measured everyday, until the control (pathogen inoculated in Petri dishes, without Whatman® filter paper) filled the dish. The percentage of radial growth inhibition (PRGI) of Fusarium and R. solani was calculated using the following formula: PICR = [(D1-D2)/D1] X 100 (Worasatit et al., 1994). In this formula, D1 is the pathogen colony diameter in PDA without Trichoderma and D2 is the pathogen colony diameter in the dish where Trichoderma had previously grown on Whatman® filter paper. The isolates with 55 %+ percentages were selected for the in vitro comparison test (Michel-Aceves et al., 2005), in order to define their antagonistic potential.
The Trichoderma strain mycoparasitism was determined by the colonized Petri dish surface (in dishes with prior phytopathogen presence), according to the Bell et al. scale (1982), where: 1) Trichoderma colonizes 100 % of the medium surface and grows over the phytopathogen; 2) Trichoderma colonizes two thirds of the culture medium surface and restricts the phytopathogen growth; 3) Trichoderma and phytopathogen colonize half of the surface each (neither fungi dominates); 4) phytopathogen colonizes two-thirds of the medium surface and restricts the growth of Trichoderma; and 5) phytopathogen colonizes 100 % of the medium surface and grows over Trichoderma.
Antagonistic potential of pre-selected Trichoderma isolates
Comparisons between the seven Trichoderma strains and the six pathogen strains were performed in triplicate, pairing cultures in Petri dishes with PDA. A 7-mm diameter colony disc of each Trichoderma strain and another phytopathogenic fungus colony disc were placed equidistant from each other’s microorganisms (at ±6.0 cm).
The radial growth of both colonies was measured every 24 h, in order to determine the number of days required for the first contact between the antagonist’s and the pathogen’s hyphae. The antagonism level was classified using the Bell et al. scale (1982).
Statistical analysis
The data from each bioassay were analyzed with ANOVA and Duncan’s new multiple range test (MRT) that compares differences between means (p≤0.05). Unrelated treatments were compared in order to establish a merit order, using the SAS statistical software, version 9.1 (SAS Institute Inc., 2009).
Phylogenetic analysis
ITS sequences were analyzed using BLAST (Basic Local Alignment Search Tool) (Morgulis et al., 2008) at the NCBI (National Center for Biotechnology Information). The Trichoderma phylogenetic trees were developed using the Neighbor-Joining statistical method and the Kimura-2-parameter model; bootstrap values were calculated from 1000 replications (Chaverri et al., 2003). Multiple sequence alignment was performed with Clustal W of the MEGA 7.0 software (Molecular Evolutionary Genetics Analysis) (Kumar et al., 2016).
Results and Discussion
Out of the 90 soil samples, we were able to identify the species of 42 isolates of the Fusarium spp., R. solani, and Trichoderma spp. genera.
Pathogens and antagonists identification
Fusarium and Rhizoctonia solani identification
Six phytopathogenic fungus strains: F. solani (Fsol-1 and Fsol-9), F. oxysporum (Foxy 23 and Foxy-43), and F. verticillioides (Fvert-4), were analyzed using the keys of Nelson et al. (1983) and R. solani was analyzed according to Barnett and Hunter’s dichotomous keys (1988) (Figure 1).
Trichoderma’s morphological identification
Out of the seven strains, five were identified as T. harzianum and two as T. asperellum, according to their morphology. The following phenotypic traits were found in all strains: hyaline colored conidiophores, ovoid unicellular conidia, small hyaline or yellowish green terminal clusters, and colouring of the colony at the core of the PDA culture medium. The strains were designated Tri-1 to Tri-7.
The Tri-1 and Tri-2 strains colonies showed a yellowish-white color and cottony appearance; in contrast, the Tri-3 and Tri-4 strains were white. Only Tri-3 formed cottony-looking mycelium. Tri-5 was the only one that showed a yellowish-green color, while Tri-6 and Tri-7 showed a yellow color and flat mycelium growth (Figure 2). This agrees with the results described by Infante et al. (2009), who indicated that most Trichoderma colonies were white, some turned dark green, yellowish green or yellow; the mycelium growth of some strains was sparse and very thin, and its appearance was that of a flat colony (the printed versión shows figures in gray shades and the eletronic version shows in color.
Trichoderma molecular identification
DNA sequence analysis for the molecular identification of Trichoderma species showed a high percentage of identity with several T. asperellum and T. harzianum samples. Tri-3 and Tri-5 had a 100 % match with T. asperellum; the rest of the strains showed 95 and 100 % identity with T. harzianum (Table 1).
Table 1 Morphological and molecular identification of the ITS1 and ITS2 regions in seven Trichoderma strains. E-value for all strains=0.0.

*National Center for Biotechnology Information.
In the past, identification and description were based on morphological features. Now, molecular analysis (such as rRNA sequencing) enables greater accuracy in species identification (Martin and Rygiewicz, 2005; Watanabe et al., 2005; Guigón-López et al., 2010). The taxonomic location of the strains is fundamental because it is the first step towards the improvement of the evaluation design of their biological activity and the determination of the development of their action mechanisms. As a whole, this information enables an efficient selection of the organisms that could be used for the biocontrol of plant pathogens, due to their antagonistic function.
The dendrogram grouped the seven Trichoderma strains in two clusters: 1) T. asperellum (Tri-3 and Tri-5), and 2) T. harzianum (Tri-1, Tri-2, Tri-4 and Tri-7) (Figure 3). The presence of T. harzianum (71.42 %) prevailed over T. asperellum (28.57 %). This may be expected, since T. harzianum is the most frequently isolated species in the world, due to its cosmopolitan adaptation and distribution (Chaverri and Samuels 2003; Sosa et al., 2014).
Pathogenicity tests with Fusarium and R. solani
Bean plants inoculated with Fusarium spp. and R. solani pathogens showed different pathogenicity, according to the CIAT visual scale (CIAT, 1987). The plants reaction to Rsol-1 was “susceptibility” (average: 7): the plants inoculated with Foxy-23, Foxy-43, and Fsol-1 were moderately susceptible (average: 6); meanwhile, those inoculated with Fsol-9 and Fvert-4 were moderately susceptible (4 and 6, respectively). Control plants showed no root or hypocotyl damage (Figure 4).
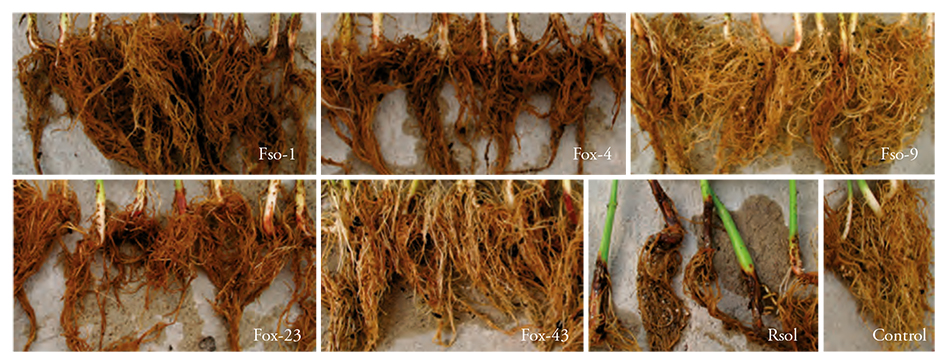
Figure 4 Fusarium spp. and Rhizoctonia solani pathogenicity in cv. Montcalm bean. Fso-1 and Fso-9: F. solani, Fox-4: F. verticillioides, Fox-23 and Fox-43: F. oxysporum, Rsol: R. solani and control: no inoculum. The plants show damage levels based on root and hypocotyl rot and density, 28 days after inoculation. Control plants with no hypocotyl and root damage showed normal root density.
Pre-selection of antagonistic strains: antibiosis
Metabolites -such as chitinases, glucanases, and other resistance proteins- produced by antagonistic Trichoderma strains in the culture medium inhibit the growth of phytopathogenic fungi (Elad et al., 1982; Baker and Griffin, 1995; Elad, 2000; El-Katatny et al., 2001). That growth is evaluated as an inhibition percentage with regard to mycelium growth. The presence of the mechanism is demonstrated by the inhibition percentage of the Fusarium and R. solani pathogens’ mycelium growth caused by Trichoderma. In this study, the average inhibition of mycelium growth varied between 72.3 and 87.5%, and antagonism was identified as type 2 (Table 2).
Table 2 Inhibition (%) of the radial growth of Fusarium oxysporum, F. verticillioides, F. solani, and Rhizoctonia solani by Trichoderma.
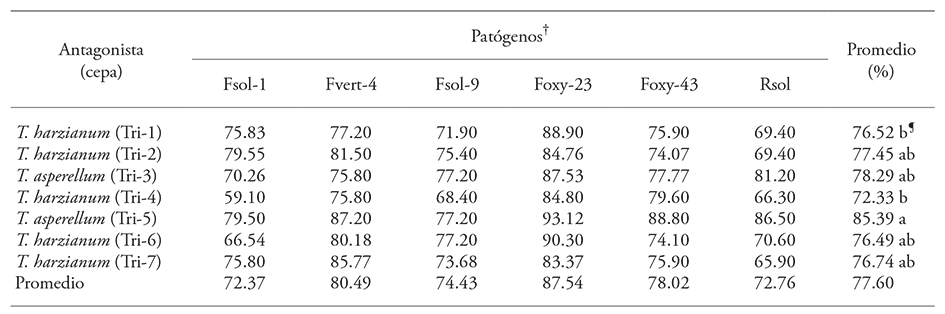
†Fsol: F. solani, Fvert: F. verticillium, Foxy: F. oxysporum, Rsol: R. solani, ¶ Means with different letter are statistically different (Duncan; p≤0.05).
Trichoderma asperellum (Tri-5) had a higher inhibition effect over all pathogens (p≤0.0001), with an 85.39 % average; the remaining Trichoderma strains showed no significant difference and the PRGI interval for phytopathogens varied between 72.37 and 78.02 % (Figure 5, Table 2). The pathogen with the highest PRGI in relation to the seven Trichoderma strains was F. oxysporum (Foxy-23) with an 87.5 % average; the pathogen less inhibited by Trichoderma strains was F. solani (Fsol-1) with 72.37 % (Table 2).
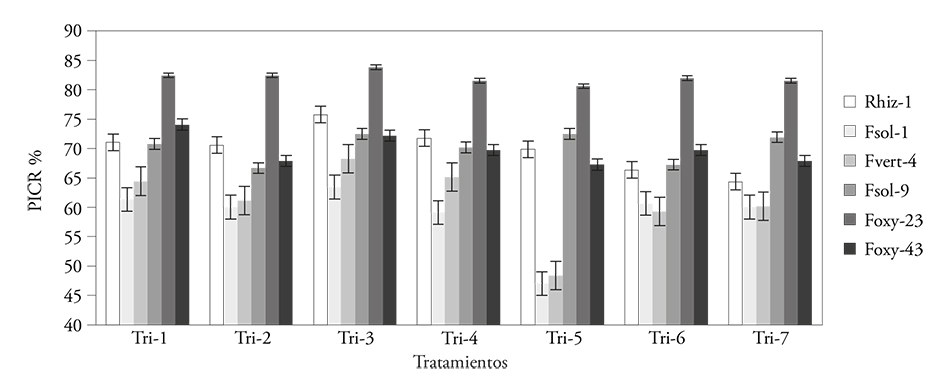
Figure 5 Percentage of radial growth inhibition (PRGI %) in Fusarium spp. and Rhizoctonia solani by Trichoderma spp., in a matched culture test.
Given that the seven strains in the present study inhibited more than 70 % of Fusarium spp. and R. solani’s mycelium growth, hydrolytic enzymes are likely to participate in the inhibitory reaction ( Yedidia et al., 2000; dos Reis-Almeida et al., 2007). Therefore, we can propose these strains as potential inhibitors of phytopathogenic soil fungi. Other field and greenhouses studies will validate their antagonistic effect in other agriculturally important crops.
In the pre-selection test, the pathogens’ PRGI of antagonists native strains was greater than 70 %; this showed its potential to confront pathogens, as they inhibited radial growth; this potential may be due to native Trichoderma strains competing with pathogens in the same ecological niche (Andrews, 1992).
Confrontation between Trichoderma spp., Fusarium spp., and R. solani
The PRGI of the seven Trichoderma strains on pathogenic fungi was variable and depended on the organisms compared (p≤0.0001). The mean values varied between 69.84 and 82.05 % (Figure 5). Overall, Trichoderma strains inhibited 74.1 % of pathogens (Figure 5). Rsol-1’s PRGI stood out; it was obtained from the action of Trichoderma strains (69.84 %). This may be because Rsol-1’s mycelium growth rate is similar to Trichoderma’s. In all confrontations, both fungi competed for space and nutrients; the contact of the antagonist with the pathogen was remarkable -in those cases where Trichoderma colonized the F. solani and R. solani pathogens. T. asperellum (Tri-5) inhibited the vegetative growth of F. solani (Fsol-1) (Figure 6A); meanwhile, R. solani (Figure 6B) showed hyperparasitism, because it practically invaded all colonies of both pathogens and cast its spores over them. Trichoderma harzianum (Tri-6) also colonized R. solani (Rsol-1) (Figure 6C) and F. solani (Fsol-1) (Figure 6D); however, the level of invasion was lower than in T. asperellum, and it only inhibited the phytopathogen’s growth.
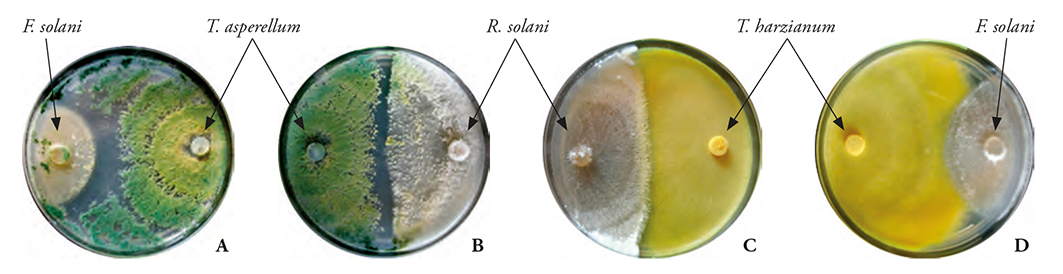
Figure 6 Confrontation between T. asperellum (Tri-5) and T. harzianum (Tri-6) with Fusarium solani (Fsol-1) and R. solani. Where A: T. asperellum on F. solani showed a total mycoparasitism (9 days after inoculation). B: showed almost total mycoparasitism of T. asperellum on R. solani (7 days after inoculation). C: mycoparasitism of T. harzianum on R. solani (7 days after inoculation). D: colonization of T. harzianum on F. solani (10 days after inoculation). Red arrows indicate the limit of Trichoderma spp. mycoparasitism in pathogenic fungi.
When confronted with Fusarium, the results of the seven Trichoderma strains occupied the 1st level of the Bell et al. antagonism scale (1982). Ten days after inoculation, all Trichoderma strains had colonized 100 % of the medium surface, growing over the F. solani, F. oxysporum, and F. verticillioides phytopathogens. This demonstrated the isolations’ high antagonistic capacity. Seven days after inoculation, the antagonism between Trichoderma and R. solani had also reached level 1. Inhibition of the latter exceeded 90 %, with spore masses on the pathogenic fungi, beyond the line between Trichoderma and R. solani (Figure 6B). This indicated that all Trichoderma strains are able to grow fast, even in the presence of highly aggressive pathogens (such as R. solani). This characteristic demonstrated the antagonistic potential and showed that if the surface colonized by the antagonist is great, its domain over the pathogen will be greater as well. It will also give a competitive advantage in the struggle for space, before showing its toxic and mycoparasitic potential in in vitro conditions (Küçük and Kivanç, 2004). Therefore, we are able to state that the antagonism between microorganisms will depend on the antagonist’s biotrophic or necrotrophic action, the extracellular enzymes production, corresponding to the composition and structure of the cell walls of parasitic fungi, and the pathogen’s virulence (Infante et al., 2009).
Trichoderma’s antagonistic activity was not statistically different (mean PRGI: 73.1 to 76.4 %). The coincidence in the range of PRGI means among the seven native Trichoderma strains may be the result of the coadaptation of the antagonists with the pathogenic fungi, since all the microorganisms in this study came from similar weathers and habitats (Andrews, 1992). The high capacity of Trichoderma strains to inhibit mycelium growth allows us to emphasize their efficiency over the fungi that cause root diseases and confirms that the coadaptation of antagonitic and pathogenic fungi is required for this to be accomplished (Baker and Cook, 1974).
The confrontations that involved interaction took place quickly (3 to 4 days after the first contact). Trichoderma strains invaded pathogens’ (Figure 7). These results are similar to those observed by Ezziyyani et al. (2004b), who found out that the T. harzianum-P. capsici intersection zone eventually increased, and this event was accompanied by the destruction of P. capsici’s mycelium. Kexiang et al. (2002) indicated that the intersection zone between the antagonists (T. harzianum and T. atroviride) and the mycelium growth inhibition of the pathogen (Botryosphaeria berengeriana f. sp. piricola) increased over time, and that mycoparasitism suppressed the pathogen’s mycelium growth.
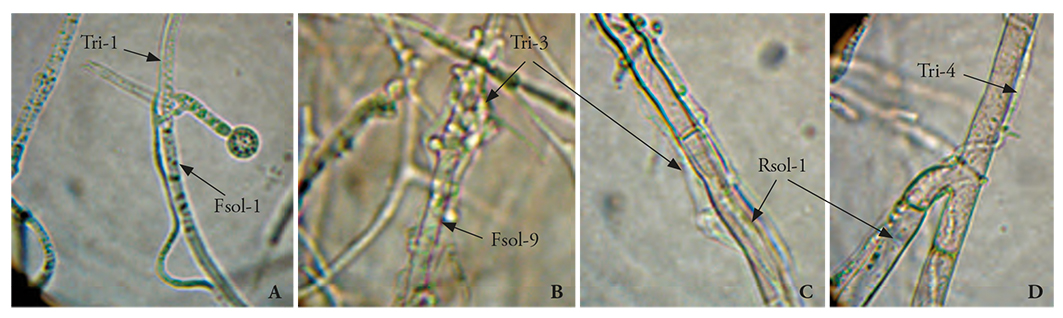
Figure 7 Trichoderma spp. mycoparasitism in dual culture tests. A) Tri-1 vs. Fsol-1 (100X); B) Tri-3 vs. Fsol-9 (100X); C) Tri-3 vs. R. solani (400X); D) Tri-4 vs. R. solani (400X).
The antagonism level is mainly the result of the mycoparasitism mechanism. The results of our study were similar to those of Michel-Aceves et al. (2013), who documented that the inhibition of 6 out of 12 Trichoderma isolates was 10-94.4 % of the Sclerotium rolfsii’s radial growth. Trichoderma’s mycoparasitism over pathogens was remarkable. In general, the Trichoderma seven strains parasitized the five pathogens; however, T. asperellum (Tri-3 and Tri-5) strains showed greater formation of haustoria over the Fusarium spp. and R. solani hyphae. The ways in which Trichoderma spp. parasitized pathogens were observed under a microscope: T. harzianum (Tri-1) hyphae adhered to F. solani (Fsol-1) hyphae and phialide (Figure 7A), they formed haustoria, and penetrated the hypha; meanwhile, T. asperellum (Tri-3) formed large numbers of haustoria in Fsol-9 hyphae, and parasitized and compressed (Figure 7B); T. asperellum hyphae (Tri-3) penetrated, forming haustoria on R. solani hyphae (Figure 7C); T. harzianum (Tri-4) formed haustoria, penetrated, and grew as a parasite within R. solani hyphae (10 to 12 days after the confrontation started) (Figure 7D). Michel-Aceves et al., 2005) mentioned a positive correlation between the number of enzymes and the antagonist’s mycoparasitism percentage, and the disease reduction in affected plants. This coincides with our observations of the T. asperellum and T. harzianum strains: T. asperellum formed more haustoria, which facilitated the entry and mycoparasitism of the pathogen’s hyphae. In the case of T. harzianum, we speculate that there was not enough recognition between Trichoderma and the pathogen, through the interaction of lectins-carbohydrates, which agglutinate the cells that interact with the pathogen’s cell surface and favor mycoparasitism (Infante et al., 2009), therefore, the mycoparasitism level is not the same than with T. asperellum.
Pathogenicity and antagonism results were significant (r=0.41, p=0.05). R. solani showed greater pathogenicity in the plant and it was the pathogen with less in vitro inhibition by Trichoderma strains. The antagonists and pathogens inoculated in bean plants would behave similar to those found in in vitro confrontations. Therefore, these confrontations are a tool that can help us to define the biological control level of Trichoderma species faced with soil pathogenic fungi.
Conclusions
The seven T. harzianum and T. asperellum strains, that were morphologically and molecularly identified, showed different mycoparasitism levels in confrontations with the Fusarium spp. and R. solani bean root pathogens. The T. asperellum Tri-5 strain was remarkable for the greater inhibition of mycelial and radial growth. The average PRGI of the action of all Trichoderma strains over Rsol-1 was lower than the general average; however, due to its antagonistic capacity, T. asperellum was able to invade 90 % of the pathogen. The formation of more haustoria by T. asperellum -which facilitated the entry and mycoparasitism of the pathogen hyphae- confirmed this characteristic.
Literatura Citada
Andrews, J. H. 1992. Biological control in the phyllosphere. Ann. Rev. Phytopathol. 30: 603-635. [ Links ]
Baker, K. F., and J. R Cook. 1974. Biological control of plants pathogens. Firth edition. CRS Press. Freeman, San Francisco, CA. USA. 157 p. [ Links ]
Baker, R., and G. J. Griffin. 1995. Novel approaches to integrated pest management. In: Reuveni, R. (ed). Molecular Strategies for Biological Control of Fungal Plant Pathogens. CRS Press, Boca Raton, Florida. pp: 153-182. [ Links ]
Barnett, H. L., and B. B. Hunter. 1988. Illustrated Genera of Imperfect Fungi. APS Press, St. Paul, Minnesota. 218 p. [ Links ]
Bell, D. K., H. D. Wells, and C. R. Markham. 1982. in vitro antagonism of Trichoderma species against six fungal plant pathogens. Phytopathology 72: 379-382. [ Links ]
Bellemain, E., T. Carlsen, C. Brochmann, E. Coissac, P. Taberlet, and H. Kauserud. 2010. ITS as an environmental DNA barcode for fungi: An in silico approach reveals potential PCR biases. BMC Microbiol. 10: 189. [ Links ]
Bolkan, H. A. 1980. Las pudriciones radicales. In: Schwartz, H. F., y G. E. Gálvez (eds). Problemas de Producción del Frijol. Centro Internacional de Agricultura Tropical. Colombia. pp: 65-99. [ Links ]
Cichy, K. A., S. S. Snapp, and W.W. Kirk. 2007. Fusarium root rot incidence and root system architecture in grafted common bean lines. Plant Soil. 300: 233-244. [ Links ]
Chaverri, P., G. J. Samuels. 2003. Hypocrea/Trichoderma (Ascomycota, Hypocreales, Hypocreaceae): species with green ascospores. Stud. Mycol. 48: 1-116. [ Links ]
Chaverri, P ., L. A. Castlebury, B. E. Overton, and G. J. Samuels. 2003. Hypocrea/Trichoderma: species with conidiophore elongations and green conidia. Mycologia 95: 1100-1140. [ Links ]
Dennis, C., and J. Webster. 1971. Antagonistic properties of species-groups of Trichoderma. Hyphal interactions. Trans. Brit. Mycol. Soc. 57: 363-369. [ Links ]
dos Reis-Almeida, F. B., F. Menezes-Cerqueira, R. do Nascimento-Silva, C. J. Ulhoa, and A. Lopes-Lima. 2007. Mycoparasitism studies of Trichoderma harzianum strains against Rhizoctonia solani: evaluation of coiling and hydrolytic enzyme production. Biotechnol Lett. 29: 1189-1193. [ Links ]
Druzhinina, I. S., A. G. Kopchinskiy, and C. P. Kubicek. 2006. The first 100 Trichoderma species characterized by molecular data. Mycoscience 47: 55-64. [ Links ]
Elad, Y. 2000. Biological control of foliar pathogens by means of Trichoderma harzianum and potential modes of action. Crop Prot. 19: 709-714. [ Links ]
Elad, Y., I. Chet, and Y. Henis. 1982. Degradation of plant pathogenic fungi by Trichoderma harzianum. Can. J. Microbiol. 28: 719-725. [ Links ]
Ezziyyani, M., M. C. Pérez S., A. Sid A., M.A. Requena, y M. E. Candela. 2004a. Trichoderma harzianum como biofungicida para el biocontrol de Phytophthora capsici en plantas de pimiento (Capsicum annuum L.). Anal. Biol. 26: 35-45. [ Links ]
Ezziyyani, M ., S. C. Pérez, M. E. Requena, L. Rubio, y M. E. Candela. 2004b. Biocontrol por Streptomyces rochei Ziyani de la podredumbre del pimiento (Capsicum annuum L.) causada por Phytophthora capsici. Annal. Biol. 26: 69-78. [ Links ]
González, I., D. Infante, B. Martínez, Y. Arias, N. González, I . Miranda, y P. Peteira. 2012. Inducción de quitinasas y glucanasas en cepas de Trichoderma spp. promisorias como agentes para el control biológico. Biotecnol. Appl. 29: 7-11. [ Links ]
Guigón-López, C. et al. 2010. Identificación molecular de cepas nativas de Trichoderma spp. su tasa de crecimiento in vitro y antagonismo contra hongos fitopatógenos. Rev. Mex. Fitopatol. 28: 87-96. [ Links ]
Harman, G. E. 2004. Mythos and dogmas of biocontrol. Changes in perceptions derive from research on Trichoderma harzianum T22. Plant Dis. 84: 377-393. [ Links ]
Hermosa, M. R. et al. 2000. Molecular characterization and identification of biocontrol isolates of Trichoderma spp. Appl. Environ. Microbiol. 66: 1890-1898. [ Links ]
Howell, C. R. 2003. Mechanisms employed by Trichoderma species in the biological control of plant diseases: The history and evolution of current concepts. Plant Dis . 87: 4-10. [ Links ]
Infante, D., B. Martínez, N. González, y Y. Reyes. 2009. Mecanismos de acción de Trichoderma frente a hongos fitopatógenos. Rev. Protec. Veg. 24: 14-21. [ Links ]
Kexiang, G., L. Xiaoguang, L. Yonghong, Z. Tianbo, and W. Shuliang. 2002. Potential of Trichoderma harzianum and T. atroviride to control Botryosphaeria berengeriana f. sp. piricola, the cause of apple ring rot. J. Phytopathol. 150: 271-276. [ Links ]
Kumar, S., G. Stecher, and K. Tamura. 2016. MEGA7: Molecular Evolutionary Genetics Analysis version 7.0 for bigger datasets. Mol. Biol. Evol. Publicado en línea. doi: 10.1093/molbev/msw054 [ Links ]
Küçük, Ç., and M. Kivanç. 2004. in vitro antifungal activity of strains of Trichoderma harzianum. Turk J. Biol. 28: 111-115. [ Links ]
Kullnig, C. M. et al. 2001. Confusion abounds over identities of Trichoderma biocontrol isolates. Mycol. Res. 105: 769-772. [ Links ]
Lieckfeldt, E., G. J. Samuels, H. I. Nirenberg, and O. Petrini. 1999. A morphological and molecular perspective of Trichoderma viride: is it one or two species? Appl. Environ. Microbiol. 65: 2418-2428. [ Links ]
Martin, K. J., and T. Rygiewicz. 2005. Fungal-specific PCR primers developed for analysis of the ITS region of environmental DNA extracts. BMC microbiology. p. 28. [ Links ]
Michel-Aceves, A. C. et al. 2013. Eficiencia biológica de cepas nativas de Trichoderma spp., en el control de Sclerotium rolfsii Sacc., en cacahuate. Avanc. Investig. Agrop. 17: 89-107. [ Links ]
Michel-Aceves, A. C., M. A. Otero S., O. Rebolledo D., R. Lezama G., R. Ariza F., y A. Barrios A. 2005. Producción y efecto antagónico de quitinasas y glucanasas por Trichoderma spp., en la inhibición de Fusarium subglutinans y Fusarium oxysporum in vitro. Rev. Chapingo Serie Hortic. 11: 273-278. [ Links ]
Mitra, A., and V. C. Lele. 1981. Morphological and nutritional studies on mango malformation fungus, Fusarium moniliforme var. subglutinans. Indian Phytopathol. 34: 475-483. [ Links ]
Mora, F. 1996. Combate biológico de Rhizoctonia solani mediante el empleo de Rhizobium leguminosarum biovar phaseoli en el campo. Agron. Mesoam. 7: 23-30. [ Links ]
Morgulis, A. et al. 2008. Database indexing for production MegaBLAST searches. Bioinformatics 15: 1757-1764. [ Links ]
Nashwa, M. A., A. M. Sallam, K. Albo-Elyousr, and A. E. Hassan. 2008. Evaluation of Trichoderma species as biocontrol agents for Damping-Off and wilt diseases of Phaseolus vulgaris L. and efficacy of suggested formula. Egyp. J. Phytopatol. 36: 81-93. [ Links ]
Nelson, P. E., T. A. Toussoun, and F. O. Marasas. 1983. Fusarium species: An Illustrated Manual for Identification. The Pennsylvania State University, University Park. Pennsylvania, USA. 193 p. [ Links ]
Nirenberg, H. I. 1976. Untersuchungenüber die morphologische und biologische differenzierungin der Fusarium-sektion Liseola. Mitt. Biol. Bundesanstalt. Land-Forst. Berlin-Dahlem 169: 1-117. [ Links ]
Samuels, G. J., A. Ismaiel, M. C. Bon, S. De Respinis, and O. Petrini. 2010. Trichoderma asperellum sensu lato consists of two cryptic species. Mycol. 102: 944-966. [ Links ]
Schneider, K. A., and J. D. Kelly. 2000. A greenhouse screening protocol for Fusarium root rot in bean (Phaseolus vulgaris L.). HortSci. 35: 1095-1098. [ Links ]
Schuster, A., and M. Schmoll. 2010. Biology and biotechnology of Trichoderma. Appl. Microbiol. Biotechnol. 87: 787-799. [ Links ]
Sosa, D., M. S. Pérez, S. Molina, J. Demey, K. Gómez, D. Domínguez, M. Istúriz, R. Rumbos, y D. Parra. 2014. Diversidad genética de Trichoderma spp. en Venezuela, determinada mediante análisis combinado ITS-ALFP. Rev. Protec. Veg . 29: 42-51. [ Links ]
Watanabe, S., K. Kumakura, H. Kato, H. Iyozumi, M. Togawa, and K. Nagayama. 2005. Identification of Trichoderma SKT-1, a biological control agent against seed borne pathogens of rice. J. Gen. Plant Pathol. 71: 351-356. [ Links ]
White, T. J., T. Bruns, S. Lee, and J. W. Taylor. 1990. Amplification and direct sequencing of fungal ribosomal RNA genes for phylogenetics. In: Innis, M. A., D. H. Gelfand, J. J. Sninsky, and T. J. White. (eds). PCR Protocols: A Guide to Methods and Applications. Academic Press, Inc., New York. pp: 315-322. [ Links ]
Worasatit, N., K. Sivasithamparam, E. L. Ghisalberti, and C. Rowland. 1994. Variation in pyrone production, lytic enzymes and control of Rhizoctonia root rot of wheat among single-spore isolates of Trichoderma koningii. Mycol . Res . 98: 1357-1363. [ Links ]
Yedidia, I., N. Benhamou, Y. Kapulnik, and I. Chet. 2000. Induction and accumulation of PR proteins activity during early stages of root colonization by the mycoparasite Trichoderma harzianum strain T-203. Plant Physiol. Biochem. 38: 863-873. [ Links ]
Received: January 2016; Accepted: December 2016