Servicios Personalizados
Revista
Articulo
Indicadores
-
Citado por SciELO
-
Accesos
Links relacionados
-
Similares en SciELO
Compartir
Agrociencia
versión On-line ISSN 2521-9766versión impresa ISSN 1405-3195
Agrociencia vol.50 no.8 Texcoco nov./dic. 2016
Plant protection
Tolerance of strawberry (Fragaria × ananassa Duch.) plants pre-mycorrhized with Rhizophagus intraradices and inoculated with PGPR’s to Phytophthora capsici
1 Programa de Fitopatología. Colegio de Postgraduados, Campus Montecillo. 56230. Km. 36.5 carretera Federal México-Texcoco, Montecillo, Texcoco, Estado de México, México. (serret1702@hotmail.com).
2 Programa de Edafología. Colegio de Postgraduados, Campus Montecillo. 56230. Km. 36.5 carretera Federal México-Texcoco, Montecillo, Texcoco, Estado de México, México. (despinos@colpos.mx).
The tolerance to wilting caused by the oomycete Phytophthora capsici in strawberry (Fragaria×ananassa Duch.) var. Festival was evaluated by premycorrhization with the fungus Rhizophagus intraradices and inoculation with a consortium of PGPR’s (Plant Growth Promoting Rhizobacteria) composed of Pseudomonas tolassi, Bacillus pumilus, and Paenibacillus sp. The inoculation of biocontrolling microorganisms was carried out separately and in combination 8 d after transplanting. The inoculation of P. capsici was carried out 45 d after applying biocontrollers. The experimental design was completely randomized, data were analyzed by ANOVA and the averages of treatments were compared using Tukey’s test (p≤0.05). In a previous antagonism in vitro experiment, P. tolassi and B. pumillus were found to inhibit oomycete growth by 54 %, while Paenibacillus sp. did the same by 43 %. The production of metabolites by rhizobacteria that disseminate and inhibit the growth of the oomycete is suggested. The severity of the disease in plants treated with P. capsici was 63 %, but it was lower (23 %) in plants inoculated with PGPR’s+R. intraradices. Plants inoculated with PGPR’s+R. intraradices showed the highest values for foliar area (239.95 cm2), dry biomass of aerial section (6.24 g), root volume (2.1 mL) and number of stolons (5). Plants previously treated with PGPR’s and R. intraradices and then inoculated with P. capsici, increased foliar area, aerial biomass, and root volume by 44, 7, and 58 %, respectively, in comparison to plants inoculated only with the oocmycete. According to the analysis of results, bio-controlling microorganisms promote the tolerance of strawberry plants to wilting induced by P. capsici.
Key words: Phytophthora capsici; Fragaria×ananassa Duch.; Rhizophagus intraradices; Pseudomonas tolassi; Bacillus pumilus; Paenibacillus sp.; biocontrol; PGPR’s
La tolerancia a la marchitez causada por el oomiceto Phytophthora capsici en fresa (Fragaria×ananassa Duch.) variedad Festival, se evaluó mediante premicorrización con el hongo Rhizophagus intraradices e inoculación con un consorcio de rizobacterias promotoras del crecimiento vegetal o PGPR’s (Plant Growth Promoting Rhizobacteria) conformado por Pseudomonas tolassi, Bacillus pumilus, y Paenibacillus sp. La inoculación de los microorganismos biocontroladores se hizo por separado y en combinación 8 d después del trasplante. La inoculación de P. capsici se hizo 45 d después de aplicar los biocontroladores. El diseño experimental fue completamente al azar, los datos se analizaron con ANDEVA y las medias de los tratamientos se compararon con la prueba de Tukey (p≤0.05). En un experimento de antagonismo in vitro previo, P. tolassi y B. pumillus inhibieron el crecimiento del oomiceto en 54 %, y Paenibacillus sp. lo hizo en 43 %. Se sugiere la producción de metabolitos por las rizobacterias que difunden e inhiben el crecimiento del oomiceto. La severidad de la enfermedad en las plantas tratadas con P. capsici fue 63 %, pero fue menor (23 %) en las plantas inoculadas con PGPR’s+R. intraradices. Las plantas inoculadas con PGPR’s+R. intraradices mostraron los valores más altos de área foliar (239.95 cm2), biomasa de aérea seca (6.24 g), volumen radical (2.1 mL) y número de estolones (5). Las plantas tratadas previamente con PGPR’s y R. intraradices e inoculadas después con P. capsici, aumentaron en 44, 7, y 58 % el área foliar, la biomasa aérea y el volumen radical, respectivamente, en comparación con las plantas inoculadas solo con el oomiceto. De acuerdo con el análisis de los resultados, los microorganismos biocontroladores favorecen la tolerancia de las plantas de fresa a la marchitez inducida por P. capsici.
Palabras clave: Phytophthora capsici; Fragaria×ananassa Duch.; Rhizophagus intraradices; Pseudomonas tolassi; Bacillus pumilus; y Paenibacillus sp.; biocontrol; PGPR’s
Introduction
The strawberry plant (Fragaria X ananassa Duch.) belongs to the Rosaceae family and stands out for its content of vitamin C, tannins, flavonoids, anthocyanins, catechins, quercetin, kaempferol, and organic acids (Kessel, 2012). Strawberry plantations are socioeconomically important for Mexico, due to its large workforce demand and because it provides a considerable portion of incomes produced by fruit exports (Sánchez, 2008).
The main strawberry-producing states in Mexico are Michoacán with 5896 ha and Baja California with 2273.30 ha planted (SIAP, 2014). In the area of Zamora, Michoacán, the most widely-planted varieties are Festival, with 32 % of the total surface, Camino Real with 28 % and Aromas with 20 %. In northern-central Mexico, the varieties Camino Real, Camarosa and Festival cover 97 % of the total surface (Sánchez, 2008).
The genus Phytophthora is an oomycete and is among the main agents that cause diseases; it is directly responsible for high economic losses in strawberry plantations. High relative humidity, abundant soil humidity and temperatures between 25 and 30 °C increase the severity of Phytophthora capsici (Granke et al., 2012; Sanogo and Bosland, 2013; Callaghan et al., 2016). Host plants infected with Phytophthora spp. strong wilting, chlorosis and cracking of the stem, making them weak, vulnerable to other pathogens, and leading to their death (Dorantes et al., 2008).
Exudates from strawberry plant roots colonized by Arbuscular Mycorrhizal Fungi (AMF) reduce the sporulation of Phytophthora fragariae (Norman and Hooker, 2000). Mycorrhized strawberry plants are tolerant to wilting caused by Fusarium oxysporum, although little is known on the mechanisms of tolerance to the disease in mycorrhized plants (Matsubara et al., 2012). Arbuscular mycorrhiza and the Plant Growth Promoting Rhizobacteria (PGPR’s) have a protective effect against diseases caused by some root pathogens such as Phytophthora capsici, Erwinia carotovora and Pseudomonas syringae (Dorantes et al., 2008).
The aim of this study was to evaluate premycorrhization with Rhizophagus intraradices and inoculation with the rhizobacteria Pseudomonas tolassi, Bacillus pumilus, and Paenibacillus sp. in the suppression of wilting caused by P. capsici in strawberry plants. Given that inoculation of the bio-controlling microorganisms separately reduces the incidence of the oomycete, we hypothesized that joint inoculation will increase its effectiveness by reducing the incidence and severity of wilting in strawberry plants infested with P. capsici.
Materials and Methods
This investigation was carried out in two phases: The first was in the laboratory, and the second, in a controlled environment chamber (Sherer® Modelo CEL 37-14, Gillet Marshall Mich. USA).
Biological material
Festival variety strawberry plants were acquired in the company named Insumos Agrícolas para Invernadero INAPI, in Irapuato, Guanajuato.
The bacterial strains (Pseudomonas tolassi, B. pumilus and Paenibacillus sp.) and the Arbuscular Mycorrhizal Fungus (R. intraradices) were provided by the Soil Microbiology Area, from the Soil Science Graduate Program, Colegio de Postgraduados, Campus Montecillo.
Phytophthora capsici strain PcT17 used in this experiment was provided by the Universidad Michoacana in San Nicolás de Hidalgo, Mexico.
Pathogenicity of Phytophthora capsici strain PcT17 on strawberry
Eight Festival variety strawberry plants with three and four leaves were inoculated (8 d after transplant) with 200 000 zoospores per plant, to show the pathogenicity of the oomycete in this variety of strawberry under greenhouse conditions.
First phase: Antagonistic capacity in vitro of three strains of de rhizobacteria towards Phytophthora capsici
The first phase was a test for antagonism by confrontation in vitro of the rhizobacteria of P. tolassi, B. pumilus, and Paenibacillus sp. against P. capsici. The rhizobacteria were cultivated in nutritive agar for 48 h at 27 °C and the strain P. capsici was developed in V8 medium V8 (juice of eight vegetables: calcium carbonate: agar) at 27 °C for 10 d.
Test in vitro
The mycelial growth of P. capsici in vitro was evaluated using the dual cultivation technique described by Landa et al. (1997). V8 medium discs, 5 mm in diameter, with the active growth of the oomycete, were placed in the center of a Petri dish with V8 medium. At the same time, the bacteria were planted individually in a straight line, 3 cm away from the disc with mycelial content. The cultures were incubated for 5 d at 28 °C. The control was a 5 mm disc with V8 medium with mycelial growth, but without bacterial inoculant.
Treatments and experimental design
The experiment had four treatments, 1) P. toolassi confronted with P. capsici, 2) B. pumilus confronted with P. capsici, 3) Paenibacillus sp. confronted with P. capsici and 4) Control. The experimental design was totally at random and each treatment had four repetitions. The experimental unit was one Petri dish.
The percentage of inhibition was calculated using the following formula (Landa et al., 1997):
where r is the radius of P. capsici and R is the maximum radius of the oomycete without the bacteria.
Second phase: Test under controlled environment conditions
Uniformly sized Festival variety strawberry plants were disinfected with sodium hypochlorite at 1 % for 3 min and rinsed three times with sterile distilled water. Each plant was moved to a 400 cm3 container, which was the experimental unit.
The substrate was a mixture of peat moss, agrolite and vermicompost (4:4:2 v/v/v), sterilized in an autoclave at 120 °C for 2 h, twice. The plants were watered with sterile water as required and each week a Long Asthon nutritive solution was added, modified to apply 11 mg of P L-1 (Hewitt, 1966).
The strawberry plants were inoculated 8 d after transplanting with 0.5 g of sorghum roots colonized in 80 to 85 % by R. intraradices, and 1 mL of the bacterial suspension composed of P. toolassi, B. pumilus and Paenibacillus sp. at a concentration of 108 UFC mL-1. Strawberry plants with and without inoculant were placed in a controlled environment chamber (Sherer® Model CEL 37-14, Gillet Marshall Mich. USA), at a constant temperature of 26 °C.
Multiplication and inoculation of Phytophthora capsici
P. capsici cultures with 10 d of growth in V8 medium were added 10 mL of sodium cloride solution at 0.9 % (AbbottMR) per Petri dish for 10 min. The isotonic solution was poured, divided into four parts, and each was placed in a sterile Petri dish. Later, sterile distilled water was added and the P. capsici cultures were placed under cold white light at 26 °C for 48 h, and at 28 °C in the dark for 24 h.
The Petri dishes with P. capsici were placed for 30 min at 4 °C, then for 30 min at 28 °C to promote the release of zoospores. The content of each Petri dish was emptied into a container and the number of zoospores was counted using a cytometer (Marienfeld®). The zoospore suspension was adjusted to 200 000 zoospores mL-1.
The oomycete was inoculated in strawberry plants 45 d after the inoculation of bio-controlling microorganisms, adding 1 mL of the suspension in each container. Inoculated plants were kept for 5 d in moisture-saturated soil to promote the displacement of the oomycete. This experiment was performed twice.
Disease incidence and severity
The incidence (total number of plants wilted by the pathogen in regard to the total expressed as a percentage) and severity of the disease was registered on days 5, 10, 15 and 20 after the pathogen inoculation. Severity was evaluated using the modified scale by Adorada et al., (2000), that assigns values from 1 to 50 according to the degree of plant wilting: 1=0 % wilted plants; 2=1-25 % of wilted plants; 3=26-50 % of wilted plants; 4=51-75 % of wilted plants; 5=76-100 % of wilted plants or death.
The disease severity (DS) was expressed as a proportion (percentage) according to the formula by Adorada et al., (2000):
Foliar area, dry biomass weight of the aerial part, radical volume, and number of stolons
The foliar area was registered directly using a foliar area meter (Area Meter, Model LI-3100; Nebraska, U.S.A.). The weight of the dry biomass of the aerial part was determined by drying the samples in an oven (FELISA, Model 242-A) at 70 °C for 72 h y and then weighed using an analytical scale (Acculab, Model ALC-104). The volume of the root was evaluated by water displacement, submerging the root system in a graduated 100 mL test tube. The number of stolons was determined in each treatment. All variables were evaluated at the end of the experiment.
Determination of nitrogen (N) and phosphorous (P)
N and P were determined in the Soil Fertility lab of the Colegio de Postgraduados. Nitrogen, using the semi-microkjeldahl method (Bremner, 1975) and phosphorous, using photocolorimetry by reduction with Molibdo-vanadate.
Determination of mycorrhizal colonization
Mycorrhizal colonization was determined using the root staining and clearing method (Phillips and Hayman, 1970).
The frequency of the mycorrhizal colonization in strawberry plant roots was determined using the method by Biermann and Linderman (1981) and was expressed as a percentage.
Experimental design and treatments
The experimental design was totally at random, with eight treatments and six repetitions each. The treatments were: 1) Negative control (not inoculated), 2) Positive control (inoculated only with P. capsici), 3) Plants inoculated with PGPR’s (Pseudomonas tolassi, Bacillus pumilus, and Paenibacillus sp.), 4) Plants inoculated with PGPR’s+P. capsici, 5) Plants inoculated with R. intraradices, 6) Plants inoculated with R. intraradices+P. capsici, 7) Plants inoculated with PGPR’s+R. intraradices and 8) Plants inoculated with PGPR’s+R. intraradices+P. capsici. The experimental unit was a 400 cm3 pot with one plant.
An ANOVA was performed using the data, the means of the treatments were compared using Tukey’s test (p>0.05), and SAS Version 9.0 (SAS Institute, 2002) was used.
Results and Discussion
First phase: Antagonistic capacity in vitro of P. tolassi, B. pumillus and Paenibacillus sp. towards Phytophthora capsici
The three bacterial strains had an antagonistic effect against P. capsici (Table 1 and Figure 1). P. tolassi and B. pumillus inhibited the growth of the oomycete by 54 %, and Paenibacillus sp. by 43 %. These results suggest that the rhizobacteria probably produce secondary metabolites that spread throughout the agar and inhibit the growth of the phytopathogen.
Table 1 InvitroinhibitionofmycelialgrowthofPhytophthora capsici caused by three strains of rhizobacteria, after five days of co-cultivation.
Cepa rizobacteriana | Inhibición del oomiceto (%) |
Pseudomonas tolassi | 54.93±0.09 a |
Bacillus pumilus | 54.12±0.89 a |
Paenibacillus sp. | 43.11±0.05 a |
Testigo | 0 b |
a, b Averages with different letters in a column are statistically different (p≤0.05). ± standard deviation; n: 4 repetitions per treatment.

Figure 1 Inhibition of Phytophthora capsici by rhizobacteria. A) P. capsici growing alone (Control), (B) P. capsici with Bacillus pumilus (C) P. capsici with Pseudomonas tolassi and (D) P. capsici with Paenibacillus sp.
Landa et al. (1997) report that some strains of Pseudomonas produce secondary metabolites that spread in agar, causing the inhibition of Fusarium spp. Also, some Bacillus species exert an antagonistic effect due to the production of lytic enzymes, antibiotics or metabolites, or both, which produce changes in the cytoplasmic membrane (Sicuia et al., 2015; Ramyabharathi and Raguchander, 2014).
Second phase: Test in controlled environment
Disease incidence and severity
On days 0 and 3 no incidence of the disease was observed. Plants inoculated only with the pathogen showed 83 % incidence of the plant at the end of the experiment. However, plants pre-inoculated with PGPR’s or R. intraradices showed incidences of 50 and 33 %, respectively. The manifestation of the disease in plants inoculated with PGPR’s+R. intraradices was 33 % (Figure 2).

Figure 2 Disease incidence caused by Phytophthora capsici in strawberry plants pre-inoculated with Rhizophagus intraradices and PGPR’s. The incidence was registered on days 5, 10, 15, and 20 after inoculationg with the pathogenic oomycete. Each point corresponds to the average of six plants.
The severity of the disease began manifesting itself in plants not inoculated with beneficial microorganisms, five days after the inoculation of the pathogen. At the end of the experiment, the severity of the disease in positive control plants was 63 %, yet plants pre-inoculated with PGPR’s showed a severity of 37 % (Figure 3), due possibly to the induction of systemic resistance (Nadeem et al., 2014), or when competing for nutrients and niche (Ochoa et al., 2010).

Figure 3 Disease severity caused by Phythophthora capsici in strawberry plants pre-inoculated with Rhizophagus intraradices and PGPR’s. The severity was registered on days 5, 10, 15, and 20 after inoculation with the pathogenic oomycete. Each point corresponds to the average of six plants.
Plants inoculated with R. intraradices displayed a severity of 33 %. Alarcón et al. (2000) indicate that apart from the nutritional benefit of the symbiosis, AMFs also participated as root pathogens biological control agents.
Plants inoculated with PGPR’s+R. intraradices presented a lower severity (23 %) (Figure 3). AMFs and rhizobacteria act syngergically, stimulating plant growth and the inhibition of the pathogenic oomycete (Figure 4). PGPR’s stimulate AMF growth (Linderman, 1993), root development, a greater susceptibility of the root to the colonization of mycorrhizal fungi, and improves the process of recognition between roots and fungi (Artursson et al., 2006; Bonfante and Anca, 2009).
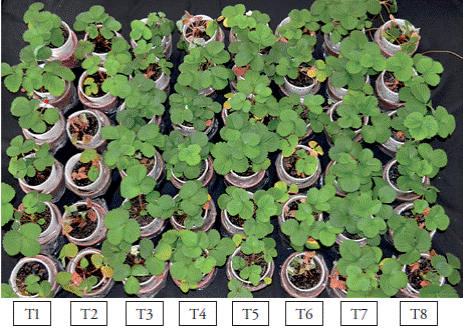
Figure 4.Response of the Festival commercial variety strawberry premycorrhized with Rhizophagus intraradices and inoculated with PGPR’s to the attack of Phytophthora capsici. Non-inoculated plants (T1), plants inoculated with P. capsici (T2), plants inoculated with only PGPR’s (T3), plants inoculated with PGPR’s+ P. capsici (T4), plants inoculated with only R. intraradices (T5), plants inoculated with R. intraradices + P. capsici (T6), plants inoculated with R. intraradices +PGPR’s (T7) and plants inoculated with R. intraradices, PGPR’s+ P. capsici (T8).
PGPR’s suppress diseases caused by pathogenic microorganisms with the production of siderophores, synthesis of antibiotics, enzymes, or fungicidal compounds or both (Lugtenberg and Kamilova, 2009). For example, Pseudomonas and Bacillus can control pathogens, particularly fungi, synthesizing antifungal molecules (Whipps, 2001). Bacteria of the genus Pseudomonas induce plant resistance by increasing the speed and levels of synthesis of phytoalexins, substances directly implied in the plant’s defense (Lemanceau and Alabouvette, 1993).
Foliar area, dry biomass weight of the aerial part, radical volume, and number of stolons
Plans inoculated with PGPR’s+R. intraradices showed greater foliar areas (239.95 cm2), dry biomass weight (6.24 g) and root volume (2.1 mL) (Table 2). These results coincide with reports by Vosatka et al. (2000) and Linderman (1993), who state that the use of joint mutualistic symbionts (nitrogen fixers in free life such as Pseudomonas and Bacillus) affects the increase in plant growth and production. Also, the symbiotic-mutualistic relationship in the roots of fruit-producing plants leads to diverse physiological changes or modifications and highlights the increase in photosynthetic activity due to the greater CO2 fixation capacity (Alarcón and Ferrera-Cerrato, 2000); therefore, the rates of dry biomass produced increase (Alarcón et al., 2000). According to Díaz et al. (2013), the symbiosis with AMF provides benefits in the host plant, such as increase in growth and mineral nutrition.
Table 2 Growth variables in pre-mycorrhized strawberry plants inoculated with PGPR’s (Pseudomonas tolassi, Bacillus pumilus and Paenibacillus sp.) in response to the attack of Phytophthora capsici.
Tratamiento | Área foliar (cm2) | Materia seca (g) | Volumen radical (mL) |
Testigo negativo (sin inocular) | 170.74±30.92 ab | 5.80±0.26 ab | 0.90±0.12 bc |
Testigo positivo (inoculado con P. capsici) | 93.77±5.64 b | 5.24±0.28 b | 0.33±0.08 c |
Plantas inoculadas con PGPR’s | 174.92±19.24 ab | 5.56±0.13 ab | 0.90±0.12 bc |
Plantas inoculadas con PGPR’s y P. capsici | 189.28±27.26 ab | 5.97±0.17 ab | 0.90±0.15 bc |
Plantas inoculadas con R. intraradices | 189.72±10.36 ab | 5.54±0.20 b | 1.36±0.17 b |
Plantas inoculadas con R. intraradices y P. capsici | 128.36±17.97 b | 5.54±0.21 ab | 0.80±0.10 bc |
Plantas inoculadas con PGPR’s y R. intraradices | 239.95±24.29 a | 6.24±0.19 a | 2.10±0.31 a |
Plantas inoculadas con PGPR’s, R. intraradices y P. capsici | 167.43±27.85 ab | 5.60±0.22 ab | 0.80±0.10 bc |
Averages with different letters in a column are statistically different (p≤0.05). ± standard deviation; n: 6 repetitions per treatment.
Plant growth and nutrition (Table 2) was improved by the rhizobacterial production of growth regulators, as reported by Molina-Romero et al. (2015). Plants inoculated with PGPR’s+R. intraradices produced five stolons, plants inoculated with R. intraradices produced three, and plants inoculated with PGPR’s, only one. This result may be due to the synthesis of growth regulators such as gibberellins and cytokinins (Alarcón et al., 2000). In the other treatments there was no stolon production.
Nitrogen (N) and phosphorous (P) contents
The concentration of N and P in the foliage was not different (p>0.05) between treatments (Table 3), although PGPR’s promote plant growth by directly providing N, P, and essential minerals (Ahemad and Kibret, 2014). PGPR’s can fix atmospheric nitrogen due to the presence of the enzyme nitrogenase, making this N accessible to plants with which they associate (Gupta et al., 2015). The three species of PGPR’s used in our study (B. pumilus, P. tolassi, and Paenibacillus sp.) are reported as non-symbiotic N-fixating bacteria (Hernández et al., 2009; Glick et al., 2007; Fernandes et al., 2014). There were no significant differences in the concentration of N in the foliage and the highest value (1400 mg kg-1) was observed in plants inoculated only with PGPR’s. The non-symbiotic, N-fixating PGPR’s only provide small amounts of the N fixated onto the host plant (Glick, 2012). Also, the low contribution of N by bacteria is affected by environmental conditions, such as high concentrations of O2 in the soil atmosphere, which inhibit nitrogenase (Alquéres et al., 2010), or high concentrations of H2 and NO that compete with the substrate of nitrogenase (Hichri et al., 2015; Gabrielyan et al., 2015). The inoculation of HMA and PGPR’s did not increase (p>0.05) the P in the foliage due to its high reactivity with Fe, Al, and Ca and to its resulting precipitation. The ranges of precipitation may be up to 90 % (Adesemoye et al., 2008); likewise, the soil and the environmental conditions impact the efficiency of PGPR’s and HMAs (Adesemoye et al., 2008).
Table 3 Concentration of N and P in strawberry plants, pre-mycorrhized and inoculated with PGPR’s in response to the attack of Phytophthora capsici.
Tratamiento | Nitrgeno (N) mg kg-1 | Fósforo (P) mg kg-1 |
Testigo negativo (sin inocular) | 1.18±0.09 | 0.73±0.10 |
Testigo positivo (inoculado con P. capsici) | 0.97±0.11 | 0.62±0.18 |
Plantas inoculadas con PGPR’s | 1.40±0.12 | 0.63±0.15 |
Plantas inoculadas con PGPR’s y P. capsici | 0.83±0.15 | 0.43±0.06 |
Plantas inoculadas con Rhizophagus intraradices | 0.93±0.11 | 0.68±0.05 |
Plantas inoculadas con R. intraradices y P. capsici | 1.38±0.17 | 0.63±0.08 |
Plantas inoculadas con PGPR’s y R. intraradices | 0.90±0.10 | 0.58±0.11 |
Plantas inoculadas con PGPR’s, R. intraradices y P. capsici | 1.37±0.15 | 0.64±0.09 |
There are no statistical differences between treatments (p>0.05). ± standard deviation; n: 4 repetitions per treatment.
The highest growth rate was registered in plants inoculated with HMA+PGPR’s. Genera Pseudomonas, Azotobacter, and Bacillus release indole3-acetic acid (AIA), gibberellins or cytokinins in the rhizosphere of plants and they exert a growthstimulating effect, particularly in the plantlet stage (Lugtenberg and Kamilova, 2009). Also, some PGPR’s produce enzymes that favor plant growth and development with the reduction of ethylene. Such bacteria take ACC, the precursor of ethyene, and turn it into 2 oxobutanoate and NH3 (Glick et al., 2007).
Mycorrhizal colonization
For total mycorrhizal colonization there was no statistical differences between treatments. The highest numerical value (70 %) was registered in the treatment of plants premycorrhized with R. intraradices and inoculated with PGPR’s, and the lowest (39.75 %), in plants inoculated with R. intraradices+P. capsici (Table 4). Frey-Klett et al. (2007) report the involvement of different genera of soil PGPR’s in the mychorrhizal colonization process; this group of PGPR’s is known as mycorrhization helper bacteria. The highest percentages of total colonization, vesicles and hyphae in our experiment were obtained when PGPR’s were inoculated (Table 4). Probably, these bacteria promoted mycorrhizal colonization, but not sporulation in strawberry plants.
Table 4 Percentage of mycorrhizal colonization in strawberry plants inoculated with Rhizophagus intraradices, PGPR’s and Phytophthora capsici in a controlled environment growth chamber.
Tratamiento | Colonizción micorrízica total (%) | Vesíclas (%) | Hifas (%) | Esporas (%) |
Plantas inoculadas con R. intraradices | 59.00±2.50 | 20.00±2.82 | 19.00±2.00 | 20.00±3.96 |
Plantas inoculadas con R. intraradices y P. capsici | 39.75±1.65 | 24.75±4.52 | 13.00±2.39 | 2.00±1.58 |
Plantas inoculadas con PGPR’s y R. intraradices | 70.00±0.66 | 43.00±2.30 | 19.00±1.30 | 8.00±0.84 |
Plantas inoculadas con PGPR’s, R. intraradices y P. capsici | 55.00±1.02 | 43.00±1.95 | 8.00±1.87 | 4.00±0.55 |
There are no statistical differences between treatments (p>0.05). ± standard deviation; n: 5 repetitions per treatment.
Vesicles were the predominant structures and the number was the same in plants inoculated with R. intraradices and plants inoculated with PGPR’s+R. intraradices (Figure 5).
Conclusions
Phytophthora capsici strain PcT17 was pathogenic for Festival commercial variety strawberry plants. The PGPR’s (Pseudomonas tolassi, Bacillus pumilus, and Paenibacillus sp) inhibited the growth in vitro of the oomycetes. Rhizophagus intraradices and the PGPR’s (Pseudomonas tolassi, Bacillus pumilus, and Paenibacillus sp.) reduced the incidence and severity of Phytophthora capsici under controlled conditions.
In aerial plant tissue, the concentrations of N and P did not increase, in contrast to reports of other studies. The greatest growth in plants inoculated with HMA and PGPR’s is probably due to the production of plant growth regulators, which is documented in genera Pseudomonas and Bacillus.
Literatura Citada
Adesemoye, A. O., H. A. Torbert, and J. W. Kloepper. 2008. Henhanced plant nutrient use efficiency with PGPR and AMF in an integrated nutrient management system. Can. J. Microbiol. 54: 876-886. [ Links ]
Adorada, D. L., C. L. Billes, C. M Liddell, S. Pvia-Fernandez, K. O Waugh, and M. E. Waugh. 2000. Susceptibility of wounded pepper roots to Phytophthora capsici. Plant Pathol. 49: 719-726. [ Links ]
Ahemad, M., and M. Kibret, 2014. Mechanisms and applications of plant growth promoting rhizobacteria: Current perspective. J. King Saud University - Sci. 26: 1-20. [ Links ]
Alarcón, A., R. Ferrera-Cerrato, M. C González-Chávez, y A. Villegas-Monter. 2000. Hongos micorrízicos arbusculares en la dinámica de aparición de estolones y nutrición de plantas de fresa cv. fern obtenidas por cultivo in vitro. Terra Latinoam. 18: 4-8. [ Links ]
Alarcón, A., y R. Ferrera-Cerrato. 2000. Manejo de la micorriza arbuscular en sistemas de propagación de plantas frutícolas. Terra Latinoam. 17: 179-191. [ Links ]
Alquéres, S. M. C., J. H. M. Oliveira, E. M. Nogueira, H. V. Guedes, P. L. Oliveira, F. Cámara, J. I. Baldani, and O. B. Martins. 2010. Antioxidant pathways are up-regulated during biological nitrogen fixation to prevent ROS-induced nitrogenase inhibition in Gluconacetobacter diazotrophicus. Arch. Microbiol. 192: 835-841. [ Links ]
Artursson, V., R. D. Finlay, and J. K. Jansson. 2006. Interactions between arbuscular mycorrhizal fungi and bacteria and their potential for stimulating plant growth. Environ. Microbiol. 8: 1-10. [ Links ]
Biermann, B., and R. G. Linderman. 1981. Quantifying vesicular arbuscular mycorrhizae: A proposed method towards standardization. New Phytophatol. 97: 63-67. [ Links ]
Bonfante, P., and I. Anca. 2009. Plants, mycorrhizal fungi, and bacteria: a network of interactions. Annual Rev. Microbiol. 63: 363-383. [ Links ]
Bremner, J. M. 1975. Total nitrogen. In: C. A. Black (ed). Methods of Soil Analysis Part 2. Agronomy 9: 1149-1178. [ Links ]
Callaghan S. E., A. P. Williams, T. Burgess, D. White, T. Keovorlajak, P. Phitsanoukane, S. Phantavong, S. Vilavong, K. B. Ireland, G. S. Duckitt, and L. W. Burgess. 2016. First report of Phytophthora capsici in the Lao PDR. Austr. Plant Dis. Notes 11: 22. [ Links ]
Díaz, F. A., C. M Alvarado, C. F Ortiz, y C. O Grageda. 2013. Nutrición de la planta y calidad del fruto de pimiento asociado con micorriza arbuscular en invernadero. Rev. Mex. Cienc. Agric. 4: 315-321. [ Links ]
Dorantes, G., N. C Abud, y F. P. Pavía. 2008. Reducción de la susceptibilidad a Phytophthora capsici Leonian causante de la pudrición de raíz en jitomate (Solanum lycopersicum L.) Biológicas 10: 100-108. [ Links ]
Fernandes, G. C., L. J. Trarbacha, B. Samanta, S. B. de Campos, A. Beneduzi, and L. M. P. Passaglia. 2014. Alternative nitrogenase and pseudogenes: unique features of the Paenibacillus riograndensis nitrogen fixation system. Res. Microbiol. 165: 571-580. [ Links ]
Frey-Klett, M., J. Garbaye, and M. Takka. 2007. The mycorrhiza helper bacteria revisited. Tansley review. New Phytol. 176: 22-36. [ Links ]
Gabrielyan, L., H. Sargsyan, and A. Trchounian. 2015. Novel properties of photofermentative biohydrogen production by purple bacteria Rhodobacter sphaeroides: effects of protonophores and inhibitors of responsible enzymes. Microb. Cell Fact. 14: 131. [ Links ]
Glick, B. R., 2012. Plant growth-promoting bacteria: Mechanisms and applications. Scientifica 2012: 15 pages. [ Links ]
Glick, B. R., Z. Cheng, J. Czarny, and J. Duan. 2007. Promoting of plant growth by ACC deaminase producing soil bacteria. Plant Pathol. 119: 329-39. [ Links ]
Granke, L. L., M. L. Quesada-Ocampo, y M. K. Hausbeck. 2012. Differences in virulence of Phytophthora capsici isolates from a worldwide collection on host fruits. Eur. J. Plant Pathol. 132: 281-296. [ Links ]
Gupta, G., S. S. Parihar, N. K. Ahirwar, S. K. Snehi, and V. Singh. 2015. Plant Growth Promoting Rhizobacteria (PGPR): Current and future prospects for development of sustainable agriculture. J. Microb. Biochem. Technol. 7: 096-102. [ Links ]
Hernandez, J. P., L. E. de-Bashan, D. J. Rodriguez, Y. Rodriguez, and Y. Bashan. 2009. Growth promotion of the freshwater microalga Chlorella vulgaris by the nitrogen-fixing, plant growth-promoting bacterium Bacillus pumilus from arid zone soils. Eur. J. Soil Biol. 45: 88-93. [ Links ]
Hewitt, E. J. 1966. The composition of the nutrient solution. In: Hewitt, E. J. (ed). Sand and Water Culture Methods Used in the Study of Plant Nutrition. Farnham Royal, England. pp: 187-246. [ Links ]
Hichri, I., A. Boscari, C. Castella, M. Rovere, A. Puppo, and R. Brouquisse. 2015. Nitric oxide: a multifaceted regulator of the nitrogen-fixing simbiosis. J. Exp. Bot. 66: 2877-2887. [ Links ]
Kessel, D. A. 2012. Mejora genética de la fresa (Fragaria ananassa´Duch.) a través de métodos biotecnológicos. Cultivos Trop. 33(3): 34-41. [ Links ]
Landa, B., A. Hervás, W. W. Bettiol, and R. Jiménez-Díaz. 1997. Antagonistic activity of bacteria from the chickpea rhizosphere against Fusarium oxysporum f. sp. Ciceris. Phytoparasitica 25: 305-318. [ Links ]
Lemanceau, P., and C. Alabouvette. 1993. Supression of Fusarium wilts by fluorescent pseudomonads: mechanisms and applications. Biocontrol Sci. Tech. 3: 219-34. [ Links ]
Linderman, R. G. 1993. Effects on microbial interactions in the micorrhizosphere of plant growth and health. In: Ferrera-Cerrato, R., y R. Quintero L. (eds). Agroecología, Sostenibilidad y Educación. Centro de Edafología, Colegio de Postgraduados. Montecillo, Estado de México. pp: 138-152. [ Links ]
Lugtenberg, B., and F. Kamilova. 2009. Plant growth promoting rhizobacteria. Annu. Rev. Microbiol. 63: 541-556. [ Links ]
Matsubara, Y., Y. Li, T. Okada, and M. A. Maya. 2012. Factors on induced systemic disease resistance in mycorrhizal strawberry plants. In: Mezzetti, B., and P. Brás de Oliveira (eds). Proc. XXVIIIth IHCInt. Berry Symp. Acta Hort. 926: 497-502. [ Links ]
Molina-Romero, A., M. R. Bustillos-Cristales, O. Rodríguez Andrade, Y. E. Morales-García, Y. Santiago-Saenz, M. Castañeda-Lucio, y J. Muñoz-Rojas. 2015. Mecanismos de fitoestimulación por rizobacterias, aislamientos en América y potencial biotecnológico. Biológicas 17: 24-34. [ Links ]
Nadeem, S. M, Z. A. Zahir, M. Naveed, and M. Ashraf. 2014. Microbial ACC-deaminase: prospects and applications for inducing salt tolerance in plants. Crit Rev. Plant Sci. 29: 360-93. [ Links ]
Norman, J., and E. Hooker. 2000. Sporulation of Phytophthora fragarie shows greater stimulation by exudates of nonmycorrhizal than by micorrhizal strawberry roots. Mycol Res. 104: 1069-1073. [ Links ]
Ochoa, M. C., R. P. Madrigal, M. T. Martínez, y A. Carreón. 2010. Plantas, hongos micorrízicos y bacterias su compleja red de interacciones. Biológicas 12: 65-71. [ Links ]
Phillips, J. M., and D. S. Hayman. 1970. Improved procedures for clearing roots and staining parasitic and vesicular arbuscular mycorrhizal fungi for rapid assessment of infection. Trans. Br. Mycol. Soc: 55: 158-161. [ Links ]
Ramyabharathi, S. A., and T. Raguchander. 2014. Characterization of antifungal antibiotic synthesis genes from different strains of Bacillus subtilis. J. Pure Appl. Microbiol. 8: 2337-2344. [ Links ]
Sánchez, R. G. 2008. La red de valor fresa: Sistema de inteligencia de mercados. Fundación Produce Michoacán. 145 p. [ Links ]
Sanogo, S., and P. W. Bosland. 2013. Biology and management of Phytophthora capsici in the Southwestern USA. In: Lamour, K. (ed). Phytophthora: A Global Perspective. CABI Plant Protection Series, Oxfordshire. pp: 87-95. [ Links ]
SAS Institute Inc. 2002. The SAS system for Windows version 9.0 SAS Institute Inc. Cary, NC. USA. [ Links ]
SIAP (Servicio de Información Agroalimentaria y Pesquera). 2014. Cierre de la producción agrícola por estado Cierre de la producción agrícola por estado http://www.siap.gob.mx/cierre-de-la-produccion-agricola-porestado/ (Consulta: Julio 2016). [ Links ]
Sicuia, O-A., I. Grosu, F. Constantinescu, C. Voaides, and C. P. Cornea. 2015. Enzymatic and genetic variability in Bacillus spp. strains with plant beneficial qualities. AgroLife Scient. J. 4: 124-131. [ Links ]
Vosatka, M., M. Gryndler, J. Jansa, and M. Vohnik. 2000. Post vitro mycorrhization and bacterization of micropropagated strawberry, potato and azalea. Acta Hort. 530: 313-324. [ Links ]
Whipps, J. M. 2001. Microbial interactions and biocontrol in the rhizosphere. J. Exp. Bot. 52: 487-511. [ Links ]
Received: September 2015; Accepted: August 2016