Serviços Personalizados
Journal
Artigo
Indicadores
-
Citado por SciELO
-
Acessos
Links relacionados
-
Similares em SciELO
Compartilhar
Agrociencia
versão On-line ISSN 2521-9766versão impressa ISSN 1405-3195
Agrociencia vol.50 no.5 Texcoco Jul./Ago. 2016
Crop Science
Flowering and fructification of Physalis peruviana L. by ammonium and nitrate application, vigor and plant age
1Edafología. Campus Montecillo. Colegio de Postgraduados. 56230. Montecillo, Estado de México, México (msandoval@colpos.mx)
The consumption and economic value of Cape gooseberry fruit (Physalis peruviana L.) increase due to its flavor, aroma and nutritional and medicinal qualities. The simultaneous application of NH4 + and NO3 - increases carbohydrates and proteins of the plant and favors yield, with respect to the application of only NO3 -. However, the species respond differently to NH4 + and NO3 - and age of the plant (shoots with high, medium and low vigor against new plants derived from seed). The study was conducted in a greenhouse; the treatments of the first experiment resulted from the combination of the ratio NH4 +/NO3 - (0:100, 25:75 and 50:50) and shoot vigor (low, medium and high). In another experiment a new lot of plants derived from seed was used, and with the same ratios NH4 +/NO3 -. An ANOVA was carried out with the results and treatments means were compared with the Tukey test (p≤0.05). In shoot plants, maximum SPAD readings, number of floral buds, flowers and green fruits were presented in plants with high and medium vigor, and with 25 and 50 % of NH4 + (p≤0.05). In seed plants, the NH4 + increased SPAD readings, number of floral buds, flowers and green fruits (p≤0.05). A joint application of NH4 + and NO3 - favors flowering and fructification, but reduces SPAD; in contrast, shoot vigor is not determinative but is consistent over SPAD.
Key words: Cape gooseberry; nitrogen; ratio NH4 +/NO3 -; shoot vigor
El consumo y el valor económico del fruto de uchuva (Physalis peruviana L.) aumentan debido a su sabor, aroma y características nutricionales y medicinales. El suministro simultáneo de NH4 + y NO3 - aumenta los carbohidratos y las proteínas en la planta y favorece el rendimiento, respecto al suministro de solo NO3 -. Sin embargo, las especies responden diferente al NH4 + y NO3 - en la solución nutritiva. El objetivo del presente estudio fue evaluar la floración y fructificación de P. peruviana L., en respuesta a la aplicación conjunta de NH4 + y NO3 - y edad de la planta (brotes con alto, medio y bajo vigor y plantas producidas por semilla). El estudio se realizó en invernadero; los tratamientos del primer experimento resultaron de la combinación de la relación NH4 +/NO3 - (0:100, 25:75 y 50:50) y el vigor del brote (bajo, medio y alto). En otro experimento se usó un nuevo lote de plantas producidas por semillas, y con las mismas relaciones NH4 +/NO3 -. Con los resultados se realizó un ANDEVA y las medias de tratamientos se compararon con la prueba de Tukey (p≤0.05). En plantas de brote, los valores máximos de SPAD, número de botones florales, flores y frutos verdes se presentaron en plantas con vigor alto y medio, y con 25 y 50 % de NH4 + (p≤0.05). En plantas de semilla, el NH4 + incrementó las lecturas SPAD, número de botones florales, flores y frutos verdes (p≤0.05). Una aplicación conjunta de NH4 + y NO3 - favorece la floración y fructificación, pero disminuye SPAD; en contraste, el vigor de los brotes no es determinante pero sí consistente sobre SPAD.
Palabras clave: Uchuva; nitrógeno; relación NH4 +/NO3 -; vigor del brote
Introduction
The Cape gooseberry plant (Physalis peruviana L.) is wild, its origin and diversification are American, principally in the Andes valleys of Peru, Colombia and Ecuador. It was later introduced in Africa and India, and there are more than 80 varieties in the world (Medina, 1991). Its fruit are known since the time of the Incas, who used it for self-consumption. The plant was considered a weed, since its nutritional and commercial value were unknown (Fischer et al., 2014).
The consumption and economic value of the fruit has increased, because it is considered an exotic fruit due to its aroma, sweet flavor and nutraceutic qualities. The fruit is mainly consumed fresh or processed, in juices, marmalades, conserved in syrup, wines and sauces. Among its nutraceutic properties, it contains vitamin A (3000 UI of carotene), vitamin C (43 mg), iron (1.70 mg) and phosphorus (38 mg) (Fischer, 2000). It is used as an antioxidant and anti-inflammatory (Wu et al., 2006) and for its hypoglucemic activity (Mushagalusa-Kasali et al., 2013).
Nitrogen is the nutrient with the highest influence on the growth and fructification of the gooseberry plant, promoting growth of stems, branches, leaves and fruits (Samra and Arora, 1997; Navarro and Navarro, 2000), which determines the reproductive growth of the plant. It is absorbed during the vegetative stage and is stored in the meristematic tissues for its translocation (Navarro and Navarro, 2000). According to Fischer and Angulo (1999), nitrogen is the nutrient with the most influence on yield of gooseberry plants; Its deficiency is manifested in a reduction of the number and length of branches, provoking reduction in size and quality of the fruits.
An adequate supply of N in the plant accelerates the rates of division and cellular differentiation, as well as photosynthetic activity, which increases vegetative or reproductive biomass through a greater efficiency in the interception and conversion of radiation (Uhart and Andrade, 1995). Nitrogen content in the biomass of the plant varies from 1 to 5 % (Hammad et al., 2007; Marschner, 2012). The plant absorbs the N in the form of NO3 -, and to a lesser extent, as NH4 +. The NO3 - in the plant converts to NH4 + and is used for the synthesis of proteins (Adams et al., 1984). NO3 - is absorbed more because the NH4 + is oxidized to NO3 - due to the action of the nitrifying bacteria (Taiz and Zeiger, 2010). However, NH4 + would be the preferred source of N due to its low energetic cost for the synthesis of proteins, compared to NO3 -, although in high concentrations it is toxic for the plant. Therefore, the plants that use NH4 + as N source present higher levels of carbohydrates and proteins with respect to the plants that use NO3 -. However, not all of the species behave the same when these two nitrogen sources are found in the culture medium (Echeverría and Sainz, 2005).
A combined supply in adequate proportions of NH4 + and NO3 - favors most of the species, by providing a balance in the metabolism and an efficient absorption of N (Hageman, 1992; Villalobos, 2001). According to Britto Kronzucker (2002), the absorption of NH4 + is influenced by the presence of NO3 - and K+ (Szczerba et al., 2006) in the solution, which could reduce the toxicity of the NH4 +. The absorption of NO3 - increases the concentration of some of the growth hormones in the xylem (Rahayu et al., 2005), regulating cellular division and expansion (Francis and Sorell, 2001), and the NH4 + absorbed by the root is incorporated to the amino acids or other compounds that contain reduced N (Resh, 2001), thus the energy demand is lower (5 moles of ATP) compared with the nitrate (12 moles of ATP). However, high proportions of NH4 + cause physiological disorders such as a lower concentration of Ca+2, K+ and Zn+2 in leaves.
Based on the above, the objective of this research was to evaluate the flowering and fructification of P. peruviana L., with the joint application of NH4 + and NO3 - and age of the plant (shoots with high, medium and low vigor against new plants derived from seed). The hypothesis was that the presence of NH4 + and NO3 ‒ in the nutrient solution and vigorous shoots improve the morphology of the plant.
Materials and Methods
The study was conducted from August to December of 2013, in a tunnel type greenhouse, with polyethylene UVII-720 cover, and galvanized steel structure with lateral ventilation. Maximum temperature was 38 °C and minimum 9 °C, with a light intensity of 653.43 mmol m-2 s-1, at the Montecillo Campus of the Colegio de Postgraduados, Estado de Mexico. The gooseberry plants were of two ages: one was the established plants of two years of age; the other was new plants obtained from seed. The plant material used was provided by the Plant Nutrition Area. The treatments of the first experiment resulted from the combination of the NH4 +:NO3 - (0:100, 25:75 and 50:50) and shoot vigor (low, medium and high; 10, 15, 20 cm height, respectively). In the second experiment we used new plants derived from seed and with the same ratios of NH4 +:NO3 - as the first experiment. The experimental design in both experiments was completely randomized, with five replicates in shoot plants and four replicates in seed derived plants. The experimental unit was one plant placed in a black polyethylene bag with 19 L capacity, with tezontle as substrate, with granulometry of 1 to 10 mm, average apparent density of 0.82 g cm―3, 50 % total porosity, 45 % aeration porosity, 5.42 % of available water, without cation exchange capacity and electric conductivity close to zero (Gutiérrez et al., 2011). The plants were maintained in vertical position with tutoring using raffia placed along the rows and attached to wooden posts at the ends. The nutrient solutions were made based on universal Steiner solution (1984) which was modified according to the treatments, by using N in the form of NH4 +, given that originally NO3 - is used and also based on the best treatment found by Gastelum et al. (2013), which was the Steiner solution with 50 % of its original ionic strength; the pH of the solutions was adjusted to 6.0.
The variables evaluated were the number of floral buds, flower and green fruits per plant, respectively, and SPAD readings, for which measurements were carried out every 15 d. In shoot plants, the quantification of the number of floral buds, flowers and green fruits was made from 41 d after pruning (dap), whereas the measurement of SPAD readings started 15 dap. In seed derived plants, the count of the number of floral buds, flowers and green fruits began 41 d after transplant (dat) and the SPAD readings initiated 60 dat. For the SPAD readings, the chlorophyll detector meter SPAD 502 (Minolta SPAD, Osaka, Japan) was used from the laboratory of Plant Nutrition of the Postgraduate Soil Science department. The measurements were made in five recently matured leaves located in the upper portion of each plant and an average was obtained. For the number of floral buds, flowers and green fruits, their total number in the plant was counted, in each sampling.
The analysis of variance was made to confirm the effect of the ammonium:nitrate ratio and shoot vigor; the mean of the treatments were compared with the Tukey test (p≤0.05). In the second experiment the effect of the ammonium:nitrate ratio was evaluated. The analyses were made with SAS (SAS, 2002) version 9.1.
Results and Discussion
Plants from shoot
The number of floral buds per plant changed at 71 dap due the joint application of NH4 + and NO3 -; the maximum value was in plants treated with NH4 + in the nutrient solution. Plant vigor only showed positive effect in the last sampling, at 116 dap; the highest number of floral buds was found in plants with high and medium vigor (Table 1).
Table 1: Number of floral buds in gooseberry (Physalis peruviana L.) plants derived from shoots in response to the effects of the ratio NH4 +/NO3 - and plant vigor.
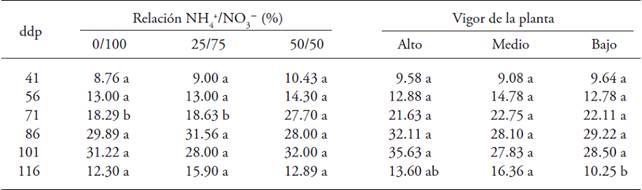
♦ Different letters in a row and factor indicate significant differences (p≤0.05). dap = days after pruning.
Morgan (2001) evaluated the effect of proportions of NH4 + (0, 10, 20 and 30 %) in spinach plants (Spinacia oleracea L.) cultivated in NFT system and with low light, and found that by increasing the percentage of NH4 + in the nutritive solution, flowering is accelerated; the highest values were found with 30 % of NH4 +, and the lowest with 0 and 10 % of NH4 +.
An excessive application of NH4 + increases the concentration of reduced N in the plant, which causes a higher production of polyamines that are associated with the increment in the synthesis of gibberellin, DNA, RNA and proteins (Amberger, 1984). To this respect, Marschner (2012) indicates that the foliar application of urea increases plant vigor, as it is a source of N that participates in the synthesis of amino acids, and is responsible for causing hormonal changes in the plant, by increasing the concentration of cytokinins.
Plant vigor is directly correlated with the capacity of the root to store a reserve energy source, which continues throughout its growth period. Afterwards, storage of the reserves (photo-assimilates) declines because it is used by the plant to initiate flowering, due to the fact that most of the N present in the energy reserve is directed to the formation of flowers and fruit ripening, or otherwise, to continue with vegetative growth (Taiz and Zeiger, 2010).
The ammonium-nitrate ratio affected the number of flowers per plant at 116 dap (Table 2); the highest number of flowers appeared in plants treated with the 50:50 ratio (9 flowers). Plant vigor had influence at 86 and 116 dap; the plants with high (15 flowers) and medium vigor (10 flowers) presented the highest number of flowers per plant in each sampling, respectively (Table 2).
Table 2: Number of flowers in gooseberry (Physali peruviana L.) plants derived from shoots in response to the effects of the ratio NH4 +:NO3 - and plant vigor

♦ Different letters in a row and factor indicate significant differences (p≤0.05). dap = days after pruning.
High levels of N inhibits flowering and promotes vegetative growth (Lovatt, 1990). Therefore, concentrations lower than 30 % NH4 + of the total N favor from 3 to 7 d the precocity of flowering, and have a positive effect on the number of inflorescences/ stem, stem length and green color of the leaves (Kasten and Sommer, 1990; Stensvand and Gisrelod, 1992). In citruses (Citrus spp.) there is a relationship between the intensity of flowering and the content of reduced N and polyamines in the plant can increase with foliar applications of urea (Lovatt, 1990).
Plant size serves as an indicator of yield, thus vigorous growth and an adequate differentiation of the apical meristem of the plant will allow higher productivity by determining to a great extent the number of flowers formed, as a result of the dynamic of the process and cellular expansion that occurs in the interface, which depends on the rate of photosynthesis and the distribution of assimilates. Furthermore, the number of flowers will vary according to the genotype, environmental condition, plant health and management (Taiz and Zeiger, 2010; Jemmali and Boxus, 1993; Stapleton et al., 2001).
The application of NH4 +:NO3 - at 41 dap affected the number of green fruits per plant (Table 3); plants treated with a higher proportion of NH4 + in the nutrient solution had a higher number, but from 56 to 116 dap no effect was found of the ratio NH4 +:NO3 -. Plant vigor influenced at 41, 101 and 116 dap; the maximum value was for plants with high vigor (Table 3).
Table 3: Number of green fruits in gooseberry (Physalis peruviana L.) plants derived from shoots in response to the effects of the ratio NH4 +:NO3 - and plant vigor.
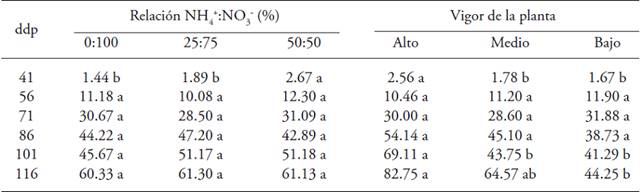
♦ Different letters in a row and factor indicate significant differences (p≤0.05). dap = days after pruning.
The combination of NO3 - with low amounts of NH4 + produces higher growth; however, the optimum proportion differs among the species and could change with the age of the plant (Haynes, 1986). High concentrations of NH4 + accumulate in the young tissues, in this case, in shoots, and cause a reduction in photosynthesis, growth and crop production (Marques et al., 1983).
The growth and productivity of each plant are determined by its genetics, environmental conditions, availability of water and nutrition. However, variations in these aspects are related to vigor and the amount of assimilates in the plant, as energy reserve for zones of high demand. In plants such as tomato (Solanum lycopersicum L.), vigor has a very strong influence over the distribution of the assimilates over the long term and affects the number of fruits on the plant (Papadopoulos and Ormrod, 1990). Therefore, an increment in vigor results in an increase in the number of fruits, in simultaneous growth, and indirectly participates in the generation of biomass (Heuvelink, 1995).
The ratio NH4 +/NO3 - and plant vigor affected the SPAD readings (Table 4) at the start of the cycle. The ratio NH4 +:NO3 - only affected the SPAD readings at 30 dap, and the maximum value was in plants treated with the ratio 50/50 (47.50). Plant vigor influenced the SPAD reading at 15 dap, and the highest reading was in plants with high vigor (39.30), whereas from 45 to 105 dap there was no effect of the ratio NH4 +:NO3 - nor of plant vigor.
Table 4: SPAD readings in gooseberry (Physalis peruviana L.) plants derived from shoot in response to the ratio NH4 +/NO3 - and plant vigor.
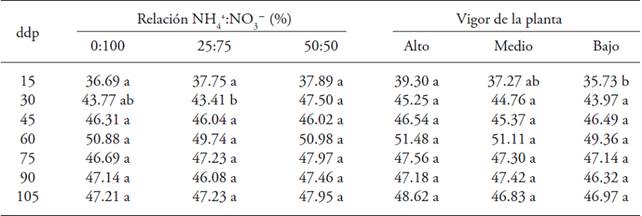
♦ Different letters in a row and factor indicate significant differences (p≤0.05). dap = days after pruning.
Chlorophyll content and N absorption correlated with the SPAD readings under diverse environmental conditions, such as light intensity, temperature, relative humidity, pests, population density, nitrogen source (Sandoval-Villa et al., 1999; Dong et al., 2004). To this respect, Sandoval-Villa et al. (1999) found that the SPAD readings in tomato plants increase when the concentration of NH4 + is increased by 25 % in the nutritive solution; in contrast, high concentrations of NH4 + reduce the value of the SPAD readings.
For a vigorous growth, the plant must have an energy reserve. The carbohydrates that comprise this reserve are used to initiate new growth after each pruning, and to survive under stress conditions (Ho et al., 1983; Hellman et al., 2000; Stapleton et al., 2001). The plant does not use energy to synthesize its amino acids because it absorbs them directly from the soil solution. Its means of action are: 1) as supplement of high consumption amino acids, which include glycine, necessary for the synthesis of porphyrins that are structural components of chlorophyll and the cytochromes and; 2) the formation of biologically active substances that provide vigor and stimulate the plant in critical periods, and in highly intensive productions (Fischer et al., 1998; Lalonde et al., 2004).
Seed derived plants
The application of NH4 + and NO3 - had positive influence on the number of floral buds, flowers and green fruits per plant; the highest quantity appeared in plants treated with NH4 + in the nutrient solution (Table 5). In the number of floral buds and flowers per plant varied constantly and decreased with time. The number of green fruits per plant, the ratio NH4 +: NO3 - had a constant effect throughout the cycle, and the highest number of fruits was found in plants treated with the ratio 25:75, except at the end of the cycle, at 116 dat, when there was no effect from the ratio NH4 +:NO3 - (Table 5). This response is attributed to the aging of the plant or to the effect of the form of N present in the medium, due to the fact that it participates importantly in the synthesis of proteins and formation of chlorophyll, vegetative growth and the determination of the number of flowers and fruits. However, this reduction could be attributed to a deficiency in phosphorus, which retards the development of the plant and delays the differentiation of the floral buds, causing a reduction in the number of fruits per plant (Rodríguez et al., 1989; Menezes dos Santos, 1992).
Table 5: Table 5. Number of floral buds, flowers and green fruits in gooseberry plants (Physalis peruviana L.) derived from seed in response to the ratio NH4 +:NO3 -.

♦ Different letters in a row indicate significant differences (p≤0.05). dat = days after transplant.
Similar results were obtained by Bugarín et al. (1988), who evaluated the response to different concentrations of NH4 +: cations in the nutritient solution in the growth and flowering of three varieties of chrysanthemum [Dendranthema x glandiflorum (Ramat) Kitam.]: Puma, Funflower and Improved Funshine, and found a positive effect of the NH4 + on the number of inflorescences at the harvest. The maximum values were obtained with the concentrations 4.5 and 3.0 meq L-1 of NH4 +. Sandoval et al. (1992) indicate that in wheat (Triticum aestivum L.), a higher production of grain and dry matter can be obtained by using NH4 + in percentages lower or equal to 50 % of the amount of N applied, with respect to the exclusive application of NO3 - to the plant. Furthermore, Osorio et al. (2003) and Chen et al. (1998) observed a higher production of biomass in plants treated, as compared to an independent utilization of respect to plants treated independently with NH4 + or NO3 -.
The ratio NH4 +:NO3 - did not cause significant differences over the SPAD readings throughout the crop cycle (Table 6). The maximum values of SPAD readings were reached in plants treated exclusively with nitrate (0/100), with respect to those treated with NH4 + in the nutrient solution.
Table 6: SPAD readings in gooseberry plants (Physalis peruviana L.) derived from seed in response to the ratio NH4 +:NO3 ― .

♦ Different letters in a row indicate significant differences (p≤0.05). dat = days after pruning.
After 90 dat, there were SPAD readings lower than those registered in the other samplings, which is related to the stage of flowering-fructification of the plant, thus the demand of N is higher toward fruit development (Sandoval-Villa et al., 2001). To this respect, Rodríguez et al. (1998) found a high correlation among the SPAD readings, the concentration of extractible chlorophyll in the fifth leaf and the percentage of N in tomato plants cultivar Rio Grande. Similarly, there is a direct relationship between the SPAD readings and the concentration of N in the plant, where the plants fertilized adequately with NO3 - as principal source of N present a green color in their leaves (Villar and Ortega, 2003). The contrary was reported by González et al. (2009), who evaluated different ratios NH4 +:NO3 - in the production of aromatic herbs in hydroponics and did not find statistical differences among the SPAD readings in chives (Allium schoenoprasum Regel & Tiling), basil (Ocimum basilicum L.) and dill (Anethum graveolens L.). Maximum values were found in plants treated with NH4 + in the nutrient solution, and the best ratio was 20:80. Besides, Kond and Higuchi (2012) indicate that the application of N in the form of ammonium provokes low SPAD values in Passiflora edulis.
The orthogonal contrast test, to compare the effect of the origin of the plant (seed and shoot), significant differences (p≤0.05), for the showed SPAD readings and the total number of green fruits per plant. The highest SPAD readings and the highest yield of green fruits per plant were found in new plants obtained from seed (Table 7). These differences are due to the fact that gooseberry propagates mainly and without problems, from seed; which could originate genetic variability. Sandhu et al. (1989) point out that the gooseberry propagated by seed varies in growth, vigor, yield and fruit quality. To this respect, Angarita and Santana (1997) indicate that gooseberry presents great phenotypic variability, being a pollinated plant of sexual propagation; therefore, in the plants obtained from seed, growth, yield and fruit quality are variable.
Conclusions
In plants derived from shoot, the joint application of ammonium and nitrate, and plant vigor did not affect the number of floral buds, flowers and green fruits per plant, nor the value of SPAD readings; only the effect of shoot vigor was observed over the number of green fruits per plant.
In seed derived plants, the presence of ammonium in the nutritive solution incremented the number of floral buds, flowers and green fruits per plant, in contrast, the SPAD readings were not affected.
Literatura Citada
Adams, C. R., K. Bamford M., y P. Early M. 1984. Principios de la Hortofruticultura. Acribia. España. 316 p. [ Links ]
Amberger, A. 1984. Uptake and metabolism of hydrogen cyanamide in plants. Proceeding of bud dormancy in grapevines: Potential and practical uses of hydrogen cyanamide. UCD. pp: 5-10. [ Links ]
Angarita, A., y G. Santana. 1997. Regeneración adventicia de somaclonales en uchuva (Physalis peruviana). Agron. Colomb. 14: 59-65. [ Links ]
Britto, D. T., and H. J. Kronzucker. 2002. NH4 + toxicity in higher plants. J. Plant Physiol. 159: 567-584. [ Links ]
Bugarín M., R., G. A. Baca C., J. Martínez H., J. L. Tirado T., y G. Martínez A. 1998. Amonio/nitrato y concentracion ionica total de la solución nutritiva en crisantemo. I. Crecimiento y floración. Terra 16: 113-124. [ Links ]
Chen, J. G., S. H. Cheng, W. Cao, and X. Zhou. 1998. Involvement of endogenous plant hormones. In: the effect of mixed nitrogen source on growth and tillering of wheat. J. Plant Nutr. 21: 87-97. [ Links ]
Dong, C., Q. Shen, and G. Wang. 2004. Tomato growth and organic acid changes in response to partial replacement of NO3 .-N by NH4 +-N. Pedosphere 14: 159-164. [ Links ]
Echeverría, H., E., y H. R. Sainz. 2005. Nitrógeno en el suelo. In: Echevarría, H. E., y F. García (eds). Fertilidad de Suelos y Fertilización de Cultivos. Ediciones INTA, Buenos Aires, Argentina. pp: 69-97. [ Links ]
Fischer, W. N., B. André, D. Rentsch, S. Krolkiewicz, M. Tegeder, K. Breitkreuz, and W.B. Frommer. 1998. Amino acid transport in plants. Trends Plant Sci. 3: 188-195. [ Links ]
Fischer, G., y R. Angulo. 1999. Los frutales de clima frío en Colombia. La uchuva. Ventana al Campo Andino 2: 3-6. [ Links ]
Fischer, G. 2000. Crecimiento y desarrollo. In: Flórez, V. J., G. Fischer, y A. D. Sora (eds). 2000. Producción, Poscosecha y Exportación de la Uchuva (Physalis peruviana L.). Unibiblos, Universidad Nacional de Colombia, Bogotá. pp: 9-26. [ Links ]
Fischer, G., P. J. Almanza-Merchán, y D. Diego Miranda. 2014. Importancia y cultivo de la uchuva (Physalis peruviana L.). Rev. Bras. Frutic. 36: 1-15. [ Links ]
Fleming, L. A., D. T. Krizek, and R. M. Mirecki. 1987. Influence of ammonium nutrition on the growth and mineral composition of two chrysanthemum cultivars differing in drought tolerance. J. Plant Nutr. 10: 1869-1881. [ Links ]
Francis, D., and D. A Sorell. 2001. The interface between the cell cycle and plant growth regulators: a mini review. Plant Growth Regul. 33: 1-12. [ Links ]
Gastelum O., D. A., M. Sandoval V., C. Trejo L., y R. Castro B. 2013. Fuerza iónica de la solución nutritiva y densidad de plantación sobre la producción y calidad de frutos de Physalis peruviana L. Rev. Chapingo Serie Hort. 19: 197-210. [ Links ]
González G., J. L., M. N. Mendoza R., P. Sánchez G., y E. A. Gaytan A. 2009. Relación amonio/nitrato en la producción de hierbas aromáticas en hidroponía. Agric. Téc. Méx. 35: 5-11. [ Links ]
Gutiérrez C., M. C., Hernández Escobar, J., C. A., Ortiz Solorio, R., Anicua Sánchez, y M. E. Hernández Lara, 2011. Relación porosidad-retención de humedad en mezclas de sustratos y su efecto sobre variables respuesta en plántulas de lechuga (Lactuca sativa L.). Rev. Chapingo Ser. Hortic. 17: 183-196. [ Links ]
Hageman, R. H. 1992. Ammonium versus nitrate nutrition of higher plants. In: Nitrogen in Crop Production. American Society of Agronomy, Crop Science Society of America and Soil Science Society of America. Madison, WI, USA. pp: 67-68. [ Links ]
Hammad, S. A., M. A. Abou-Seeda, A. M. El-Ghamry, and E. M. Selim. 2007. Nitrate accumulation in spinach plants by using N-fertilizer types in alluvial soil. J. Appl. Sci. Res. 3: 511-518. [ Links ]
Haynes, R. J. 1986. Uptake and assimilation of mineral nitrogen by plants. In: Haynes, R. J., K. C. Cameron, K. M. Goh, and R. R. Sherlock (eds). Mineral Nitrogen in the Soil Plant System. Academic Press. Orlando, Florida, USA. pp: 303-358. [ Links ]
Hellman, H., L. Barker, and W. Fromemer. 2000. The regulation of assimilate allocation and transport. Austr. J. Plant Physiol. 27: 583-594. [ Links ]
Heuvelink, E. 1995. Effect of plant density on biomass allocation to the fruits in tomato (Solanum lycopersicum L.). Scientia Hortic. 64: 193-201. [ Links ]
Ho, L., V. Sjut, and V. Hoad. 1983. The effect of assimilate supply on fruit growth and hormone levels in tomato plants. Plant Growth Reg. 1: 155-171. [ Links ]
Jemmali, A., and P. Boxus. 1993. Early estimation of strawberry floral intensity and its improvement under cold greenhouse. Acta Hort. 348: 357-359. [ Links ]
Kasten, P., and K. Sommer. 1990. Cultivation of cut flowers with ammonium as nitrogen source. In: Van Beusichem, M. L. (ed). Plant Nutrition, Physiology and Application. Kluwer Academic. Publisher. pp: 533-537. [ Links ]
Kond, T. and Higuchi, H. 2012. Effects of nitrogen form and concentration in fertilizer on vegetative growth, flowering, and leaf mineral contents of Passion fruit (Passiflora edulis). Trop. Agr. Develop. 56:123-128. [ Links ]
Lalonde, S., D. Wipf, and W. B. Frommer. 2004. Transport mechanisms for organic forms of carbon and nitrogen between source and sink. An. Rev. Plant Biol. 55: 341-372. [ Links ]
Lovatt, C. J. 1990. The role of nitrogen in citrus flowering and fruit set. Low-input citrus management. University of California. Riverside, USA. 10 p. [ Links ]
Marschner, P. 2012. Mineral Nutrition of Higher Plants. 3a ed. Academic Press. London, U.K. 651 p. [ Links ]
Marques, Y. A., M. J. Oberholzer, and K. H. Erismann. 1983. Effects of different nitrogen sources on photosynthetic carbon metabolism in primary leaves of non nodulated Phaseolus vulgaris L. Plant Physiol. 71:555-561. [ Links ]
Medina, M. 1991. El cultivo de la uchuva tipo exportación. Rev. Agric. Trop. Palmira 2: 55-58. [ Links ]
Menezes dos Santos, J. R. 1992. Producción de tomate en América Latina y el Caribe. In: Izquierdo J., G. Paltrinieri, y C. Arias (eds). Producción, Postcosecha, Procesamiento y Comercialización de Ajo, Cebolla y Tomate. FAO. Santiago, Chile. pp: 173-215. [ Links ]
Morgan, L., 2001. El gran debate: amonio vs nitrato ¿Cómo quieren las plantas el nitrógeno? Practical Hidroponics & Greenhouse No. 50. In: Rodríguez D., A. (ed). RED Hidroponía, 2001. Boletín Informativo No. 10. Centro de Investigación de Hidroponía y Nutrición Mineral. Universidad Nacional Agraria La Molina. Lima. Perú. [ Links ]
Mushagalusa-Kasali, F., J. Ntokamunda-Kadima, P. Tshimankinda-Mpiana, Koto-te-Nyiwa Ngbolua, and D. Sha-Tshibey-Tshibangu. 2013. Assessment of antidiabetic activity and acute toxicity of leaf extracts from Physalis peruviana L. in guinea-pig. Asian Pac. J. Trop. Biomed. 3: 841-846. [ Links ]
Navarro, S., y G. Navarro. 2000. Química Agrícola. Ediciones Mundi-Prensa, Madrid. España. 487 p. [ Links ]
Osorio, N. W., X. Shuai, S. Miyasaka, B. Wang, R. L. Shirley, and W. J. Wigmore. 2003. Nitrogen level and form affect taro growth and nutrition. Hort. Sci. 38: 36-40. [ Links ]
Papadopoulos, A., and D. Ormron. 1990. Plant spacing effects on yield of the greenhouse tomato. Canadian J. Plant Sci. 70: 565-573. [ Links ]
Rahayu, Y. S., P. Walch-Liu, G. Neumann, V. Romheld, N. Von Wiren, and F. Ban Gerth. 2005. Root-derived cytokinins as long-distance signals for NO3 - induced stimulation of leaf growth. J. Exp. Bot. 56:1143-1152. [ Links ]
Resh, H. M. 2001. Cultivos Hidropónicos. Mundi-Prensa. Madrid, España. 558 p. [ Links ]
Rodríguez, J. A., J. M Tabares, y J. A. Medina. 1989. Técnicas de cultivo. In: Cultivo Moderno del Tomate. Editorial Mundi-Prensa. Madrid. España. 189 p. [ Links ]
Rodríguez, M., G. González, A. Aguilar, J. Etchevers, y J. Santizo R. 1998. Estimación de la concentración de nitrógeno y clorofila en tomate mediante un medidor portátil de clorofila. Terra 16: 135-141. [ Links ]
Samra, J. S., and Y. K. Arora. 1997. Mineral nutrition. In: Litz, R. E. (ed). The Mango: Botany, Production and Uses. CAB International. pp: 175-201. [ Links ]
Sandhu, A. S., S. N. Singh, P. P. S. Minhas, and G. P. Grewal. 1989. Rhizogenesis of shoot cuttings of raspberry (Physalis peruviana L.). Indian J. Hort. 46: 376-378. [ Links ]
Sandoval V. M., G. Alcántar G., J. L. Tirado T., and A. Aguilar S. 1992. Effect of the NH4 +/NO3 - ratio on GS and PEP Case activities and on dry matter production in wheat. J. Plant Nutr. 15:2545-2557. [ Links ]
Sandoval-Villa, M., C. W. Wood, and E. A. Guertal. 1999. Ammonium concentration in solution effects chlorophyll meter readings in tomato leaves. J. Plant Nutr. 22: 1717-1729. [ Links ]
Sandoval-Villa, M., E. A. Guertal, and C. W. Wood. 2001. Greenhouse tomato response to low ammonium-nitrogen concentrations and duration of ammonium-nitrogen supply. J. Plant Nutr. 24: 1787-1798. [ Links ]
SAS Institute. (2002). SAS/STAT User’s Guide. Version 9.1. Volumes 1-7. SAS Institute, Cary, NC, USA. [ Links ]
Stapleton, S. C., C. K. Chandler, D. E. Legard, J. E. Price, and J. C. Sumler. 2001. Transplant source affects fruiting performance and pests of ‘Sweet Charlie’ strawberry in Florida. Hor. Technol. 11: 61-65. [ Links ]
Steiner, A. A. 1984. The universal nutrient solution. In: Proceedings of the 6th International Congress on Soilless Culture. ISOSC. Wageningen, The Netherlands. pp: 633-649. [ Links ]
Stensvand, A., and H. R. Gislerod. 1992. The effect of the NO3 -/ NH4 + ratio of nutrient solution on growth and mineral uptake in Chrysanthemum morifolium, Passiflora coeruka and Cordylim frusticula. Garttenbau-missenschaff 57: 193-198. [ Links ]
Szczerba, M. W., D. T. Britto, and H. J. Kronzucker. 2006. Rapid, futile K+ cycling and pool-size dynamics define low-affinity potassium transport in barley. J. Plant Physiol. 141:1494-1507. [ Links ]
Taiz, L., and E. Zeiger. 2010. Plant Physiology. 5a ed. Sinaver Associates. Sunderland, MA. USA. 782 p. [ Links ]
Uhart, S. A., and F. H. Andrade. 1995. Nitrogen deficiency in maize: I. Effects on crop growth, development, dry matter partitioning, and kernel set. Crop Sci. 35:1376-1383. [ Links ]
Villalobos, R. E. 2001. Fisiología de la Producción de los Cultivos Tropicales. Universidad de Costa Rica (ed). San José, Costa Rica. 227 p. [ Links ]
Villar, D., y R. Ortega. 2003. Medidor de clorofila. Bases teóricas y su aplicación para la fertilización nitrogenada en cultivos. Agron. For. UC. 18:4-8. [ Links ]
Wu, S. J., J.Y. Tsai, S. P. Chang, D. L. Lin, S. S. Wang, S. N. Huang, and L. T. Ng. 2006. Supercritical carbon dioxide extract exhibits enhanced antioxidant and anti-inflammatory activities of Physalis peruviana L. J. Ethnopharmacol. 108: 407-413. [ Links ]
Received: October 2015; Accepted: April 2016