Serviços Personalizados
Journal
Artigo
Indicadores
-
Citado por SciELO
-
Acessos
Links relacionados
-
Similares em SciELO
Compartilhar
Agrociencia
versão On-line ISSN 2521-9766versão impressa ISSN 1405-3195
Agrociencia vol.50 no.2 Texcoco Fev./Mar. 2016
Biotechnology
Antifungal activity and molecular identification of native strains of Bacillus subtilis
1 División de Estudios de Postgrado e Investigación, Instituto Tecnológico de Conkal, Conkal, Yucatán, México.
2 Universidad Autónoma Metropolitana Iztapalapa, Laboratorio Divisional de Biología Molecular, División de Ciencias Biológicas y de la Salud, Rafael Atlixco 186, Colonia Vicentina, México.
3 Universidad de Guadalajara, Centro Universitario de Ciencias Exactas e Ingenierías, Bulevard Marcelino García Barragán 1421, Colonia Olímpica, Guadalajara, Jalisco, 44430 México.
4 Universidad de Guadalajara, Centro Universitario de la Ciénega. Avenida Universidad 1115, Colonia Lindavista, Ocotlán, Jalisco, 47810, México. (botin77@gmail.com).
The bioprospection for antagonistic bacteria, as biological control agents to manage plant pathogenic fungi, is a preponderant activity to reduce the use of chemical fungicides. The bacterium Bacillus subtilis has shown an excellent capacity to inhibit the growth and sporulation of a wide variety of plant pathogenic fungi. The phenotypic and biochemical characteristics to differentiate among subspecies of B. subtilis vary at the regional level. In this research, Bacillus strains were isolated from the Yucatan Peninsula in Mexico and their antagonistic activities were assessed by direct confrontation in a completely randomized design. To identify the most active strains, molecular characterization was performed. For an accurate identification, partial 16S rRNA, gyrA and rpoB genes were sequenced and compared with those deposited in the GenBank. The most active strains CBCC2, CBMT2, CBMN22, CBRF24, CBCK36, CBCK47, and CBMT51 caused 32 to 78 % inhibition of mycelial growth in Alternaria alternata, Helminthosporium rostratum and Curvularia lunata in direct confrontation tests; likewise the cell-free culture filtrates of these Bacillus strains showed significant differences (p≤0.05) in the degree of inhibition of conidial germination of the plant pathogenic fungi. The amplification, sequencing and comparison of 16S rRNA, gyrA and rpoB genes showed that the strains had 95 to 100 % identity with Bacillus subtilis subsp. inaquosorum and B. subtilis subsp. subtilis. The gyrA gene showed the highest evolutionary rate using the analysis of phylogenetic relationship within the strains studied.
Key words: Bacillus subtilis; biocontrol; gene-protein sequence; DNA gyrase; RNA polymerase
La bioprospección de bacteria antagónicas, como agentes de control biológico para el manejo de hongos fitopatógenos, es una actividad preponderante para reducir el uso de fungicidas químicos. La bacteria Bacillus subtilis ha mostrado una excelente capacidad para inhibir el crecimiento y esporulación de una gama amplia de hongos fitopatógenos. Las características bioquímicas para diferenciar entre subespecies de B. subtilis varían a nivel regional. En esta investigación se aislaron cepas de Bacillus de la Península de Yucatán en México y se evaluaron sus actividades antagónicas por confrontación directa en un diseño completamente al azar. Para identificar las cepas más activas se realizó una caracterización molecular. Para una identificación precisa se secuenciaron parte de los genes 16S rRNA, gyrA y rpoB y se compararon con los depositados en el GenBank. Las cepas más activas CBCC2, CBMT2, CBMN22, CBRF24, CBCK36, CBCK47 y CBMT51 causaron la inhibición del crecimiento micelial de 32 a 78 % en Alternaria alternata, Helminthosporium rostratum y Curvularia lunata en pruebas de confrontación directa; asimismo, los filtrados de cultivos libres de células de estas cepas Bacillus mostraron diferencias significativas (p≤0.05) en el grado de inhibición de germinación conidial de los hongos fitopatógenos. La amplificación, secuenciación y comparación de los genes 16S rRNA, gyrA y rpoB mostraron que las cepas tenían una identidad de 95 a 100 % con Bacillus subtilis subsp. inaquosorum y B. subtilis subsp. subtilis. El gen gyrA mostró la tasa evolutiva más alta al usar el análisis de la relación filogenética con las cepas estudiadas.
Palabras clave: Bacillus subtilis; biocontrol; secuencia gen-proteína; DNA girasa; RNA polimerasa
Introduction
Fungi form a large eukaryotic group of organisms characterized by their chitinous cell walls and also by their lack of photosynthetic pigment. Although the majority of fungal species are saprophytes, a number of them are plant parasites, damaging different organs in cultivated and wild plants (Agrios, 2005; González-Fernández et al., 2010). Chemical fungicides are essential for the effective control of plant diseases (Knight et al., 1997). In the last two decades new classes of fungicides with novel modes of action were developed and new molecules will be identified for the foreseeable future (Knight et al., 1997; De Waard et al., 1993).
The extensive and irrational uses of synthetic fungicides caused damage to the environment and to human health due particularly to occupational exposure and to their residues in food products. In addition, resistant populations of fungi in high artificially managed agro-ecosystems are increasingly detected (Blasco et al., 2002; Waard et al., 2006). To cope with the risk associated to the use of chemical fungicides, antagonistic microorganisms represent a feasible alternative to manage plant pathogenic fungi. In this context, the bacterium Bacillus subtilis has shown an excellent capacity to inhibit in vitro and in vivo the growth and sporulation of a wide variety of plant pathogenic fungi (Korsten and Jager, 1995; Podile and Laxmi, 1998; Schisler et al., 2004).
The antagonistic effects of B. subtilis are associated at least to five mechanisms: 1) direct parasitism, where bacteria move to germinating spore and attach to the surface via polarity (Korsten and Jager, 1995); 2) production of extracellular antibiotics, like bacilomycin, iturin, mycosubtilin and zwittermicin (Pal and Gardener, 2006; Fickers, 2012); 3) production of lytic enzymes, like chitinase, protease, β-1,3 glucanase and cellulose (Kumar et al., 2012); 4) competition for nutrients on the host, which causes nutrient stress and starvation in the germinating fungus (Janiciewickz et al., 2003); and 5) stimulation of host defenses through induced systemic resistance via jasmonic acid pathway (Van Loon, 2007).
Bacillus subtilis has also shown other beneficial applications in agricultural production, environmental engineering and industrial processes. For example, some strains can promote plant growth in horticultural, field and fruit crops (Adesemoye et al., 2008; Lugtenberg and Kamilova, 2009). Bacillus subtilis can also be used for bioremediation of crude-oil and heavy metal polluted soils, as well as for bioremediation of textile industrial effluents (Augusto-Costa and Pereira-Duta, 2001; Cubitto et al., 2004; Ajao et al., 2011).
There are well-documented cases of success using Bacillus subtilis for production of antibiotic, surfactant and enzymes (Harwood, 1992; Price et al., 2007). Bacillus subtilis is a species commonly found in the environment, its taxonomy and that of related species has been extensively studied in the last two decades (Rooney et al., 2009). Bacillus subtilis and related species have high levels of similarity in their 16S rRNA gene sequence (99 % or higher), therefore their identity has to be aided by other genes that give more precision to the species level.
Moreover, there are few phenotypic and biochemical characteristics to differentiate among subspecies of B. subtilis, which make the scenario more difficult to identify species among the B. subtilis complex, as well as to resolve the evolutionary relationship of such species. In this respect, the use of amplification, sequencing and comparison of genes gyrA, that codify for the subunit A of DNA gyrase, and rpoB, that codifies for the subunit b of the RNA polymerase, may be integrated to the analysis of 16S rRNA to identify B. subtilis strains (Chun and Bae, 2000; Geetha et al., 2007; Geetha and Manonmani, 2008; Geetha and Manonmani, 2010).
This study was carried out to evaluate the antagonistic activity of native strains of Bacillus isolated from the Yucatan Peninsula, Mexico, and to identify the most active strains by molecular characterization using the genes 16S rRNA, gyrA and rpoB. The hypothesis was that the knowledge of the particular characteristics of endemic Bacillus strains will contribute to the enrichment of the genetic records in the region, with the possibility of future applications of such resources for the control of plant fungi.
Materials and methods
Isolation of Bacillus strains
The strains were isolated from soil samples collected in the Yucatan Peninsula in Mexico. Soil samples were taken from natural vegetated areas by scrapping off surface material with a sterile spatula and about 200 g samples were obtained from 10 to 20 cm depth. All samples were placed in sterile plastic bags and stored at 4°C until processed. Isolation was carried out using the methodology described by Basurto-Cadena et al. (2012). Briefly, 1 g of each sample was suspended in 10 mL sterile distilled water and pasteurized at 80°C for 20 min. Subsequently, 50 μL of the suspension was placed in microfuge tubes with 500 μL of distilled water.
Aliquots of 50 μL of the diluted suspensions were spread in duplicate on a thin layer of nutrient agar (NA) (Merck, Germany) in Petri dishes. The incubation was carried out under aerobic conditions at 30oC for 48 h. After incubation the Bacillus-like colonies, roughly identified based on their morphology, were transferred and incubated in new Petri dishes for one week at the above conditions, before storing at 4°C. The colonies were counted and the results expressed as bacteria count per milliliter (CFU mL-1). Prior to use, all isolates were reactivated in NA for five days.
Evaluation of antagonistic activity
Plant pathogenic fungi were kindly donated by Dr. Jairo Cristobal from the Laboratory of Plant Pathology at the Instituto Tecnológico de Conkal. Alternaria alternata and Curvularia lunata were originally isolated from Chamaedorea elegans, and in turn, Helminthosporium rostratum was isolated from Heliconia wagneriana. Antagonistic activity was evaluated by the inhibition of fungal colony growth in direct confrontation and by inhibition of conidial germination using the Bacillus culture filtrates.
Bioassays of direct confrontation were carried out in potato-dextrose-agar (PDA) medium. Briefly, discs of PDA (5 cm diameter) from axenic fungal culture were inoculated in the center of a 15 cm diameter Petri dish with PDA. Spore suspensions (1x107 CFU mL-1) of Bacillus strains were obtained in sterilized distilled water by scrapping with sterile scalpel blade 5-day-old bacterial cultures grown in NA. Bacterial spore suspensions were inoculated 2 cm from the margin of PDA discs that contained the fungal culture. All control fungal colonies were not confronted with the antagonists. Petri dishes with fungal cultures were incubated at 30°C. The inhibition of radial growth of fungal colony was daily evaluated and the percentage of mycelial growth inhibition was calculated with respect to the control colony according to Korsten and Jager (1995) as follows: GI(%)=((RC-RE)/RC)x100, where GI (%) is the percentage of mycelial growth inhibition, RC is the radius of the fungal colony in the control Petri dish, and RE is the radius of the fungal colony that was confronted with the antagonistic Bacillus.
For the bioassays of conidial germination, Bacillus strains were cultured in agar broth (BD, USA) for 72 h in an orbital shaker at 150 rpm and 30°C. Cultures were centrifuged at 7000 g for 10 min and the precipitate (vegetative cells and spores) was discarded. A previous study carried out in our laboratory showed that autoclaved culture filtrates from Bacillus spp. revealed antifungal activity on Colletotrichum gloeosporioides. Thus, in this study, filtered free-cell culture were autoclaved at 120 °C for 15 min and cooled down before using. Culture filtrates were mixed with conidial suspensions of fungi (1:1 v/v) obtained from colony grown in PDA. Mixture of culture filtrate and conidial suspensions were then inoculated in a PDA plate and observed for 12 h for conidial germination using a light microscope (LEICA DM500®, USA) at 100x magnification. Conidia were counted as germinated when the germ tube had at least the length of the germinated conidia, according to Mahadtanapuk et al. (2007).
Extraction of DNA of Bacillus strains
All Bacillus strains were cultured in Brain Hearth Infusion Broth (BHI) (BIOXON, USA) in 10 mL tubes at 30 °C for 48 h. Cultures were centrifuged at 2500 g for 5 min and washed with saline solution (0.85 %). Pellet was collected and supernatant discarded. DNA extraction was carried out with the kit Master Pure® Gram Positive DNA purification (Epicentre Biotechnologies, USA). Briefly, pellet was suspended in 150 μL TE buffer (Tris-EDTA), then added with 1 μL of lisozime ready lyse and incubated at 37°C for 30 min. Subsequently, 159 μL of proteinase K was added and incubated at 65°C for 15 min; tubes were agitated every 5 min. Then, tubes were maintained for 2 min at 37 °C, followed by 5 min in ice, to cool down. Samples were precipitated in a 175 μL of MPC (protein precipitation reagent) solution, agitated vigorously and centrifuged at 10 000 g for 10 min. The supernatant was separated and mixed with 1 μL RNase in a new tube. Samples were incubated at 37 °C for 30 min and 500 μL isopropanol was added. Tubes were centrifuged at 10000 x g for 10 min, the supernatant eliminated and the pellet remixed to suspension in 500 μL of ethanol (ETOH 70 %). Tubes were again centrifuged at 13000 x g for 1 min, supernatant eliminated and the pellet remixed to suspension in 35 μL of TE buffer (10 mM Tris-HCl and 1 mM EDTA Na2, pH 8.0; PROMEGA Corp., USA). The DNA was kept in a freezer at -20°C. DNA quality was confirmed by agarose gel electrophoresis 1.2 % (87 V for 60 min). Gel was stained with ethidium bromide (0.5 μg mL-1) for 30 min. Image was taken using a photo documentation system (Canon-Biotop, USA).
Polymerase Chain Reaction (PCR) analysis for molecular identification
For molecular identification of the strains, gen amplification by polymerase chain reaction (PCR) was carried out using the partial genes 16S rRNA, species-specific rpoB and gyrA. The methodology for each case was as follows:
For PCR analysis of 16S rRNA gene, specific primers for the internal portion of the “B. subtilis group” 16S rRNA, Bsub5F (5’-AAG TCG AGC GGA CAG ATG G-3’) and Bsub3R (5’CCA GTT CCA ATG ACC CTC CCC-3’) were used (Wattiau et al., 2001; Geetha et al., 2007). The reaction mixtures (25 μL) consisted of 12.5 μL master mix 1X (PROMEGA Corp., USA), 1 μL of the primer (0.4 mM) (IDT Technologies, USA), 9.5 μL DNAse-free water and 1 μL genomic DNA. The PCR program consisted of denaturation at 94°C for 12 min, followed by 30 cycles of denaturation at 95°C for 30 s, annealing at 65°C for 2 min, extension at 72°C for 2 min and, a final extension at 72°C for 7 min.
For PCR analysis of rpoB gene specific primers corresponding to B. subtilis position 6-585; rpoB-f (5’-AGG TCA ACT AGT TCA GTA TGG AC-3’) and rpoB-r (5’-AAG AAC CGT AAC CGG CAA CTT-3’) were used (De Clerck et al., 2004; Geetha and Manonmani, 2010). The reaction mix (25 μL) consisted of 12.5 μL master mix 1X (PROMEGA Corp., USA), 0.5 μL primer (0.2 mM) (IDT Technologies, USA), 10.5 μL DNAase-free water and 1 μL genomic DNA. The PCR program consisted of denaturation at 94°C for 2 min, followed by 40 cycles of denaturation at 94°C for 30 s, alignments at 51°C for 45 sec, extension at 68°C for 50 min and, final extension at 68°C for 90 sec.
For PCR analysis gyrA gene, specific primers corresponding to B. subtilis, position 43-1070; gryA-f (5’-CAG TCA GGA AAT GCG TAC GTC CTT-3’) and gyrA-r (5’CAA GGT AAT GCT CCA GGC ATT GCT-3’) were used (De Clerck et al., 2004; Geetha and Manonmani, 2008). The PCR reaction mixture (25 μL) consisted of 12.5 μL master mix 1X (PROMEGA, Corp., USA), 0.5 μL primer (10 mM) (IDT Technologies, USA), 10.5 μL DNAse-free water and 1 μL genomic DNA. The PCR program consisted of denaturation at 94°C for 2 min, 40 cycles denaturation at 94°C for 30 s, alignment at 51°C for 45 s, extension at 68°C for 50 min and, a final extension at 68°C for 10 min.
The resulted amplicons were visualized in agarose gel 1.2 %, purified with the kit Qiagen QIAquick (Qiagen Corp., USA) and sequenced in both directions using the corresponding primers (Bioscience, San Diego, CA. USA).
Phylogenetic analysis
Gene sequences were edited and aligned with the software BioEdit version 7.1.3.0 (Hall, 1999). To obtain the identity, the sequences were compared with those of the database from GenBank (National Center for Biotechnology Information), using the program BLASTN (Nucleotid Basic Local Alignment Search Tool). All sequences were deposited in the GenBank.
To study the relationship of the isolates with other members of the genus Bacillus, sequences of the regions used for each gene were obtained from GenBank and multiple alignments were carried out in the program CLUSTALW (Larkin et al., 2007). The evolutionary model for each gene was estimated and the analyses of Neighbor-Joining with Bootstrap of 1000 replicates were carried out in the program MEGA (Molecular Evolutionary Genetics Analysis) 5 (Tamura et al., 2011).
A matrix of partitions, with the sequences of the three analyzed regions, was built. The homogeneity test suggested that the three regions show a similar phylogenetic signal; therefore, a combined of such partitions may be built. Bacillus sonorensis as external group was used. The analysis of Neighbor-Joining was carried out with analysis Bootstrap of 1000 replicates. A matrix of evolutionary distances was built using the program MEGA 5 to determine the highest evolutionary rate of the analyzed region.
Data analysis
Experimental data were statistically analyzed using ANOVA with a completely randomized design. The Tukey test was used to determine significant differences among means (p≤0.05), using SAS 9.1. Unless otherwise indicated, all measurements were carried out in triplicate. Prior to run, data in percent were transformed to arcsin [y=arcsin(sqrt x/100)].
Results and discussion
Antagonistic activity of the Bacillus strains on three plant pathogenic fungi
The antagonistic activity of the strains by direct confrontation and by the use of the cell-free culture filtrate was evaluated in three plant pathogenic fungi: Alternaria alternata, Helminthosporium rostratum and Curvularia lunata. The strains used in this were molecularly identified as B. subtilis subsp. inaquosorum and B. subtilis subsp. subtilis. The results showed that the percentage of mycelial growth inhibition ranged from 32 to 78 % (Table 1). Moreover, the strain CBK36 presented the highest activity, causing more than 60 % inhibition of mycelial growth among the three evaluated fungi. In contrast, the strain CBMT51 showed the lowest effect, less than 40 % inhibition of colony growth in two of the tested fungi (Table 1).
Table 1 Effects of Bacillus strains on the percentage of mycelial growth inhibition of three plant pathogenic fungi.

Mean values (± standard errors) in a column followed by different letters are significantly different (Tukey; p≤0.05). CV(%): Coefficient of variation, HSD: Tukey’s Honest Significant Differences
The antagonistic activity of B. subtilis on plant pathogenic fungi is reported in literature. In vitro effects, specifically on mycelial growth and conidial germination are reported. In vivo effects are also reported, particularly on incidence and severity of foliar spots and blights, postharvest fruit rots and root rots (Chaurasia et al., 2005; Guillén-Cruz et al., 2006; Zongzheng et al., 2009; Basurto-Cardena et al., 2012).
The effect of culture filtrates on conidial germination of the fungi showed significant differences (p≤0.05). Culture filtrate of the strains CBCK36, CBMN22 and CBRF24 caused up to 37 % inhibition of conidial germination in at least two of the evaluated fungi. The culture filtrates of the strains CBCC2 and CBCK47 caused up to 46 % inhibition of conidial germination in A. alternata, but their effects on the other two fungi were lower (Table 2).
Table 2 Effect of the Bacillus culture filtrates on the inhibition of conidial germination in three plant pathogenic fungi.

Mean values (± standard errors) in a column followed by different letters are significantly different (Tukey; p≤0.05). CV (%)=Coefficient of variation; HSD = Tukey’s Honest Significant Differences
Moreover, the culture filtrate of this strain showed also one of the highest effects on conidial germination of the evaluated fungi, which suggest that part of its activity is mediated by the production of antifungal compounds. Culture filtrates of B. subtilis have suppressed the mycelial growth as well as the conidial germination of plant pathogenic fungi (Zongzheng et al., 2009; Islam et al., 2012). Notice that particularly the culture filtrates of the strains CBCK36, CBCC2 and CBCK47 showed more than 30 % inhibition of conidial germination at least in one of the tested fungi. As these culture filtrates were autoclaved prior to use, the antifungal compounds responsible for the effects were heat resistant. The identities of these compounds were not determined in this study, but we might hypothesize that heat resistant lipopeptides are likely involved. Heat resistant antifungal lipopeptides, like iturins, were extracted from the culture media of various strains of B. subtilis (Rezuanul et al., 2012). Indeed, culture filtrates containing heat resistant antifungal compounds were used to suppress the incidence and severity of fungal disease in vivo (Cubeta et al., 1985).
PCR amplification and phylogenetic analysis
To confirm the identity of the Bacillus isolates, sequence of partial regions of the 16S rRNA, gyrA and rpoB genes were obtained by PCR. Amplification of the hyper variable region of 16S rRNA, species-specific gyrA and rpoB genes of the seven Bacillus strains yielded amplicons of the 535 bp, 960-1005 bp and 546 bp, respectively (Figure 1).
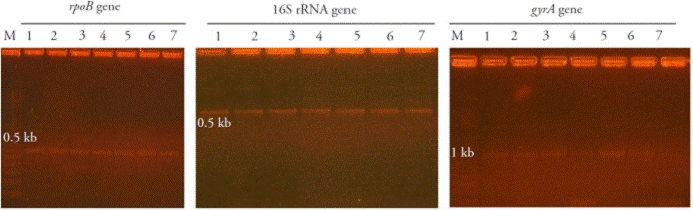
Figure 1 Agarose gel (1.2 %) electrophoresis of the PCR amplification of rpoB DNA, 16S rRNA and gyrA DNA genes of seven native Bacillus subtilis. Lane M: 1 kb ladder Promega, 1: CBCC2, 2: CBMT2, 3: CBMN22, 4: CBRF24, 5: CBCK36, 6: CBCK47 and 7: CBMT51.
On the basis of this analysis all the isolates were placed taxonomically as B. subtilis subsp. inaquosorum and B. subtilis subsp. subtilis on phylogenetic trees. Chun and Bae (2000) showed the use of the rpoB gene sequences in Bacillus. Later, Geetha and Manonmani (2010) also showed the utility of this gene sequence to identify the isolate B. subtilis subsp. subtilis VCRC B471. The protein rpoB was also used to infer relationships among archaea orders and Gram positive and Gram-negative bacteria (Matte-Tailliez et al., 2002; Morse et al., 2002).
The highest BLASTN identity of each of the strain was confirmed and also the genus identity of Bacillus subtilis group (Table 3). The gene sequences 16S rRNA, rpoB and gyrA-based PCR had values in a range of 95 to 100 %, similar to those deposited in Genbank (Table 3).
Table 3 Maximum identity values (%) of identified Bacillus strains based on the analysis of 16S rRNA, gyrA and rpoB genes.

The amplicons obtained for regions 16S rRNA, rpoB and gyrA were 535, 546 and 953 bp, respectively. The Neighbor-Joining analyses of each of the regions (Figures 2, 3 and 4) and the total evidence (Figure 5) showed that the isolates were separated into two clades. In all analyses, the isolates CBRF24 and CBMT2 were grouped in a different clade. Using the 16S rRNA region, the strains CBMT2, CBMN22, CBCK36, CBMT51 and CBCK47 formed a clade (Figure 2), with sequences of B. subtilis taken from GenBank. These isolates formed a clade with stand of 100 with B. subtilis subsp. subtilis; while with the analysis performed using the regions gyrA and rpoB, the mentioned strains formed a clade with B. subtilis subsp. inaquosorum.
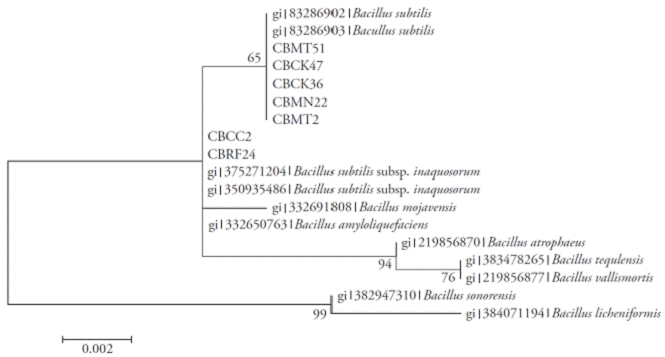
Figure 2 Neighbor-joining tree with bootstrap values based on partial sequences of the 16S rRNA gene. Analysis made with a nucleotide substitution model of Jukes-Cantor.
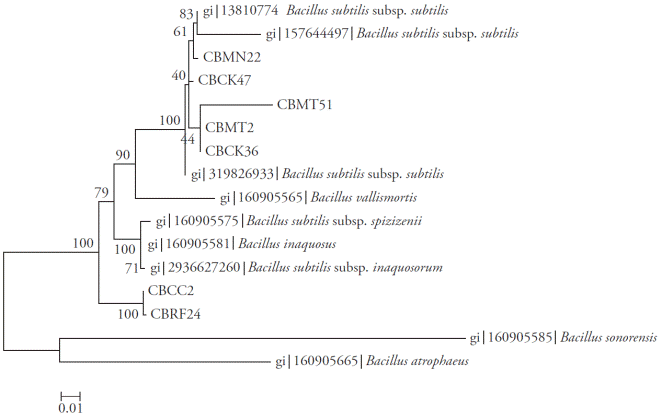
Figure 3 Neighbor-Joining tree with bootstrap values based on partial sequences of the gyrA gene. Analysis made with a nucleotide substitution model of Tamura-Nei with discrete gamma distribution.

Figure 4 Neighbor-Joining tree with bootstrap values based on partial sequences rpoB gene. Analysis made with a nucleotide substitution model of Tamura-3 parameter using discrete gamma distribution.
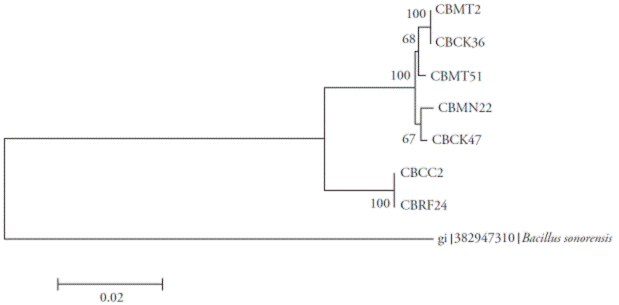
Figure 5 Neighbor-Joining tree with bootstrap values based on partial sequences of the 16S rRNA, gyrA and rpoB genes. Analysis made with a nucleotide substitution model of Tamura-3 parameter using discrete gamma distribution.
The analysis of matrix partitions separated the isolates into two distinct clades, forming a clade with CBRF24 and CBMT2, completely independent of the other strains (Figure 5). Amplification, sequencing and sequence analysis of 16S rRNA, rpoB and gyrA genes for molecular identification of Bacillus species are reported only in separate studies (Geetha et al., 2007; Geetha and Manonmani, 2008; Geetha and Manonmani, 2010). In our research, however, the first sequence analysis of 16S rRNA, gyrA and rpoB genes to locate taxonomically seven native strains of B. subtilis was successfully integrated. This molecular technique allowed us to infer the species of Bacillus and the existence of two new subspecies. Although 16S rRNA gene sequence was very useful for the determination of bacterial species, in the genus Bacillus some problems have arisen (Wattiau et al., 2001; Janda et al., 2007).
Evolutionary distance matrices
The region that showed lower evolutionary distances was the 16S rRNA (from zero to 0.002) followed by the rpoB gene (up to 0.004). In contrast, the gyrA gene showed the highest evolutionary rate (up to 0.09), being even higher than that obtained from the matrix partitions, which includes information from the three regions analyzed (0.034 maximum).
The greatest distances were observed between the isolates CBRF24 and CBMT2. Thus, for the 16S rRNA, the proper determination of the B. subtilis subspecies could be difficult. In contrast, for the gyrA gene, its higher evolutionary rate allowed to allocate B. subtilis at the subspecies level. In particular, the comparative analysis of gyrA sequences proved to be robust for rapid classification and identification of B. subtilis and related taxa (Chun and Bae, 2000), as shown in our study. The characterization of all strains at the subspecies level was not possible with the data obtained. The strains CBMT2 and CBRF24 might eventually form new subspecies, since the rest of the strains were grouped into clades with different subspecies.
Conclusions
The native Bacillus strains from the Yucatan Peninsula, Mexico, were identified as Bacillus subtilis subsp. inaquosorum and Bacillus subtilis subsp. subtilis by the analysis of gene: 16S rRNA, gyrA and rpoB. Seven strains showed antagonistic activity on mycelial growth inhibition of the plant pathogenic fungi Alternaria alternata, Helminthosporium rostratum and Curvularia lunata. The cell-free culture filtrates of CBCK36, CBCC2 and CBCK47 strains showed inhibition of mycelial growth and caused different degree of inhibition of conidial germination in the evaluated plant pathogenic fungi. The strain CBCK36 showed the highest activity on inhibition of fungal mycelial growth.
Literature Cited
Adesemoye, A. O., M. Obini, and E. O. Ugoji. 2008. Comparison of plant growth promotion with Pseudomonas aeruginosa and Bacillus subtilis in three vegetables. Braz. J. Microbiol. 36: 423-426. [ Links ]
Agrios, G. N. 2005. Plant Pathology. Elsevier Academic Press, San Diego, California, USA. 903 p. [ Links ]
Ajao, A. T., G. B. Adebayo, and S. E. Yakubu. 2011. Bioremediation of Textile Industrial Effluent using mixed culture of Pseudomonas aeruginosa and Bacillus subtilis immobilized on agar-agar in a Bioreactor. J. Microbiol. Biotech. Res. 1: 50-56. [ Links ]
Augusto-Costa, A. C., and F. Pereira-Duta. 2001. Bioaccumulation of copper, zinc, cadmium and lead by Bacillus SP., Bacillus cereus, Bacillus speaerecus and Bacillus subtillus. Braz. J. Microbiol. 32:1-5. [ Links ]
Basurto-Cadena, M. G. L., M. Vázquez-Arista, J. GarcíaJiménez, R. Salcedo Hernández, D. K. Bideshi, and J. E. Barboza-Corona. 2012. Isolation of a new Mexican strain of Bacillus subtilis with antifungal and antibacterial activities. Scientific World J. doi:10.1100/2012/384978, 7 p. [ Links ]
Blasco, C., Y. Picó., J. Mañes, and G. Font. 2002. Determination of fungicide residues in fruits and vegetables by liquid chromatography-atmospheric pressure chemical ionization mass spectrometry. J. Chomatogr. 947: 227-235. [ Links ]
Chaurasia, B., A. Pandey., L. M. S. Palni., P. Trivedi., B. Kumar, and N. Colvin. 2005. Diffusible and volatile compounds produced by an antagonistic Bacillus subtilis strain cause structural deformations in pathogenic fungi in vitro. Microbiol. Res. 160: 75-81. [ Links ]
Cubeta, M. A., G. L. Hartman., and J. B. Sinclair. 1985. Interaction between Bacillus subtilis and fungi associated with soybeans seeds. Plant Dis. 69:506-509. [ Links ]
Cubitto, M. A., A. C. Morán., M. Commendatore, M. N. Chiarello., M. D. Baldini, and F. Siñeriz. 2004. Effects of Bacillus subtilis O9 biosurfactant on the bioremediation of crude oil-polluted soils. Biodegradation 15: 281-287. [ Links ]
Chun, J., and K. S. Bae. 2000. Phylogenetic analysis of Bacillus subtilis and related taxa based on partial gyrA gene sequences. Anton. Leeuw. 78: 123-127. [ Links ]
De Clerck, E., T. Vanhouette., T. Hebb., J. Geerinck, and P. De Vos. 2004. Isolation, characterization and identification of bacterial contaminants in semifinal gelatin extracts. Appl. Environ. Microb. 70: 3664-3672. [ Links ]
De Waard, M. A., S. G. Georgopoulas, D. W., Hollomon, H. Ishii, P. Leroux, N. M. Ragsdale, and F. J. Schwinn. 1993. Chemical control of plant diseases: problems and prospects. Annu. Rev. Phytopathol. 31: 403-421. [ Links ]
Fickers, P. 2012. Antibiotic compounds from Bacillus: Why are they so amazing? Am. J. Biochem. Biotechnol. 8: 40-46. [ Links ]
Geetha, I., G. Prabakaran., K. P. Paily, and A. M. Manonmani. 2007. Characterisation of three mosquitocidal Bacillus strains isolated from mangrove forest. Biol. Control. 42: 3440. [ Links ]
Geetha, I., and A. M. Manonmani. 2001. Mosquito pupicidal toxin production by Bacillus subtilis subsp. subtilis. Biol. Control. 44: 242-247. [ Links ]
Geetha, I., andA. M. Manonmani. 2010. Surfactin: a novel mosquitocidal biosurfactant produced by Bacillus subtilis ssp. subtilis (VCRC B471) and influence of abiotic factors on its pupicidal efficacy. Lett. Appl. Microbiol. 51: 406-412. [ Links ]
González-Fernández, R., E. Prats, and J. Jorrín-Novo. 2010. Proteomics of plant pathogenic fungi. Journal of Biomedicine and Biotechnology. doi:10.1155/2010/932527, 36 p. [ Links ]
Guillén-Cruz, R., F. D. Hernández-Castillo., G. Gallegos-Morales., R. Rodríguez-Herrera., C. N. Aguilar-González., E. Y. Padrón-Corral, y M. H. Reyes-Valdés. 2006. Bacillus spp. como biocontrol en un suelo infestado con Fusarium spp., Rhizoctonia solani Kühn y Phytophthora capsici Leonian y su efecto en el desarrollo y rendimiento1 del cultivo de chile (Capsicum annuum L.). Rev. Mex. Fitopatol. 24: 105114. [ Links ]
Hall, T. A. 1999. BioEdit: a user-friendly biological sequence alignment editor and analysis program for Windows 95/98/ NT. Nucl. Acid. S. 41: 95-98. [ Links ]
Harwood, C. R. 1992. Bacillus subtilis and its relatives: molecular biological and industrial workhorses. Trends Biotechnol. 10: 247-56. [ Links ]
Islam, M. R., Y. T. Jeong., Y. S. Lee, and C. H. Song. 2012. Isolation and identification of antifungal compounds from Bacillus subtilis C9 inhibiting the growth of plant pathogenic fungi. Mycobiology 40: 59-66. [ Links ]
Janda, J. M., L. Sharon, and Abbott, S. L. 2007. 16S rRNA Gene Sequencing for Bacterial Identification in the Diagnostic Laboratory: Pluses, Perils, and Pitfalls. J. Clin. Microbiol. 45: 2761-2764. [ Links ]
Janiciewickz, W.J., Leverentz, B., Conway, W.S., Saftner, R.A., Reed, A.N., and Camp, M.J. 2003. Control of bitter rot and blue mold of apples integrating heat and antagonistic treatments on 1-MCP treated fruits stored under controlled atmosphere conditions. Postharvest Biology and Technology 29: 129-143. [ Links ]
Knight, S. C., V. M. Anthony., A. M. Brady., A. J. Greenland., S. P. Heaney., D. C. Murray., K. A. Powell., M. A. Schulz., C. A. Spinks., P. A. Worthington, and D. Youle. 1997. Rationale and perspectives on the development of fungicides. Annu. Rev. Phytopathol. 35: 349-372. [ Links ]
Korsten, L., and E. E. Jager. 1995. Mode of action of Bacillus subtilis for control of avocado postharvest pathogens. SAAGA Yearbook 18: 124-130. [ Links ]
Kumar D. J. M., C. L. Poovai., P. Kumar, Y. S. Saroja., A. Manimaran, and P. T. Kalaichelvan. 2012. Optimization of Bacillus cereus MRK1 cellulase production and its Biostoning activity. Der Pharmacia Lettre 4: 881-888. [ Links ]
Larkin, M. A., G. Blackshields., N. P. Brown, R. Chenna., P. A. Mcgettigan., H. Mcwilliam., F. Valentin., I. M. Wallace., A. Wilm., R. Lopez., J. D. Thompson., T. J. Gibson, and D. G. Higgins. 2007. ClustalW and ClustalX version 2. Bioinformatics 23: 2947-2948. [ Links ]
Lugtenberg, B., and F. Kamilova. 2009. Plant-growth-promoting rhizobacteria. Annu. Rev. Microbiol. 63: 541-556. [ Links ]
Mahadtanapuk, S., M. Sanguansermsri., R. W. Cutler., V. Sardsud, and S. Anuntalabhochai. 2007. Control of Anthracnose caused by Colletotrichum musae on Curcuma alismatifolia Gagnep. using antagonistic Bacillus spp. ARPN. 2: 54-61. [ Links ]
Matte-Tailliez, O., C. Brochier., P. Forterre, and H. Philippe. 2002. Archaeal phylogeny based on ribosomal proteins. Mol. Biol. Evol. 19: 631-639. [ Links ]
Morse, R., K. O’hanlon, and M. D. Collins. 2002. Phylogenetic, amino acid content and indel analyses of the beta subunit of DNA-dependent RNA polymerase of grampositive and gram-negative bacteria. IJSEM 52: 1477-1484. [ Links ]
Pal, K. K., and B. M. Gardener. 2006. Biological control of plant pathogens. The plant health instructor. Biological control. DOI: 10.1094/PHI-A-2006-1117-02. [ Links ]
Podile, A. R., and V. D. V. Laxmi. 1998. Seed bacterization with Bacillus subtilis AF1 increases phenylalanine ammonia lyase and reduces the incidence of fusarial wilt in pigeonpea. J. Phytopathol. 146: 255-259. [ Links ]
Price, N. P. J., A. P. Rooney., J. L. Swezey., E. Perry, and F. M. Cohan. 2007. Mass spectroscopic analysis of lipopeptide for Bacillus strains isolated from diverse geographical locations. FEMS Microbiol. Lett. 271: 83-89. [ Links ]
Rezuanul, I., Y. T. Jeong., Y. S. Lee, and C. H. Song. 2012. Isolation and Identification of Antifungal Compounds from Bacillus subtilis C9 Inhibiting the Growth of Plant Pathogenic Fungi. Mycobiology 40: 59-66. [ Links ]
Rooney, A. P., N. P. J. Price., C. Ehrhardt., J. L. Swezey, and J. D. Bannan. 2009. Phylogeny and molecular taxonomy of the Bacillus subtilis complex and description of Bacillus subtilis subsp. inaquosorum subsp. nov. Int. J. Syst. Evol. Micr. 59: 2429-2436. [ Links ]
Schisler, D. A., P. J. Slininger., R. W. Behle, and M. A. Jackson. 2004. Formulation of Bacillus spp. for biological control of plant disease. Phytopathology 94: 1267-1271. [ Links ]
Tamura, K., D. Peterson., N. Peterson., G. Stecher., M. Nei, and S. Kumar. 2011. MEGA5: Molecular Evolutionary Genetics Analysis using Maximum Likelihood, Evolutionary Distance, and Maximum Parsimony Methods. Mol. Biol. Evol. 28: 2731-2739. [ Links ]
Van Loon L. C. 2007. Plant responses to plant growth-promoting rhizobacteria. Eur. J. Plant Pathol. 119: 243-254. [ Links ]
Wattiau, P., M. E. Renard., P. Ledent., V. Debois., G. Blackman, and S. N. Agathos. 2001. A PCR test to identify Bacillus subtilis and closely related species and its application to the monitoring of wastewater biotreatment. Appl. Microbiol. Biotechnol. 56: 816-819. [ Links ]
Waard, M. A., A. C. Andrade., K. Hayashi., H. J. Schoonbeek., I. Stergiopoulos, and L. H. Zwiers. 2006. Impact of fungal drug transporters on fungicide sensitivity, multidrug resistance and virulence. Pest Manag. Sci. 62: 195-207. [ Links ]
Zongzheng, Y., L. Xin., L. Zhong., P. Jinzhao., Q. Jin, and Y. Wenyan. 2009. Effect of Bacillus Subtilis SY1 on antifungal activity and plant growth. IJABE 2: 55-61. [ Links ]
Received: September 2014; Accepted: November 2015