Introduction
Global warming, in recent years, has become one of the most relevant scientific, political, and economic issues on the entire planet. According to the Intergovernmental Panel on Climate Change [IPCC] (Metz et al., 2007), the period between 1995 and 2006 seems to have been one of the warmest ones recorded since 1850. Relevant processes such as ocean temperature alteration, polar ice caps decrease, shortening cold seasons, permafrost extension decrease, and rising sea levels provide clear evidence that the world is warming. Slowing down and limiting climate change requires, in the long term, a great effort at the international level. The most important current path is the United Nations Agreement on Climate Change, which 189 countries have ratified. However, taking preventive measures at the national, regional, and local levels is also essential. Industrialized countries have made some progress, although limited, in stopping greenhouse gas (GHG) emissions since 1990. During this period, an urgent need to design specific policies, such as emission permits, taxes on energy and the environment, voluntary measures in the industrial sector, regulatory policies, and research on programs aimed at mitigating GHG (García Fernández, 2011).
Carbon measurement and monitoring in high mountain ecosystems have become essential research topics due to climate change. Particularly, carbon measurement and monitoring are vital to have empirical data for negotiations to reduce greenhouse gas emissions associated with deforestation and land-use change (Goetz & Dubayah, 2011). Likewise, the records of carbon in moorland contribute to understanding and improving the quantification of carbon sinks and flows in these environments (Hall et al., 2011). Although high mountain tropical ecosystems are distinguished by their provision of hydrological services (Jiménez-García & Peterson, 2019) and biodiversity protection, their role in carbon storage is less known. Thus, studies on the quantification of carbon stored in the moorlands will highlight this ecosystem's role in international climate change policy. Also, carbon credits generated from this ecosystem can be offered in international markets. The benefits of carbon trading could be used to create programs and projects focused on the conservation of the moorland ecosystems as a strategy to slow down global warming. In return, communities nearby or within these areas will benefit from protecting and conserving these ecosystems (Ward et al., 2016).
The Chimborazo Fauna Production Reserve (RPFCH, by its Spanish acronym) was created through Ministerial Agreement No. 437 on October 26, 1987 and published in Official Register No. 806 on November 9, 1987. The RPFCH, located in Chimborazo, Tungurahua, and Bolívar provinces, has an extension of 58560 ha. The altitudinal gradient ranges from 3800 m to 6310 m (at the summit of Chimborazo volcano), and it has a cold climate with temperatures ranging from 0 °C to 10 °C. However, despite the altitude of this area, it is home to diverse flora and fauna, unique on the planet (Ministerio del Ambiente del Ecuador [MAE], 2014).
Quantifying the carbon stock in the soil of the eight ecosystems of the RPFCH by measuring and monitoring the carbon in high Andean areas has become an important research topic in recent years due to climate change. Therefore, it is vital to quantify the amount of carbon stored in this area to have an empirical database to monitor, assess, make decisions, and deal with negotiations to reduce greenhouse gas emissions, especially those emissions that are intrinsically associated with activities such as deforestation, scrubland burning, mining, flora fragmentation and the introduction of exotic species (Goetz & Dubayah, 2011). In addition, studies of carbon stocks in high Andean ecosystems help to gain knowledge and have quantitative values of carbon sinks and flows in these environments (Hall et al., 2011).
The main objectives envisaged in the creation of the area mainly focus on: firstly, maintaining the resources of the moorland ecosystems, developing and taking care of the habitat of the Andean native camelids (vicuñas, llamas, and alpacas), based on the respective ecological parameters, for breeding and promoting these valuable species linked to our cultural identity. Secondly, establishing the infrastructure and necessary services for tourism and moorland research, especially on native camelids, to obtain knowledge and technologies for breeding and promoting species included in this zoological group. Finally, the last main objective is to improve the living standard of people in the area by providing them support on camelid handling (MAE, 2014).
Despite the importance of this Andean ecosystem, its carbon storage role is not well known. Therefore, having reliable data on the carbon stored in the RPFCH will contribute positively to highlighting the role that these eight ecosystems have in the policies of the Ministry of Environment as well as in international policies regarding climate change issues. On the other hand, it will help propose new economic incentives to support better natural resources management within the reserve (Ward et al., 2016).
The general objective of protected areas worldwide is to manage biological resources to keep the biodiversity and the environmental services they provide in good condition. For example, the typical vegetation of high Andean ecosystems contributes to carbon storage and water regulation. Additionally, they prevent greenhouse gas emissions caused by deforestation, livestock, and degradation (Busch & Grantham, 2013).
Moorland ecosystems and their carbon storage Soil organic carbon stock refers to the total amount of carbon in the form of organic matter derived from animal and plant remains in the soil. The measure of organic carbon in the soil is an indicator of soil quality; that is why its variation may have implications for environmental processes, mainly in the erosion and flow of greenhouse gases (Stolbovoy et al., 2007).
Biologically, high Andean ecosystems are very diverse and provide countless environmental services. They store large amounts of carbon because they are influenced by low temperatures, reducing the rate of decomposition of organic matter since this process becomes slow. Although the availability of all aerial biomass is not abundant, the organic matter (OM) is accumulated in the soil and can reach up to 60 kg/m2 of carbon; for this reason, it is among the soils with the highest carbon reserves in the world (Castañeda & Montes, 2017).
According to Fehse et al. (2002), to mitigate the greenhouse effect caused by the increasing concentration of carbon dioxide (CO2) in Earth's atmosphere, it is essential not to alter the main sites where this element is stored. Thus, the soils of moor ecosystems, dominated by low temperatures, cause this habitat to have reduced rates of mineralization and nutrient recycling. In turn, the reduced rates of mineralization and nutrient recycling favor a slow but continuous net absorption of atmospheric CO2, which accumulates as part of the organic matter in their soils with histosolic characteristics.
Concentrations of CO2, methane (CH4), and nitrous oxide (N2O) in the atmosphere increased by anthropic activities such as changes in land use are leading causes of global warming. Unfortunately, this global warming is exceeding the levels that vegetationvegetation species can tolerate, especially in high Andean ecosystems where these species coexist in a tight ecological balance. Consequently, this change affects the composition and structure of the vegetation masses and, therefore, the soil that maintains them because there is an intimate relationship between vegetation - soil - MO and OC (Metz et al., 2007).
Floristic diversity plays a vital role in carbon capture and storage. According to a study conducted to quantify the organic carbon in the moors of Ecuador's Yacurí National Park (PNY), in 2014, at a depth of 30 cm, the bushy moorland presented values of 159.05 Mg/ha, and the herbaceous moor presented values of 116.18 Mg/ha. The carbon contents fixed in the soil at a depth of 60 cm were 537.06 Mg/ha in the herbaceous moorland and 471.59 Mg/ha in the soils of the shrub moorland, confirming that these ecosystems are actual carbon sinks (Ayala et al., 2014). In any case, an average carbon capture capacity of 108 Mg/ha in the first 30 cm depth is present in the soils of the moorlands. Such carbon capture values are common in high mountain areas due to the organic characteristics of taxonomically young soils, such as histosols, andisols, and inceptisols (Fernández Pérez et al., 2019).
Although vegetation is a determining factor when quantifying the organic carbon content since it is responsible for setting it in the soil, this is not proportional to the carbon content in the aerial biomass due to the type of species present in each vegetation formation. In addition, not all vegetation sets carbon in equal proportions. For example, the Chimborazo Fauna Production Reserve is home to eight plant formations or ecosystems, each with representative species and typical characteristics of Andean environments. From these ecosystems, the evergreen forest of the moorland stands out, which is the forest that is located at the highest altitude in Ecuador. For this reason, the tree species of this ecosystem have modified their growth to adapt to the environment, showing themselves in a squatted way in association with bushes, which contributes to a more significant amount of organic matter and, therefore, a greater amount of OC.
In a study carried out in Colombian Andean forests, Phillips et al. (2011) report average carbon values of 128 Mg/ha in mature and squatted forests with Polylepis genera species and in a range of 48.1 Mg/ha to 129.4 Mg/ha with different altitudinal gradients. Similarly, Köhl et al. (2015) report 122 Mg/ha in studies conducted in forests of South America. In Ecuador, Fehse et al. (2002) estimate between 88 Mg/ha and 183 Mg/ha stored in secondary forests with species of Alnus and Polylepis genera, 30 years and 45 years old, respectively. They are typical of high Andean areas and contain carbon in their aerial biomass but in smaller quantities than other ecosystems at lower altitudes. However, these habitats are degenerating due to global warming, which also affects carbon storage (Etter et al., 2006).
It is estimated that high Andean ecosystems in Ecuador and Latin American countries store more carbon than other terrestrial environments in the world, at an average of 3.2 Gt of CO2 per year, which advocates the importance of maintaining and conserving this environment. However, as the degradation of these ecosystems increases, it is estimated that carbon capture values of 9 Gg to 23 Gg of CO2 per year would be lost worldwide. Therefore, conservation efforts should be prioritized since they are endemic species and because of the countless environmental services they provide and from which human communities benefit (Segura et al., 2019). In this context, this research work aims to answer the following question: What is the organic carbon content in the soil of the eight vegetation formations of the Chimborazo Fauna Production Reserve that can be offered as carbon bonds as a mechanism for moorland ecosystem conservation and climate change mitigation?
Objectives
To quantify the organic carbon content in the soil of the eight vegetation formations of the Chimborazo Fauna Production Reserve to offer carbon bonds as a mechanism for moorland ecosystem conservation and climate change mitigation.
Materials and methods
Study area
The RPFCH is part of Ecuador's National System of Protected Areas. Within this area are two contiguous snowy mountains: Chimborazo, with an altitude of 6310 m, the highest snow-capped mountain in the country, and Carihuayrazo, with an altitude of 5020 m. The reserve is located in the provinces of Chimborazo, Tungurahua, and Bolívar. One of the main reasons for declaring the reserve was the implementation of a South American Camelid Reintroduction Program. It is one of the country's most important protected areas because it has varied biodiversity and is home to countless endemic species of flora and fauna. In addition, this protected area provides several environmental services, including water regulation and carbon sinks (MAE, 2014).
The study area covers over 58560 ha, containing eight ecosystems according to their typology (Fig. 1): 1) evergreen bushland and grassland of the moorland 2563 ha, 2) evergreen forest of the moorland 362 ha, 3) grassland of the moorland 6274 ha, 4); upper high montane humid grassland of the moorland 16343 ha, 5) subnival humid grassland of the moorland 4163 ha; 6) flooded grassland of the moorland 765 ha; 7) subnival ultra-humid grassland of the moorland 11067 ha, and 8) evergreen subnival grassland and bushland of the moorland 6094 ha. The temperature in the reserve depends on the altitude. According to the Instituto Nacional de Metereología e Hidrológia [Inamhi] (2019), the temperatures here range from -0.11 °C, at the summit of Chimborazo and an average of 8.81 °C in the eastern and western foothills of the reserve. The rainfall here occurs in the form of snow or frost, depending on the area where it is recorded, with an annual average of 809 mm to 998 mm and 1300 mm in more humid areas.
Statistical analysis
To determine the sampling intensity, sample size, and the number of sample units, 500 m × 500 m2 cells were developed in each ecosystem (Table 1). For this purpose, Arc Gis 10.1 software was used, and employing Hawths Tools, Sampling Tools, and Create Vector Grid, 819 cells were built in the eight vegetation formations. Only those not in an ecotone ecological area were counted to avoid the edge effect during field data collection at a depth of 0.3 m.
Table 1 Determination of the number of samples per vegetation formation through optimal plots in Chimborazo Fauna Production Reserve, Ecuador.
Code | HsNnO1 | AsSnO1 | HsSnO2 | BsSnO1 | HsSnO3 | HsSnO4 | HsNnO2 | HsNnO3 |
N | 60 | 40 | 65 | 50 | 260 | 30 | 210 | 104 |
p | 0.5 | 0.5 | 0.5 | 0.5 | 0.5 | 0.5 | 0.5 | 0.5 |
q | 0.5 | 0.5 | 0.5 | 0.5 | 0.5 | 0.5 | 0.5 | 0.5 |
ai | 0.07 | 0.05 | 0.08 | 0.06 | 0.32 | 0.04 | 0.26 | 0.13 |
Σ num | 12285.0 | 8190 | 13308.75 | 102375 | 53235 | 6142.5 | 42997.5 | 21294 |
Σ den | 15000 | 10000 | 16250 | 12500 | 65000 | 7500 | 52500 | 26000 |
ni | 19.16 | 12.77 | 20.75 | 15.96 | 83.01 | 9.58 | 67.05 | 33.21 |
(n) redon | 20 | 13 | 21 | 16 | 84 | 10 | 68 | 34 |
HsNnO1: Subnival humid grassland of the moorland; AsSnO1: Evergreen bushland and grassland of the moorland; HsSnO2: Grassland of the moorland; BsSnO1: Evergreen forest of the moorland; HsSnO3: Upper high montane humid grassland of the moorland; HsSnO4: Flooded grassland of the moorland; HsNnO2: Subnival ultra-humid grassland of the moorland; HsNnO3: Evergreen subnival grassland and bushland of the moorland
Σ num: Sum of the numerator, Σ den: Sum of the denominator, nor: Number of sample units
For the statistical analysis, the criteria and formulas of the Ordering Manual of Andalucía hills were taken into consideration (De la Hoz Rodriguez et al., 2004), where the sampling intensity was determined under the following equation:
where:
i = sampling intensity
n = number of selected plots
a = plot expressed in hectares
A= total number of established parcels
The sample size was applied using the following equation:
where:
n = sample magnitude in number of units
t2 is obtained from the t-student table, and its value depends on the magnitude of the sample n
s2 = estimated population variance N = population size
The researchers worked with a 5% error and 95% certainty for the statistical analysis. For this, optimal plots were used where the approximate sample size estimated p with a limit of B for the estimation error, determining the number of points where the data would be collected for analysis for this purpose, the following equation was used:
where:
𝑎𝑖 = fraction of observations fixed to the stratum
𝑖, 𝑝𝑖 = population proportion for the stratum
Once the number of sample units was determined from equation 3, it was rounded to obtain the exact number of sites where data collection would occur.
The apparent density (Ad) was estimated in the field and from unaltered soil samples, using cylinders of 7 cm diameter and 6 cm high. Next, the factor proposed by Jean Dumas, known as dry combustion, was used to estimate the carbon content, which allowed to determine whether the total organic carbon in the soil (TOCS) was accurate (McCarty et al., 2002). Finally, the factor proposed by Van Bemmelen was used to convert the TOCS to organic matter (OM), indicating that 58% of the OM is composed of carbon. However, since this content derives from the average elemental composition of humic acids, it does not contemplate the composition of all organic substances in the soil. Nevertheless, many authors agree with this factor, which is very useful in determining the content of organic matter (Galantini et al., 1992). Additionally, C concentrations of 58% can be found in the humidified OM and up to 40-42% in young OM. It is important to consider that the proportion of humidified particles of OM changes with the soil texture (Cantú & Yánez, 2018).
For the analysis of the soil samples, the equipment was heated to a temperature of 950 °C. All the required parameters were inspected to verify that they were well-calibrated or within their range since these were previously defined when creating an analysis method. For some soils of the Andean region, the TOCS determination techniques of Walkley & Black, Loss on ignition (LOI), were compared to the dry combustion method of Dumas, taken as a reference. A high correlation was obtained among TOCS determination techniques (r2 = 0.98-0.99). The correction factor of 1.32 was verified, usually used in the Walkley & Black methodology to convert easily oxidizable carbon (EOC) into TOCS. It was observed that the slope of the relationship between OM determined by LOI and the TOCS determined by the Dumas method (1697) did not differ from the value of 1724 usually used for converting TOCS to OM. The Dumas methodology showed greater veracity and precision than the other techniques of TOC determination. Thus, to estimate the OC, the following equation was determined:
Next, the soil's total organic carbon content was calculated using the following formula.
where:
TOCS = total organic carbon in soil per area (Mg/ha)
TOC = total organic carbon (%)
Ad = apparent density (g/cm3)
Ds = depth of soil (m)
Results
Levels of total organic carbon in the soil at 30 cm in the Chimborazo Fauna Production Reserve With the results obtained from the organic carbon content in the laboratory, the results were systematized to visualize them in the ArcGIS software. Here, with the help of geoprocessing and data interpolation tools, a mask was created using 819 organic carbon data in the eight ecosystems that the Chimborazo Fauna Production Reserve possesses. Thus, a map was obtained where the different amounts or concentrations of carbon can be visualized throughout the study area and the quantification in each of the ecosystems. In this sense, four levels were considered: low (0 Mg/ha - 50 Mg/ha), medium (50 Mg/ha-150 Mg/ha), high (150 Mg/ha - 250 Mg/ha), and very high (250 Mg/ha - ˃300 Mg/ha) (Vela et al., 2009) (Fig. 2).
Soil with vegetation formations with very high TOCS content (250 Mg/ha - 300 Mg/ha)
The soils with very high OC contents correspond to two well-defined typologies. The first corresponds to the evergreen forest of the moorland (BsSN01) and the second to the grassland of the moorland (HsSn02). The contents of these two ecosystems respond to the presence of typical species, where the transferring of carbon from the vegetation biomass to the soil is greater. In this sense, the ecosystem with the highest OC within the reserve is BsSN01 with a value of 378.16 Mg/ha, representing 3.13% of the total fixed carbon. This vegetal formation is peculiar for having dense and squatted tree species, with heights of 5 m to 7 m, which due to the effect of the climate, grow in a crooked and branched form of a particular aspect. We find it in the form of patches to the southwest and northeast of the reserve, being these places the least exposed to wind and drying; on hillsides, at the bottom of valleys, and at the foot of cliff walls (Beltrán et al., 2009).
A few species dominate the tree stratum due to physiological limitations that prevent woody growth. The canopy is generally composed of Polylepis, Gynoxys, and Buddleja genera species. These forests’ dominance varies greatly, in some cases Polylepis or Gynoxys form monotypic units.Jørgensen and Ulloa (1994) and Vásquez et al. (2014) obtained similar results, where, studies of carbon were carried out in Polylepis forests at an average altitude gradient of 4200 m, obtaining results of 397 Mg/ha;also in Apolobamba Bolivia at an altitude of 6040 m, at 50 cm, a value of 210 Mg/ha was recorded (Muñoz et al., 2013).
On the other hand, the grassland of the moorland constitutes the second vegetal formation that fixes the most carbon. According to the laboratory analysis, soils of this ecosystem reached values of 246.15 Mg/ha, corresponding to 35.26% of the total carbon in the reserve. These results are because most of the territory is monopolized by the Calamagrostis intermedia and Agrostis perennans species, which occupy 75% of this vegetation typology. Also, due to their phenology, 25% of other species of the Calamagrostis, Agrostis, Festuca, Cortaderia, and Stipa genera help maintain OC in the soil (Gobierno Autónomo Descentralizado de la Provincia de Chimborazo [GADPCH] 2013). This exceeds what Morales et al. (2007) and Ramos et al. (2013) found in studies of OC in Guantiva La Rusia, Boyacá, where the scrubland soils at a depth of 0.15 cm reported a value of 144.93 Mg/ha. In addition, these soils presented high contents of organic matter and carbon, mainly in the first horizon. On the other hand, Urgiles et al. (2018) conducted studies in different land coverings, obtaining high values of 110.03 Mg/ha in scrubland areas, while for areas with minimum coverage, 95.03% of organic carbon was registered. These studies highlight the importance of the positive effect of coverage with native vegetation as confirmed by Africano et al. (2016). They obtained high OC values of 872.26 Mg/ha, in scrubland soils, in areas without disturbance. This indicates that the variability of the stored OC depends fundamentally on the non-alteration of these zones; in addition to other factors such as precipitation, temperature, altitude, slope, vegetation type, and microbial activity.
Soil with vegetation formations with high TOCS content (150 Mg/ha - 250 Mg/ha)
The soil of the evergreen bushland and grassland of the moorland (AsSnO1) contemplates a value of 167.06 Mg/ha, which corresponds to high in the scale, according to Vela et al. (2009), containing 9.78% of the carbon stored in the study area. This is because this ecosystem is located in an altitudinal gradient from 3300 m to 3900 m, where the number of species and its floristic richness increase, which positively influences the carbon storage on this site. In addition, this is the ecotone between the grassland of the moorland and the evergreen forest of the moorland, since Calamagrostis intermedia and Agrostis perennans with a brush shape and 1.20 m in height are also found in these areas. Also, in these areas, bushes mainly of the Baccharis, Gynoxis, Brachyotum, Escallonia, Hesperomeles, Miconia, Buddleja, Monnina, and Hypericum genera occur.
Soil with vegetation formations with medium TOCS content (50 Mg/ha - 150 Mg/ha)
The soils of the vegetation formations with medium levels reported in this study correspond to the Upper high montane humid grassland of the moorland (HsSnO3), flooded grassland of the moorland (HsSnO4), and evergreen subnival grassland and bushland of the moorland (HsNnO3) with 67. 31 Mg/ha; 67.68 Mg/ha and 79.74 Mg/ha of carbon, respectively. The first vegetation formation stores 25.12% of the total carbon in the reserve. The soil does not have much carbon content here, registering 67.31 Mg/ha. However, the extension of this ecosystem of 16343 ha when multiplied by the carbon content, represents ¼ of the (TOCS) of the reserve. This ecosystem is dominated by species of Stipa, Senecio, and Plantago genera, found in volcanic enclaves located at the bottom of glacial valleys, associated with rain shadow effects, as, for example, on the western flank of the Chimborazo volcano (Sklenár & Balslev, 2005). On the other hand, the flooded grassland of the moorland with 765 ha, the evergreen subnival grassland, and bushland of the moorland with an area of 6094 ha have almost similar values of OC content. The difference lies in the area of each one, representing between them 12% and 28% of the TOCS of the reserve.
However, it should be mentioned that this formation is the most affected because the populations are adjacent to this area due to the abundant presence of water; additionally, grazing and pasture sowing negatively influence carbon storage caused by land-use change. This fact coincides with the statements made by Suáres et al. (2016), who state that this ecosystem is considered the most important since it is where water is stored; therefore, it functions as a water regulator. They also mention that these soils have a high OC content due to the plants' increased productivity and low decomposition of the organic matter. The Antisana Ecological Reserve (REA, by its Spanish acronym) and the Cajas National Park (PNC, by its Spanish acronym) in Ecuador have the highest organic carbon values recorded in high-Andean studies. In the same way, it is manifested that livestock, burning of grass, agriculture, and overgrazing reduced carbon content versus other well-preserved places.
Soil with vegetation formations with low TOCS content (0 Mg/ha - 50 Mg/ha)
The reports in the study with low levels of OC correspond to subnival humid grassland of the moorland (HsNn01) and subnival ultra-humid grassland of the moorland (HsNn02) with values of 47.25 Mg/ha and 39.37 Mg/ha of carbon, respectively. This is because these formations are located at 4500 m above sea level in the reserve. They are affected by topography, slope, precipitation, and temperature since they are specifically close to the glacier of Chimborazo and Carihuairazo volcanoes.
In this context, the researchers agree with Vistin and Barrero (2017), who claim that the richness and diversity of vegetation species decrease as altitude and latitude progress. This is confirmed when analyzing the vegetation species in these ecosystems, which are dispersed, that is to say, with little vegetation cover in geliturbized soils and, therefore, also low content of C. The most representative families are Poaceae and Asteraceae. The soil of these two ecosystems is constantly exposed to low temperatures, which favors the low rates of nutrient decomposition and recycling, the formation of stable organic-mineral complexes, and the decrease in the mineralization rate of organic matter. Thus, 14.44% of total organic carbon in the reserve was established between the two ecosystems.
Soil capacity of the Chimborazo Fauna Production Reserve to store and retain OC
The potential of this protected area to store OC is very high to be considered a natural carbon reservoir; without taking into account the aerial carbon of the vegetation, which is an essential and fundamental contribution to mitigating the effect of climate change on the world partially. This study arises from the need to obtain quantitative data on the environmental benefit offered by Chimborazo Fauna Production Reserve of the carbon stored concerning the vegetation type, taking into account the idea of establishing it as a clean development mechanism (CDM). The objective of the CDM is to maintain and expand carbon reservoirs to compensate for greenhouse gas emissions.
The soil sampling depth for this study was 0.3 m since this is the most sensitive to land-use changes. It is essential to consider that to obtain a maximum approximation to the actual values, each step in the whole process was strictly rigorous. From the 58560 ha of the RPFCH, 10929 ha were not quantified in C since they belong to a classification of intervened areas, so it is considered that 18.66% would not provide ecosystem services.
Total organic carbon in soils by vegetation type
Due to the heterogeneity of the species that live in the eight soil ecosystems of the RPFCH, the TOC per hectare values were established considering the area they occupy with each vegetation formation versus the OC stored in the entire study area (Fig. 3).
Based on the results obtained in the laboratory and its subsequent analysis, the evergreen forest of the moorland ecosystem stores the most OC in the soil. However, having a small extension, 362 ha, it accumulates 136893.92 Mg of OC, occupying the seventh place in the scale of vegetation formations that store the most organic carbon. The grassland of the moorland is next, established in 6274 ha, storing 1544345.10 Mg of OC. This ecosystem occupies the first place on this scale since it has a high OC value per hectare, in some places matching or exceeding the ecosystem mentioned above. These ecosystems are the ones that retain the highest OC per area unit.
The evergreen bushland and grassland of the moorland ecosystems report a total content of 428174.78 Mg of OC in 2563 ha, ranking fifth on this scale. The Upper high montane humid grassland of the moorland, flooded grassland of the moorland, and evergreen subnival grassland and bushland of the moorland present values of 67.31 Mg, 67.68 Mg, and 79.74 Mg of OC, respectively. These results indicate that these ecosystems store less carbon due to geographical and climatic conditions (precipitation, temperature, slope, etc.). The three ecosystems together add 23202 ha, highlighting that the upper high montane humid grassland of the moorland contributes 25% of the total carbon of the reserve. Despite the low values obtained in its soil, this ecosystem with the most significant extension, with 16343 ha, contributes positively to the scale of vegetation formations that store the most carbon, only behind the grassland of the moorland. Finally, the subnival humid grassland of the moorland and subnival ultra-humid grassland of the moorland are the ecosystems with the lowest carbon-storing values with 47.25 Mg and 39.37 Mg of OC respectively, adding together 13.94% of the total carbon stored.
Data analysis
The data used to analyze the relationship between the OM and TOCS variables resulted from the tabulation of the soil analyses and their subsequent calculation. First, an average of OC stored in the soil per hectare of the eight RPFCH vegetation formations was obtained and then multiplied by their extension to obtain the content of TOCS (Table 2).
TABLE 2 Determination of organic matter and TOC/ha in the eight vegetation formations of Chimborazo Fauna Production Reserve, Ecuador.
Vegetation type | Area (ha) | OM (%) | t/OC/ha |
Evergreen bushland and grassland of the moorland | 2563 | 11.57 | 167.06 |
Evergreen forest of the moorland | 362 | 18.11 | 378.06 |
Grassland of the moorland | 6274 | 12.09 | 246.15 |
Upper high montane humid grassland of the moorland | 16343 | 7.58 | 67.31 |
Subnival humid grassland of the moorland | 4163 | 4.85 | 47.25 |
Flooded grassland of the moorland | 765 | 7.20 | 67.68 |
Subnival ultra-humid grassland of the moorland | 11067 | 5.41 | 39.37 |
Evergreen subnival grassland and bushland of the Moorland | 6094 | 9.75 | 79.74 |
The covariance calculation was determined to obtain a value that reflects the degree to which the established variables are altered jointly concerning their values. In this regard, the values of the TOCS variable were transformed into percentages to have the same system of units as the OM and to measure the concordance degree of the relative positions of the analyzed data for each vegetation formation (Table 3).
In the covariance, where the researchers worked with not grouped data (x) for OM and (y) for TOCS, a positive value of 40.22 was obtained. This positive value indicates direct proportionality, indicating a strong correlation between the content of OM and TOCS in the soil. In this study, this result is strongly influenced by the type of vegetation formation present in each of the eight ecosystems of the reserve; this allows concluding that, the evergreen forest of the moorland and grassland of the moorland strongly influence the result. While the vegetation formation with a lower correlation is the Subnival ultra-humid grassland of the moorland (Fig. 3) however, the proportionality of OM is reflected in the TOCS.
Pearson correlation coefficient between the OM and TOCS content
In the relationship study for the TOCS between the OM and TOC, a positive linear trend is observed where the relationship between the dependent variables is stochastic (Fig. 4).
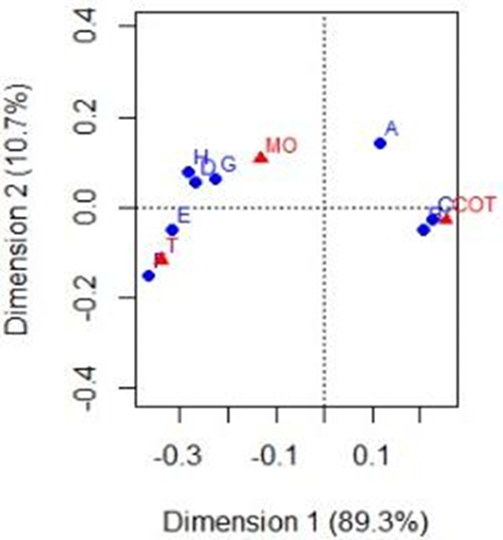
A: Evergreen bushland and grassland of the moorland, B: Evergreen forest of the moorland, C: Grassland of the moorland, D: Upper high montane humid grassland of the moorland, E: Subnival humid grassland of the moorland, F: Flooded grassland of the moorland, G: Subnival ultra-humid grassland of the moorland, H: Evergreen subnival grassland and bushland of the moorland.
FIGURE 4 Correlation analysis for the TOCS content between the OM and the vegetation formation type.
Scientific knowledge circulates from the general to the particular; in this regard, the explanation of new phenomena can be studied by the relationship it has with events of the same phenomenon, which is what the researchers want to determine in this investigation. Therefore, the need to establish the relationship between the two quantitative variables based on the covariance is established (Table 4).
Pearson's correlation yields a value of 0.50, a positive number in a range of (± 0.50 to ± 0.69 (Table 4), indicating a moderate positive linear association between these two variables. However, it is also observed that the incidence in the type of vegetation for carbon storage in the eight types of soils only represents a moderate value on the Pearson scale.
Discussion
The results of this investigation answer the research question: What is the organic carbon content in the soil of the eight vegetation formations of the Chimborazo Fauna Production Reserve that can be offered as carbon bonds as a mechanism for moorland ecosystem conservation and climate change mitigation?
Payments for environmental services, an overview in Ecuador
Payments for environmental eervices (PES) is a big challenge for rural communities within the protected areas in Ecuador. The lack of information, bureaucracy and national laws, and mainly the lack of scientific studies offering clear information about the ecosystem services the protected areas in Ecuador provide are a barrier to taking advantage of the benefits PES initiatives offer.
It is notorious that the more conserved hectares, the greater the need for economic investment for the delivery of economic incentives. In this regard, the Government of the Republic of Ecuador, from 2008 to 2015, covered a total of 1488373 ha, with an investment of around ten million dollars. This value is equivalent to seven years of implementation of the Socio Bosque Plan (PSB, by its Spanish acronym).
The implementation of incentive payments taken by the National Government is constantly growing. From 2008 to 2015, 1098723 ha were incorporated (MAE, 2013). Consequently, the incentives received 7.5 MUSD. According to the Socio Bosque Program database, in 2016, the tropical humid forest ecosystem received the highest economic incentives, with 85% of all conserved ecosystems (around 7 million dollars). The montane forest occupied second place with 67000 ha, equivalent to 5%, with an incentive that reached 1 MUSD. The moorland was in third place with an extension of 60740 ha, equal to 4% of the national total, with 1127498 USD. This value is greater than the one for the previous ecosystem but with fewer hectares. This is because the amounts of the incentive structure are greater for this ecosystem.
The goal established for the moorland ecosystem since its creation is between 600000 ha and 800000 ha (MAE, 2015). However, according to the institutional results of Socio Bosque, only 60740,14 ha have been conserved in the whole national territory. This figure represents 10% of the minimum target, which is a critical indicator that shows that, despite the provided incentives, the conservation of this ecosystem still needs a lot of work.
The leading global strategy against global warming is the reduction of greenhouse gases, which, based on the Kyoto Protocol, involves three economic instruments called flexibility mechanisms. These mechanisms oblige developed countries to fulfill their commitments to reducing greenhouse gases at the lowest possible cost. In this scenario, Ecuador has no obligation to reduce pollution under the Kyoto Protocol. However, the reduction and capture of greenhouse gas emissions offer the country opportunities to conduct business with governments and companies of industrialized countries and, through this alternative, contribute to the reduction of the causes of the climate change phenomenon; as well as to place the country on the path of truly sustainable development in the communities that conserve their territories.
Compensation for environmental services, an approach towards sustainability in the 21st century Global Warming results are not only an environmental disaster but fundamentally an economic and social difficulty for all the people inhabiting this planet (Food and Agriculture Organization [FAO], 2017). Under this context, an incentive is created for companies and individuals to change their investment, production, and consumption patterns. Through a clear and firm price signal, determining the carbon price establishes the right incentives for the necessary transition on a large scale towards an economy with low carbon emission levels.
Concerning prices, the Sistema Europeo de Negociación de CO2 [SENDECO2] provides a comfortable and safe environment for the negotiation of Carbon Dioxide Emission Rights and Carbon Credits, which determines a value of EUR 25.89, an average of the last twelve months. Meanwhile, Investing.com sets a minimum value of EUR 24.82 recorded until August 2019. On the other hand, the World Bank sets a value of 40 USD to 80 USD until 2020. Thus, the equation to calculate the economic contribution from carbon fixation can be applied by multiplying the values of the current reference price in the international market, the number of hectares, and the TOC. The result shows the contribution of each ecosystem with a value of 4379581.43 in carbon credits that can be offered to the international market. If the minimum average value, set by the three organizations responsible for setting carbon costs, is multiplied by 13.03 USD, a value of 57065490.43 USD is obtained. This value could be designated to the communities in the buffer zone and within the reserve; so that the pressure on this protected area of anthropic activity can be stopped and sustainable development be generated through the sale of carbon bonds.
This study found that the soil of the eight ecosystems of the Chimborazo Fauna Production Reserve, which has an area of 47631 ha without counting the disturbed areas, has 4379581.43 Mg of carbon stored with an average of 136.59 Mg/ha. These results agree with studies of Cely and López (2016) where soil carbon was quantified at 0 cm - 15 cm and 15 cm - 30 cm in the moorlands of Boyacá, Colombia, determining that one hectare of protected moorland can capture 200 Mg per year of CO2. In addition, Castañeda and Montes (2017) affirm that the soil is the component that stores the greatest amount of carbon concerning the aerial biomass at depths between 0 cm and 40 cm, which values ranging between 119 Mg/ha and Mg/ha. These differences may be because of the natural cover's density and the number of roots per unit area.
Conclusions
In the RPFCH soil, the highest contents of total organic carbon in very high soils (250 Mg/ha - ˃300 Mg/ha) occur in the evergreen forest of the moorland and grassland of the moorland formations because these two typologies have a greater amount of vegetation species of the Polylepis, Gynoxys, and Buddleja genera. However, it contains 3.13% of C stored. The grassland, represented by species such as Calamagrostis intermedia and Agrostis perennans, forms 75% of the ecosystem. Additionally, the results show that in valleys and cliff walls where the soil horizons are larger, there is a greater amount of OM and microbial activity, and the soil sets the highest OC content. The upper high montane humid grassland of the moorland stores 25.12% of the TOCS. Although in the laboratory samples, this ecosystem reports an average of 67.31 Mg/ha of carbon, it occupies an area of 16343 ha, representing 27.90% of the study area.
Considering that a carbon bond is equivalent to 1000 kg of captured or stored C, the RPFCH would offer to the international market 4379581.43 carbon credits. This amount of money multiplied by those established in the national territory from 2006 to 2011 in 13.03 USD, gives that 57065490 USD would enter Ecuador, which would help create conservation projects in the communities located inside the reserve as an alternative to sustainability and climate change mitigation.