Introduction
Floods are usually caused, among other factors, by excessive rainfall, rise of water table and poor irrigation management (Ganskopp, 1986; Stromberg, 2001) and their frequency and intensity are highly variable and unpredictable (Loreti, van Veen, & Perat, 2016). Lately, due to global climate change, frequency and intensity have increased (Sasidharan & Voesenek, 2015) exposing intolerant crops, many of which are fruit species, to radical anoxia.
In arid and semi-arid regions, characterized by low annual rainfall and high evapotranspiration, its groundwater that supplies perennial plants with their most important source of water (Naumburg, Mata-González, Hunter, McLendon, & Martin, 2005). The level of groundwater is dynamic, and its temporal variation is caused by several processes, including recharge, drainage and use of water resources (Ganskopp, 1986; Stromberg, 2001). This variation in groundwater level can cause contrasting effects. The decrease in level can generate water deficit in plants (Naumburg et al., 2005). On the contrary, its rise increases water availability for plants but it can also submerge the active roots and cause depletion of soil O2, generating radical anoxia (Kozlowski, 1997; Armstrong & Drew, 2002). However, the flooding process can be gradual and affect only a part of the radical system.
Most tree fruit species, including Prunus genus, are propagated from grafting of the productive variety, on rootstocks belonging to the same or other species (Webster, 2001; Gillen & Bliss, 2005). The influence of rootstocks on cultivars is significant, since they are responsible for absorption of water and nutrients (Layne, 1987), and can determine, to some extent, the tolerance of fruit trees to different types of environmental stresses such as drought, salinity, low temperatures and hypoxia (Isaakidis, Sotiropoulos, Almaliotis, Therios, & Stylianidis, 2004; Domingo, Pérez-Pastor, & Ruiz-Sánchez, 2002; Pimentel et al., 2014).
There are different degrees of susceptibility to flooding among peach rootstocks (Ranney, 1994; Martinazzo, Perbon, Farias, Bianchi, & Bacarin, 2011; Amador, Sancho, Bielsa, Gomez-Aparisi, & Rubio-Cabetas, 2012; Iacona et al., 2013; Ziegler, Ploschuk, Weibel, & Insausti, 2017) being ‘Nemared’ (Prunus persica (L.) Batsch) a susceptible specie. This rootstock is one of the most used peach rootstocks because it is resistant to root-knot nematode and is compatible with peach, nectarine and plum (Ramming & Tanner, 1983). As is the case in other species that are not very tolerant to flooding, radical hypoxia affects the growth of grafted peaches on intolerant rootstock and physiological parameters related to this (Iacona et al., 2013) like water potential (Ѱw), stomatal conductance (gs), and leaf chlorophyll content (Domingo et al., 2002; Martinazzo et al., 2011; Insausti & Gorjon, 2013; Pimentel et al., 2014; Ziegler et al., 2017).
Given the genesis of soil flooding, when this event occurs, it may affect only a part of the radical system. Therefore, the most superficial part of the rhizosphere could be oxygenated. Thus, considering complete or partial (up to the half) flooding of the radical system, this question arises: does flooding stress differentially affect vegetative growth of ‘Nemared’ peach rootstock plants, and some physiological variables associated with water and carbon balance, under each flooding condition? There is a large amount of information about the negative effects of stress caused by total flooding of roots in peach plants (Domingo et al., 2002; Martinazzo et al., 2011; Pistelli et al., 2012; Iacona et al., 2013; Insausti & Gorjon, 2013; Pimentel et al., 2014; Ziegler et al., 2017). However, there is no information about the effects of a partial flood, half of the radical system, on peach rootstocks. Therefore, the objective of this work was to investigate the physiological and growth response of ‘Nemared’ rootstock when this covers up to half of the radical system as contrasted with total flood.
Materials and methods
Plant material and experimental design
The experiment was carried out in an open field at the Agricultural Experiment Station EEA INTA Junín, in the province of Mendoza (Argentina) during January of 2015. Six-month-old rootstock cv. ‘Nemared’ (Prunus persica [L.] Batsch) was used due to its high susceptibility to total soil flooding (Ziegler et al., 2017). They were obtained through sexual reproduction (Ramming & Tanner, 1983). The rootstocks were transplanted from the nursery row to the pots in July, during winter rest, in open field covered by plastic anti-hail mesh. They grew in plastic pots for six months before starting the different treatments, with the aim of ensuring that the roots occupy the entire volume of the pot.
The selected plants were homogeneous as regards dimensions (height, stem diameter and number of stems) and were placed in 40 L pots containing a mixture of perlite, peat and grape marc (30:35:35, v/v) as substrate. Pots were randomly distributed on the ground using a completely randomized design and they were irrigated regularly. In addition, water-soluble fertilizer containing NPK 15-10-15 with micronutrients chelated in EDTA (Mg-S, B, Cu, Fe, Mn, Zn and Mo) (2-30-0.01-0.02-0.05-0.05-0.02-0.001) was applied once a week during the growing period.
At the beginning of the experiment, plants were 150 cm high (measured on trunk), and the roots occupied the entire volume of substrate in the pot. Before starting the experiment, a sample of three plants from each treatment was taken to verify that the roots were homogeneously distributed throughout the volume of the substrate in the pot profile. The treatments were as follows: 1) control, plants irrigated to prevent water stress, 2) half flooded, consisted in maintaining a height of water equivalent to half the height of the pot, and 3) fully-flooded, water level kept at 50 mm above the soil surface. To avoid water loss from flooded pots, they were placed in 50 L containers without drainage. Control tree pots were also placed in 50 L pots, but with drainage. Seven repetitions were used per treatment for a total of 21 plants under trial. Flooding treatment was continuous and lasted for 6 days.
Physiological measurements
Stomatal conductance (gs) was measured in mature fully expanded leaves located in the middle third of the central axis of each plant. The measurement was performed using a diffusion porometer (Delta T AP4, Delta-T Devices, Cambridge). The water potential (Ѱw) was measured in the same leaf where gs was quantified, using a pressure chamber (Bio-Control, Argentina). Leaf chlorophyll content was evaluated using a chlorophyll portable meter (CL-01, Hansatech Instrument Ltd., UK), on five leaves located in similar positions in each plant.
All physiological measurements were performed before starting treatments and at 0, 1, 2, 3 and 4 days after starting the flooding treatment, always between 11:00 am and 2:00 pm. On the fifth and sixth day no physiological measurements were made due to inappropriate weather conditions.
Growth measurements
Length and diameter of shoots and trunk diameter were measured and related to values at the beginning of the experiment to obtain the cumulate relative growth. The selected shoots were in the same position within the plants. These measurements were made at the beginning of the experiment, 3 and 6 days after the start of flooding treatments using a folding ruler and a digital vernier caliper. The results of the growth measurements were expressed as a percentage of cumulative increase with respect to the initial measurement.
Environmental variables
At the time when physiological variables were measured, the air vapor pressure deficit (VPD) was calculates using daily data of air temperature and atmospheric relative humidity (%). All measurements were made on sunny days and records of global solar radiation oscillated between 860 y 900 Wm-2. The data was obtained by using sensors installed in a micro meteorological station (Agrometrix GEA-X1, Agrometrix, Argentina) located in the site where the experiment took place.
Statistical analysis
Data were analyzed using analysis of variance. Previously, assumptions of normality and homogeneity of variance were verified. To analyze the response variables recorded, a repeated measure test was used (Moser, Saxton, & Pezeshki, 1990) and means were compared using the Tukey test (P <0.001). All results are presented as the mean ± standard deviation.
Results
Vapor pressure deficit
On the second day of treatment, the VPD in the atmosphere was 1.5 kPa. This moment coincided with the measurement of the maximum gs value in the control treatment. On the other days, the VPD presented similar values of 2.25 kPa.
Physiological responses
Effect of flooding on stomatal conductance
Stomatal conductance was significantly different among all treatments (P < 0.001) after 1 day from the start of the experiment (Figure 1A). At that time, the reduction of gs in half and fully-flooded treatments, in relation to the control, was 65 and 88 % respectively. Likewise, the reduction of gs in fully-flooded treatment with respect to half-flooded treatment was 66 %. After 2 days of beginning the flooding treatment, gs of both treatments was reduced by 90 % with respect to the control. No further significant differences in the gs between the two flood treatments were detected, always with respect to the control.
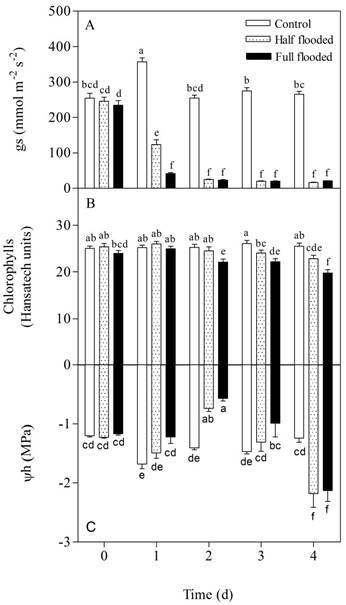
Figure 1 A) Stomatal conductance (gs), B) chlorophyll, and C) leaf water potential (Ѱw) of peach rootstock ‘Nemared’ subjected to three treatments of flooding (control, half and fully flooded) for four days. Error bars indicate standard errors of the means. Different letters indicate significant differences between treatments and between days (Tukey, P <0.001).
Effect of flooding on leaf chlorophylls
Fully and half-flooded treatments significantly reduced chlorophyll content of leaves with respect to control (P < 0.001) after 2 and 3 days, respectively (Figure 1B). After 4 days of flooding, chlorophyll content of leaves in half and fully-flooded treatments decreased by 9 and 20 % respectively, in relation to the control. At this moment there were also significant differences (P < 0.001) in the leaf chlorophyll content between the two flood treatments.
Effect of flooding on leaf water potential (Ѱw)
Leaf water potential of fully-flooded treatment differed significantly from the control one day after starting the treatment until the end of the experiment (P < 0.001) (Figure 1C). While Ѱw of half flooding treatment did not differ significantly (P > 0.05) from the control on the first day from the beginning of the experiment, but there were significant differences between both treatments on days 2 (P < 0.001). On the third day of the experiment, again only fully-flooded treatment had Ѱw values significantly higher than the control (P < 0.001), even though stomatal closure was similar for both flood treatments. However, on the fourth day, the Ѱw of both flooding treatments was significantly lower compared to the control treatment (P < 0.001), without change in gs.
Effect of flooding on plant growth
The accumulated growth in trunk diameter in both flooding treatments was significantly lower (P < 0.001) with respect to the control plants on the sixth day of treatment (Figure 2A). However, there were no significant differences (P > 0.05) in this growth variable between both flooding treatments (Figure 2A). The accumulated growth in shoot length was significantly lower in both flooding treatments compared to the control (P < 0.001) on the third and sixth day of treatment, with no difference between them (Figure 2B). The accumulated growth in shoot diameter in both flooding treatments was significantly lower than the control (P < 0.001) on the sixth day of flooding, without differences between the two flooding levels (Figure 2C). The control treatment plants had an accumulation of stem diameter, shoot length and diameter of 73, 72 and 62 %, respectively, compared to flood treatments.
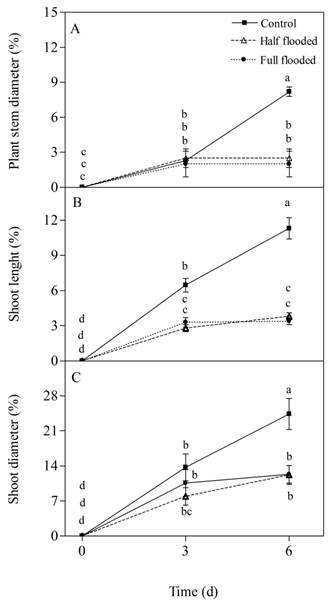
Figure 2 A) Cumulative growth expressed as a percentage of cumulative increase with respect to the initial measurement of plant stem diameter, B) shoot length, and C) shoot diameter, of peach rootstock ‘Nemared’ subjected to three treatments of flooding (control, half and fully flooded) for six days. Error bars indicate standard errors of the means. Different letters indicate significant differences between treatments and between days (Tukey, P <0.001).
Discussion
‘Nemared’ peach rootstock is very susceptible to soil flooding and, like other rootstocks from Prunus persica, it decays when planted on soils with flood episodes, and even dies if the stress persists over time (Andersen, Lombard, & Westwood, 1984; Ranney, 1994; Insausti & Gorjón, 2013; Ziegler et al., 2017). The fast-negative response to flooding recorded in this work coincides with the results obtained by Ziegler et al. (2017). However, in some variables, there is a differential behavior, whether the flood was full or half.
Stomatal conductance was the most rapidly affected variable in response to flooding (within the first 24 h). However, gs of half flooded plants were 2.5 times greater than the fully-flooded ones. Probably soil oxygenation, with half of the radical system unaltered, was the cause of the differential response between both flood treatments. However, this result shows that the stomatal sensitivity of this rootstock to soil flooding is high, since flooding of only half of the radical system was sufficient to cause, within the first 24 h, a stomatal opening three times lower than the control.
According to our results, Ѱw of both flood treatments increased after 48 h, achieving the highest values for both flood treatments. Therefore, it is assumed that water absorption by roots was not affected during the first two days of flooding. The high values of Ѱw, together with lower values of gs, suggest that stomatal closure was not a consequence of a hydraulic signal, but it was due to an anticipated response of another type, for example hormonal, which was generated as a consequence of a flood effect. This hypothesis should be tested, however, it is clear that the rapid response of stomata is due to greater speed in the signal-response mechanism that modulates stomata conductance (Vreeburg, Benschop, Peeters, Colmer, & Ammerlaan, 2005).
However, from the third day, the trend of the Ѱw changed and the lowest values for flooding treatments were recorded on the fourth day, with a significantly highest value in the control. This change in water potential response, without changes in gs or other factors that would be affected by water balance, it could be due to the fact that, after that period, the absorption of water by roots in both treatments could have been affected by flood itself. The negative effect of floods on the functioning of aquaporins in root cell membranes is well known (Tournaire-Roux et al., 2003; Tan et al., 2018) and so is their effect on water absorption.
On the other hand, a significantly greater stoma opening in the control plants was evident after 24 hours of the beginning of the experiment, with respect to the same treatment in the rest of the days. This answer can be explained as a consequence of the lower value of VPD that day. This effect of VPD on gs is widely known (Lambers, Chapin, & Pons, 2008).
Chlorophyll degradation responds to the level of flooding, being maximum with fully-flooded, and intermediate with half-flooded treatment. However, the response to flooding was very fast compared to data from other authors (Martinazzo et al., 2011; Insausti & Gorjon, 2013; Pimentel et al., 2014). Chlorophyll degradation is an active and progressive process that is regulated by various internal development and environmental factors and is mainly mediated by hormone signaling (Koyama, 2014), among which ethylene plays a very important role (Yin et al., 2016). During a flood, ethylene accelerates the degradation of chlorophyll by expressing the yellow coloration in the leaf mesophyll (Jiang, Cao, Dai, & Jing, 2003; Barnawal, Bharti, Maji, Chanotiya, & Kalra, 2012; Chen et al., 2016). When soil flooding only affects a percentage of the roots, it is likely that the mechanisms involved in the degradation of ethylene-mediated chlorophyll, which underlies the 1-aminocyclopropane-1-carboxilic acid generated in the flooded root area, may have been affected by unflooded ground oxygen (Ahmed, Nawata, & Sakuratani, 2006).
In relation to vegetative growth, it is widely known that this is rapidly affected in flood situations (Domingo et al., 2002; Iacona et al., 2013; Insausti & Gorjón, 2013, Ziegler et al., 2017). According to the results of this experiment, this response occurred at both levels of flooding, when the physiological variables evaluated, which are related to it, were affected. Vegetative growth necessarily requires a combination of cell division and expansion (Lambers et al., 2008). Water potential is the hydraulic force that, through its pressure component, participates in the cell expansion process (Lambers et al., 2008). There are previous works that have reported that floods affect cell expansion in many species (Setter & Laureles, 1996; Voesenek & Blom, 1999) through their effect on the elasticity of cell wall (Cosgrove, 1993, 2005). Hydraulic changes and stomatal closure, together with lower chlorophyll content in the leaves, which may have affected photosynthesis of flooded plants, could be the main causes of the lower final growth in diameter and elongation of the branches, as well as the stem thickening.
Conclusion
According to our results, the effect of flooding on the ‘Nemared’ rootstock can occur during total floods and with some variations, also during half floods. This response should be tested at crop level. The importance of this fact is that the occurrence of a half flood goes unnoticed because it affects the lower part of the radical system. This is frequent that occurs when the water table rises, even for a short period of time, because of flood or excessive irrigation.