INTRODUCTION
In riverine wetlands, the intra-annual distribution patterns of the concentration of physicochemical variables in the water column differ mainly between the low and high water level conditions, whose surface hydraulic interconnection maintains its natural flow (Brinson, 1993; Junk & Wantzen, 2004; Thomaz et al., 2007). In this way, the values of chlorophyll-a (Chl-a) and nutrients are influenced by the lateral exchange of water due to temporal flooding, residence times and inputs of organic matter by runoff (Tubatsi et al., 2014; Fritz et al., 2018). For example, the increase of algal biomass has been frequently recorded during the low water level (WL) condition (Brito et al., 2014; Roach et al., 2014; Cruz-Ramírez et al., 2019). On the contrary, its decrement has been recorded in conditions of high WL (for example, Noe et al., 2013) since its fluctuation has been linked to the flood pulse that allows the dispersion of the planktonic community in areas with seasonal inundation (Junk & Wantzen, 2004; Noe et al., 2013). Moreover, the enrichment in nitrogen and phosphorus has been indistinctly mentioned as a dominant abiotic processes tied to the spatial-temporal variability in the WL of the river-floodplain systems (for example, Brito et al., 2014; Tubatsi et al., 2014; Cruz-Ramírez et al., 2019).
However, the high variability of the concentrations of nutrients in short times regarding the inter-annual fluctuation of the WL can enhance the eutrophication (carbon, nitrogen, phosphorus), which in turn increase the phytoplankton biomass (Dodds, 2007; Cruz-Ramírez et al., 2019). Additionally, wastewater discharges, diffuse input of agricultural nutrients, runoffs and hydraulic disconnection are other causes associated with the enhancement of nutrients in river-floodplain systems (Cetin 2009; Sharma et al., 2010; Sánchez et al., 2012; Jeppesen et al., 2015; Lázaro-Vázquez et al., 2018).
Frequently, the hydraulic disconnection has been related to physical obstruction by infrastructure constructed for different purposes. The infrastructure built to store water or prevents floods as well as the urban sprawl has been the main anthropogenic causes that have impaired the natural variations in the WL in perturbed river-floodplain system (Poff et al., 2007). For instance, the reduction of the flow referential values, as well as changes to water quality in rivers was associated with a South Africa impoundment (Mantel et al., 2010). Moreover, the construction of roads and levees has caused hydraulic disconnection between the river and its flood area in USA (Coffin, 2007; Blanton & Marcus, 2009), which in turn has originated a decrement of the natural variation of the WL linked to the increment of the concentration of nutrients and eutrophication (Jeppesen et al., 2015).
The Maluco river-floodplain system was temporally interconnected with the Grijalva River until the early 80´s, when its hydraulic interconnection was drastically reduced from three to one storm drains placed under the highway, which was built on a natural barrier (Gil & Saenz, 1872). Since this time, the highway has worked as an embankment that has restricted the lateral connection, due to that the bankfull stage was heightened up to 4.5 m above the sea level. Although, the construction of channels and embankments has been the most common public policy to avoid inundations in the cities located in the floodplain of the Grijalva Basin (Sánchez et al., 2015), there are no environmental data available on the Maluco river-floodplain system before the 80´s to corroborate the magnitude of the awaited environmental impacts by the restriction of the hydraulic interconnection.
The reduction of lateral interconnection due to the physical effect of the embankment reduces the probability that the Grijalva River has overflowed above the bankfull stage, which opens the hypothesis that there will be no intra-annual differences of Chl-a and nutrients. To test the hypothesis, the concentrations of Chl-a, nitrogen and phosphate nutrients were measured at different WLs of a hydrological year over a 2-year period in the Maluco river-floodplain system.
METHODS AND MATERIALS
Study area. The Maluco river-floodplain system is located in the floodplain of the Grijalva Basin. The Grijalva River is originated 17.6 km upstream the study area, where the dammed Mezcalapa-Carrizal and the free-flowing Sierra-Pichucalco rivers merge. The Mezcalapa-Carrizal Rivers have four dams built in the mountains of the Grijalva Basin, which stored up to 26,081 mm3 at the ordinary high WL (Navarro & Toledo, 2008). Both rivers drain an average 17,364.17 mm3/y downriver (DOF, 2018). Whereas, the Sierra-Pichucalco Rivers drain an average 3,186.76 mm3/y (DOF, 2018) before merging to the Mezcalapa-Carrizal River. In the study area (1,580.4 ha) Eichhornia crassipes (Mart.) Solms, Pistia stratiotes L., Nymphaea ampla (Salisb.) DC.,Thalia geniculata L.and Typha domingensis Pers. are the recorded aquatic macrophytes. The adjacent lands of the lagoon are used for growing crops and raising livestock (Palma-López et al., 2007). As was mentioned above, the lateral exchange of water in the Maluco river-floodplain system was restricted by a highway built in the early 80´s.
The Maluco river-floodplain system is located 23 km downstream from the Metropolitan Area of Villahermosa city with a population of 755,425 inhabitants and 77 sewage discharge points. These 77 sites drain 282 Mm3/y into the Grijalva river and nearby wetlands, of which 18% have limited or no treatment (INEGI, 2011; 2012).
Sampling design. The Maluco river-floodplain system was sampled bimonthly during two hydrological years (2004 and 2005) to measure the intra-annual variation of average WL (Fig. 1). Two sites (18º 05’ 24.49” N, 92º 45’ 34.30” W and 18º 05’ 22.47” N, 92º 45’ 10.45” W) were simultaneously sampled in six sampling times (Fig. 2), which summed a total of 24 records per physicochemical or biological variable. On each occasion the WL (m) was measured by a depth sounder (PS-7). The maximum WL in the wetland (13 m) was referenced at 4.5 m above sea level (masl), which is equal to the altitude of the embankment. Water samples were also collected at the medium depth with a van Dorn bottle and stored at 4° C for laboratory analysis. Nitrite (NO2-), nitrate (NO3-), ammonium (NH4+) and total phosphorus (TP) concentrations in mgL-1 were determined according to APHA (1998) procedures; whereas, Chl-a (µgL-1) analyses were done immediately following the recommendations of SCOR-UNESCO (1966).
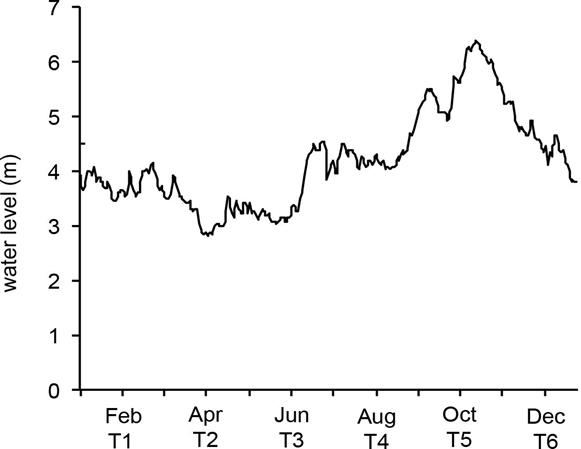
Figure 1 Intra-annual variation of average water level of Grijalva River from 1995 until 1999 in the 30083 hydrometric station in the Grijalva River. Black line= water level, T= sampling times. Data source: CONAGUA (2012).

Figure 2 Location of study area. Embankment = , floodplain =
,
Metropolitan Area of Villahermosa =
, hydrometric
station= black star, sampling sites in the river-floodplain system=
black circle. Map modified from http://antares.inegi.org.mx/analisis/red_hidro/SIATL/#
(INEGI, 2013).
Data analysis. The intra-annual variation of the physicochemical variables (NO2-, NO3-, NH4+, TP and, Chl-a) among the six sampling times was analyzed through a Principal Components Analysis (PCA). The PCA was based on a correlation matrix, and the dissimilarities were calculated based in the Euclidean distance after standardization of the five original variables (Legendre & Legendre, 2003). Additionally, the principal components (PC) with eigenvalues >1 (Kaiser-Guttman criterion) and significant variation (Bartlett; p<0.05) were selected (Legendre & Legendre, 2003). Then, the variables with absolute values in the eigenvectors greater than |0.4| and by having significant contributions were considered in the selected PC´s (Weilhoefer et al., 2008). These analyses were carried out with the JMP v10 Software (SAS Institute, 2012). Before the PCA calculation, the correlation coefficients (>|0.3|) and the determinant of the correlation matrix (0.325) were used to find the collinearity among the five variables analyzed. The multicollinearity was reviewed by means of Bartlett’s test of sphericity (p=0.011) and the Kaiser-Meyer-Olkin test (0.523), since the variables showed a medium degree of inter-correlation (Tabachnick & Fidell 2014). The last four analyses were carried out through the IBM SPSS Statistics software v24 (IBM Corp, Armonk, NY, USA). Finally, the similarity profile routine (SIMPROF) was used to determine the possible significant differences among the groups. The SIMPROF was applied with a significant level of p<0.05 (Clarke et al., 2008) through the program PRIMER v6.1.6 (Clarke & Gorley 2006). The interpretation of the variables selected was complemented by the calculation of the mean, standard deviation and error of each sampling time (four data by sampling time).
RESULTS
No variable was discarded during PCA. The PC1 (eigenvalue= 2.101) explained 42% of the significantly variation in the riverine wetland (Bartlett; p=0.006). In this first axis (PC1), Chl-a (0.567), NH4+ (0.502), and NO3- (0.497) were positively associated, while NO2-(-0.402) was negatively associated. Whereas, the PC2 (eigenvalue=1.265) described 25.3 % of the variation, but it was not significant (Bartlett; p=0.15). This second axis showed a positive relationship with NH4+ (0.463) and negative with TP (-0.738).
The analysis of the distribution of samples indicated an intra-annual similarity (SIMPROF; p=0.149) since 100% of samples were grouped (Fig. 3). The second and third sampling times presented the highest concentrations of Chl-a, NH4+, and NO3-. Indeed, in the CP1, the low percentage of the explained variation was associated with the minimal dispersion of the samples with the time.
It stands out that 54% of the total of Chl-a records exceeded 25 µgL-1, up to a maximum concentration of 208 µgL-1 (Fig. 4). However, their concentrations showed a tendency to be higher during the first three sampling times (Fig. 4). Likewise, 66.7 % of the total TP concentrations were greater than or equal to 0.1 mgL-1, although the two maximum concentrations (> 1 mgL-1) were measured in the fifth sampling time (Fig. 4). The 25% of NH4+ concentrations were greater than or equal 1 mgL-1, while only the 4.2% of the NO3- values exceeded this value (Supplement 1). NH4+ values greater than 1 mgL-1 were quantified in four of the six sampling times (2, 3, 5 and 6). Instead, NO3- concentrations only surpassed 1 mgL-1 in the second sampling (Supplement 1). Finally, none of the NO2- values exceeded 1 mgL-1, and they recorded similarity among the six times (Supplement 1).
The maximum values of WL were lower than the overflow level of the bankfull stage, which was estimated at 13 m (Supplement 1). Although higher concentrations of Chl-a, and TP were recorded throughout the study time, the highest values of Chl-a, NO3- and NH4+ coincided with the minimum values of WL, quantified in the 2 and 3 sampling times (Fig 4).
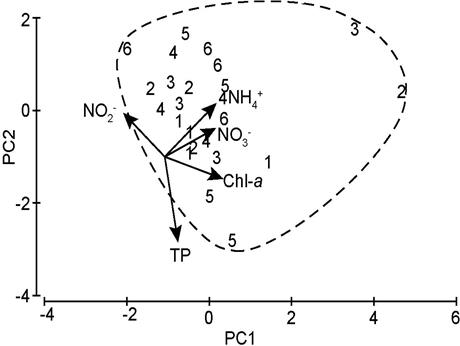
Figure 3 PCA bi-plot of the first two components synthesizing the data of the Chlorophyll-a (Chl-a), Nitrite (NO2-), Nitrate (NO3-), Ammonium (NH4+) and total Phosphorus (TP) at the Maluco river-floodplain system. The samples are classified by intra-annual sampling times (1-6). Dashed line=group.
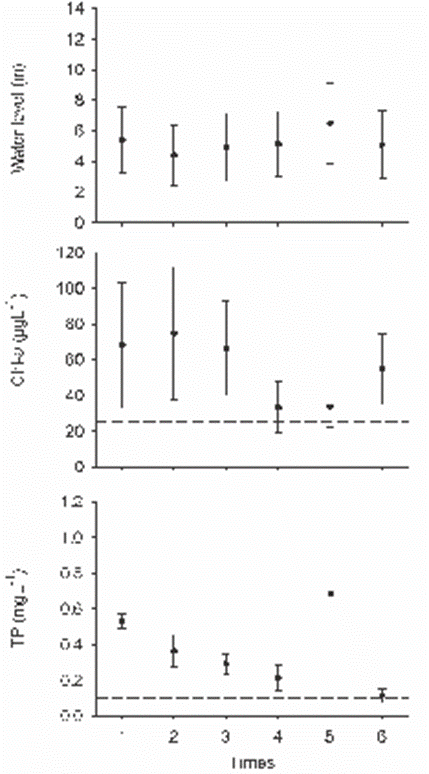
Figure 4 Mean and standard error of water level (WL), Chlorophyll-a (Chl-a), total Phosphorus (TP) in the Maluco river-floodplain system. Dashed lines are the limit to define a condition: bankfull stage = 13 m, hypereutrophic condition of Chl-a= 25 µgL-1 and TP= 0.1 mgL-1 (OECD, 1982).
DISCUSSION
The lack of the intra-annual variation of physicochemical and biological water variables in the Maluco river-floodplain system has also been reported for other hydraulic altered river-floodplain systems (Jeppesen et al., 2015; Li et al., 2019). In the study area, the maximum concentrations of Chl-a occurred during the first three times, but all their values surpassed the hypereutrophic reference value (≥ 25 µgL-1), according to OECD (1982), throughout the hydrological year (Supplement 1). Also, the nitrogen nutrients levels were below 1 mgL-1 throughout the six times sampled (Supplement 1) which is the reference inorganic enrichment value (Wilde et al., 2015; Zhu et al., 2015). Therefore, the intra-annual similarity among the concentrations of Chl-a, nutrients and water level can be related to two years of hydraulic of disconnection between the Grijalva river and the Maluco floodplain due to the fact that maximum WL measured values (≤ 12 m) promoted the persistence of the hypereutrophic conditions, since the overflow of the bankfull would begin when the Grijalva river reaches 13 m of WL. Likewise, the persistence in time of the hypereutrophic conditions (Chl-a and TP) in the Maluco river-floodplain system agree with other results in hydraulically altered river-floodplain systems (Pinilla, 2010; Reckendorfer et al., 2013; Li et al., 2019), or with different interannual times of hydraulic connection (McKinney & Charpentier, 2009). Instead, the variation of Chl-a and nutrients in non-perturbed river-floodplain systems differed significantly in relation to WL, mainly between low and high water level (Brito et al., 2014; Tubatsi et al., 2014; Roach et al., 2014; Cruz-Ramírez et al., 2019). Indeed, the maintenance of the water connectivity conditions, natural flow cycles, and flood pulses is indispensable to keep the biogeochemical processes that maintain the ecological functions and services provided by the river-floodplain systems (Junk & Wantzen, 2004; Marton et al., 2015).
In the Maluco river-floodplain system, the highest values of TP in time could be better explained by the wide fluctuation of its inputs throughout the rivers, as has been registered for others riverine ecosystems (Jarvie et al., 2005; Guan et al., 2016). In the case of the Grijalva floodplain, the TP concentrations increased significantly during the high water level in sites surrounded by extensive suburban areas (Lázaro-Vázquez et al., 2018). They related the increment of TP to the wastewater discharges, which were mainly caused by the expansion of agricultural areas and growth of human populations.
The restriction of the hydraulic connectivity by the construction of embankments and roads has also been linked to eutrophication processes (Coffin, 2007; Reckendorfer et al., 2013). In this sense, the river-floodplain system in this study kept a hypereutrophic condition independent of the time since all Chl-a and TP concentrations were greater than or equal to 25 µgL-1 and 0.1 mgL-1, respectively. Both concentrations correspond to hypereutrophic conditions according to OECD (1982).
The additional input of wastewater without treatment intensifies the hypereutrophic processes in aquatic ecosystems isolated hydrauli- cally (Jeppesen et al., 2015). In hydraulically perturbed river-floodplain systems, the reduction of the natural fluctuation in the water levels, combined with a probable low rate nutrient assimilation by primary producers, contributes to exacerbate the eutrophication (Pinilla 2010; Reckendorfer et al., 2013; Jeppesen et al., 2015; Salk et al., 2018). Therefore, the almost permanent hypereutrophic conditions registered in the Maluco river-floodplain system can be linked to the combined effect of the hydraulic disconnection, inputs of wastewater without treatment and shallowness of the ecosystem. However, this rationale will need to be demonstrated for the study area.
In conclusion, the current WL fluctuation in the Maluco river-floodplain system is insufficient to allow the often-recorded intra-annual variation between physicochemical variables and water levels in interconnected river-floodplain systems. The condition of hydraulic isolation helps to explain the intra-annual similarity of Chl-a and nutrients, the maximum WL values in the study area were lesser than the height (4.5 masl or 13 m) of the bankfull stage, and the persistence of hypereutrophic conditions linked to the Chl-a and TP enhancement. This persistence of time-independent hypereutrophic conditions may be a hypothesis to be tested by estimating the minimum input flow of water needed to restore the biogeochemical processes in the Maluco river-floodplain system.