Introduction
The Mediterranean central area of Chile is a hot spot for biodiversity conservation (Myers et al., 2000; Valdovinos, 2008) due to the high diversity and species richness of some taxonomic groups like Plecoptera, Trichoptera, and Ephemeroptera (Figueroa et al., 2013; Palma & Figueroa, 2008). In this area, physiographic and climatic conditions promote fluvial habitat diversity that enhances the fluvial habitat patchiness in low order rivers (Andreoli et al., 2012). However, the concentration of agricultural activities threatens the natural hydrologic regime of the rivers (Bonada & Resh, 2013; Figueroa et al., 2013; Habit et al., 2006).
Agriculture activities mainly increase the concentrations of nitrate and phosphorus on the aquatic ecosystems (Dudgeon et al., 2006; Vörösmarty et al., 2010) in several basins of the central zone of Chile. The actual concentration of these compounds do not represent a hazard condition (Pizarro et al., 2010; Ribbe et al., 2008), but increasing demand of water in agriculture should modify the flow and hydromorphological characteristics of low order rivers (Andreoli et al., 2012).
The natural flow regime is fundamental in low order river conservation, because it produces shifting habitat conditions (Poff et al., 1997; Stanford et al., 2005; Townsend et al., 1997), which increases the aquatic biodiversity (Death 2010; Townsend et al., 1997). Most of the scientific literature about flow alteration in Chilean rivers focuses on the relation between aquatic fauna (fish assemblage) and hydroelectric dams (Andreoli et al., 2012; García et al., 2011; Habit et al., 2007). For example, Habit et al. (2007), detected changes in the fish assemblage by the construction and operation of the Laja and Rucúe dams, since irrigation water withdrawals in the Laja River tends to increase the perturbation on the river’s ecosystem. Moreover, García et al. (2011), detected changes in the hydraulic characteristics (velocity and depth) of the Biobio River due to the daily peaks of electric demand during summer with possible effects on fish feeding behavior or spatial distribution.
Similar to other world regions (Bonada & Resh, 2013; Chessman et al., 2011; Dewson et al., 2007a), in the central zone of Chile many irrigation users collect water from low order rivers during their base flows of spring and summer (Figueroa et al., 2007). Thus, the aim of our study was to determine the effects of water abstraction on the habitat of benthic macroinvertebrates in low order rivers of Chile’s central zone. We also determined the relationship between macroinvertebrate biotic indices (CHSIGNAL and EPT) and flow reduction to improve the conservation of Mediterranean rivers in Chile.
Material and methods
Characteristics of the sampling sites. We selected 3 sampling sites (Dehesa, Recinto, and Marchant) with different regimes of water abstraction in the Itata basin. Due to the position of the diversion points in private lands and geographical access, we were only able to collect information in the selected points. All the sampling sites are located in different low order rivers of the Itata basin, a Mediterranean climate area with annual average temperature of 14.1 °C and 1550 mm of precipitation (Di Castri & Hajek, 1976; Urrutia et al., 2009a).
Native forests of evergreen Drimys winteri and Nothophagus spp. are common in the foothills, while agriculture landscapes prevail in the central valley (Figueroa et al., 2013). According to Figueroa et al. (2007), and Debels et al. (2005), there is good water quality in the watershed; the sewage discharge of the city of Chillán is the only factor related to nutrient enrichment at a lower altitude (see Fig.1).
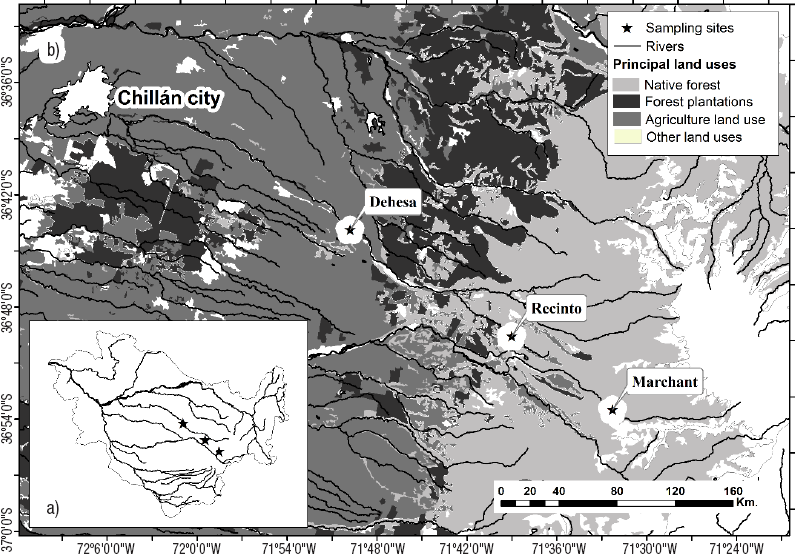
Figures 1a-b Location of the sampling sites in the Itata basin (a) and principal land use (b) in the mediterranean central area of Chile.
All sampling sites have their channel morphology modified by the construction of small weirs with boulders, sand bags, or wood. Steel doors and sand bags partially seal the irrigation channels during the winter flow, and avoid inundation of agricultural field in Dehesa and Marchant sites. The riverbed is composed of an alternate combination of cobbles with riffle and pool, a common characteristic of the rivers in Chile’s central zone (Andreoli et al., 2012; Figueroa et al., 2013).
Habitat sampling. At all sampling sites, we selected a 100 m reach section before (control) and after (impact) the weirs. According to Dewson et al. (2007c), 100 m reach is enough to observe the effects of water abstraction and there is no chance of flow recovering by groundwater. To characterize the trophic status of the sampling sites, we collected water samples for NO2 (mg/L), NO3-(mg/L), NH4+ (mg/L), Organic N (mg/L), Total N (mg/L), Organic P (mg/L), Total P (mg/L), PO43-(mg/L), and Suspended solids (mg/L) analysis in March (low flow) and August 2013 (high flow). Water samples were kept cold until their analyses in the Environmental Chemistry Laboratory, EULA Center for Environmental Sciences, University of Concepción (Eaton et al., 2005).
We recorded the habitat characteristics (water depth, river wetted width, current velocity) and in situ chemical parameters in six random points on every reach during the low flow period (October, December 2012, and March 2013) and high flow period (August 2013). The selected in situ physico-chemical parameters were temperature, conductivity (Hanna Instruments HI 9835), pH (Hanna Instruments HI 9126), and dissolved oxygen (Hanna Instruments HI 9146).
Stream discharge (L/s) was determined in control and impact reach by 10 equidistant measurements of depth and current velocity (0.6 depth) with a Gurley 1100.1 Flow Velocity Indicator (Gore, 2007).
Macroinvertebrate sampling. We sampled benthic macroinvertebrate community for each stream reach section on all sampling dates. All the macroinvertebrate sampling sites were near to habitat sampling. A Surber sampler (mesh size=250 μm, 0.09 m2 area) was placed in 6 random points to remove organisms. The organisms collected were fixed in 95% alcohol and transported to Bioindicators Laboratory at the EULA Center for Environmental Sciences. We identified the macroinvertebrates to the family level with the available taxonomic literature (Domínguez et al., 2006; Stark et al., 2009). A higher taxonomic resolution was not possible due to the scarce taxonomic knowledge of the Mediterranean area (Figueroa et al., 2013) and the small size of most of the organisms (e.g. Gripopterygidae).
Biodiversity indices of Shannon (H’) and Simpson (D’), total abundance, and family richness were determined to describe the changes in macroinvertebrate assemblage between control and impact reach sections.
In order to assess the sensitivity of biotic indices to water extraction, we selected the ChSIGNAL and EPT index. The ChSIGNAL is an adaptation of the SIGNAL index (Chessman, 2003) with benthic macroinvertebrates of Chile (see Figueroa et al., 2007). The EPT index corresponds to the count of families from the Ephemeroptera, Plecoptera, and Trichoptera orders collected at the sampling sites (McKay & King, 2006).
Statistical analysis. To assess the relation between habitat variables and flow (L/s), a Pearson correlation was used (R>0.8; p<0.05). Furthermore, two data matrices were used: 1) aggregated data of all the sites in order to detect a general response of the streams of the area, and 2) separated data of every site to define specific responses to low flow conditions.
Statistical difference between the control and impact reach sections in low flow were calculated with the Student T-test (p<0.05), but the differences between low flow (spring/summer) and high flow (winter) conditions were calculated with ANOVA BACI design (Downes, 2002). In the BACI model for statistical differences, B corresponds to each of the sampling dates in spring or summer, A is the sampling date in high flow period (winter), C refers to control reach sections, and I is the impact reach sections. Therefore, a significant difference in the BA x CI indicated that the change in the environmental parameter is associated with water withdrawals (Downes, 2002).
The relationship between habitat parameters, biotic and diversity indices was established with a Multiple Linear Regression analysis, Akaike Information Criterion, and the Forward/Backward selection in the MASS statistical package (Venables & Ripley, 2002). Then, the most important environmental predictor was determined from the previous selected variables with all-subsets by a regression model in LEAPS R statistical package (p<0.05).
The differences in the macroinvertebrate community assemblage were determined with ADONIS statistical analysis (Anderson, 2001).
Comparisons were made using the Bray Curtis similarity matrix. Previously, abundance data were transformed to Log10 (x+1) in order to reduce the contribution of the families with greater numerical representation in the samples (Anderson et al., 2006; Gauch, 1982). IndVal (Dufrene & Legendre, 1997) established the most important families in terms of the differences between control and impact reach sections. The IndVal method considers the abundance and relative frequency of the different families in each sample in order to assign an indicator value and a probability p derived from the Monte Carlo permutation.
Results
The weirs modified the discharge in all the sampling sites. The highest summer flow reduction was registered in Dehesa (97.7%), while the lowest was in Recinto (26.1%), both in December 2012. In August 2013 (winter period), we observed closed weirs in Marchant and Dehesa; however, it was possible to record some water withdrawals (Table 1). Only water depth (R: 0.85; p<0.05), current velocity (R: 0.84; p<0.05), and conductivity (R: 0.65; p<0.05) showed correlation with the flow measurements. According to the chemical characteristics, Dehesa and Recinto showed oligotrophic conditions in low and high flow periods (< 0.03 mgL-1 NH4+, < 0.2 mgL-1 NO3-, <0.04 mgL-1 PO4 3-). In Marchant the concentration of NO3-(0.384 mgL-1) and PO4 3- (0.13 mgL-1) tends to decrease in the high flow period (0.293 mgL-1 NO3and 0.06 mgL-1 PO4 3-) (Table 2).
Table 2 Concentration of nutrient and suspended solids for the sampling sites on the Itata basin, Chile, in low (March 2013) and high flow (August 2013) hydrological conditions (Values in bold = Under detection limit).
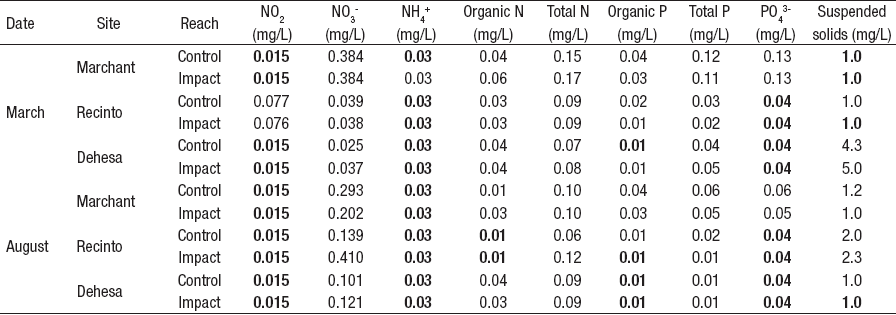
Most of the habitat variables showed variation between control and impact reach sections (Table 3). The coefficient of variation (control <impact) was higher than 10% for depth, current velocity, wetted width/depth ratio, Froude number, richness, abundance, and EPT. The t-test detected statistical differences (p<0.05) for dissolved oxygen, depth, Froude number, current velocity, wetted width/depth ratio, Shannon and Simpson’s diversity (Fig. 2). Neither EPT nor ChSIGNAL biotic indices showed statistical differences between control and impact reach sections (Fig. 2).
Table 3 Mean (
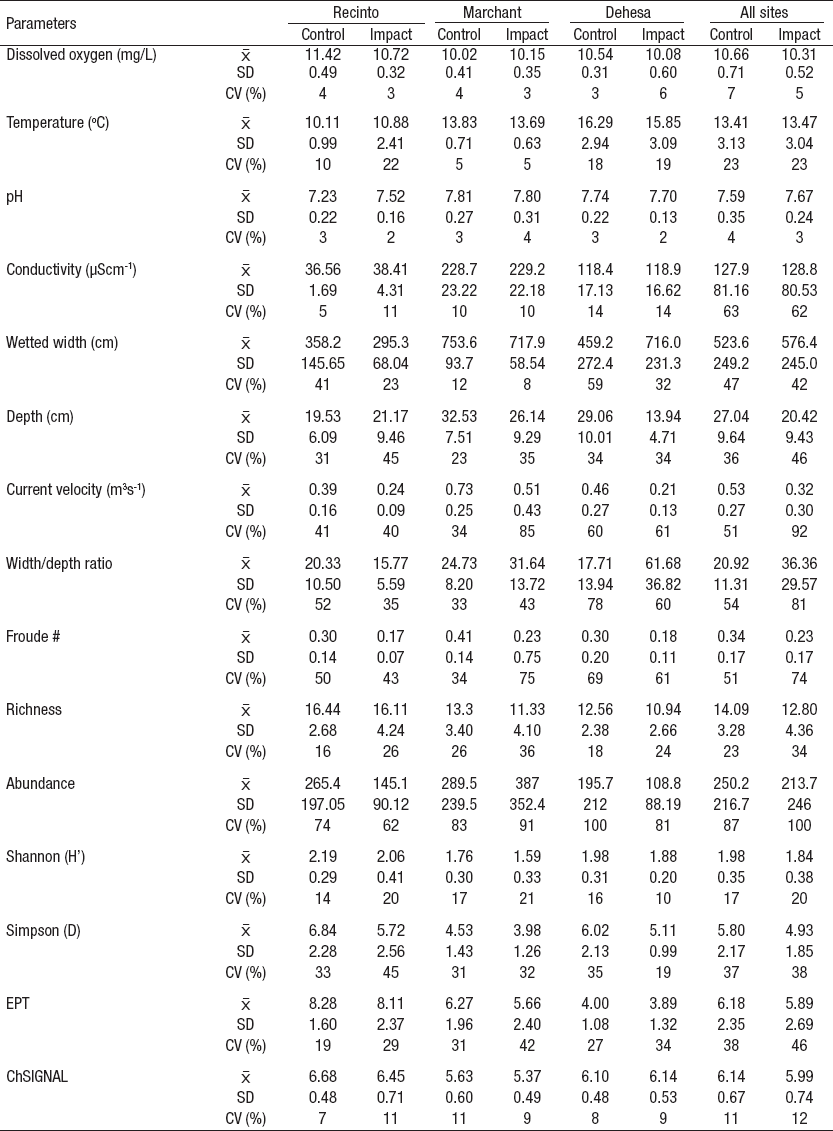
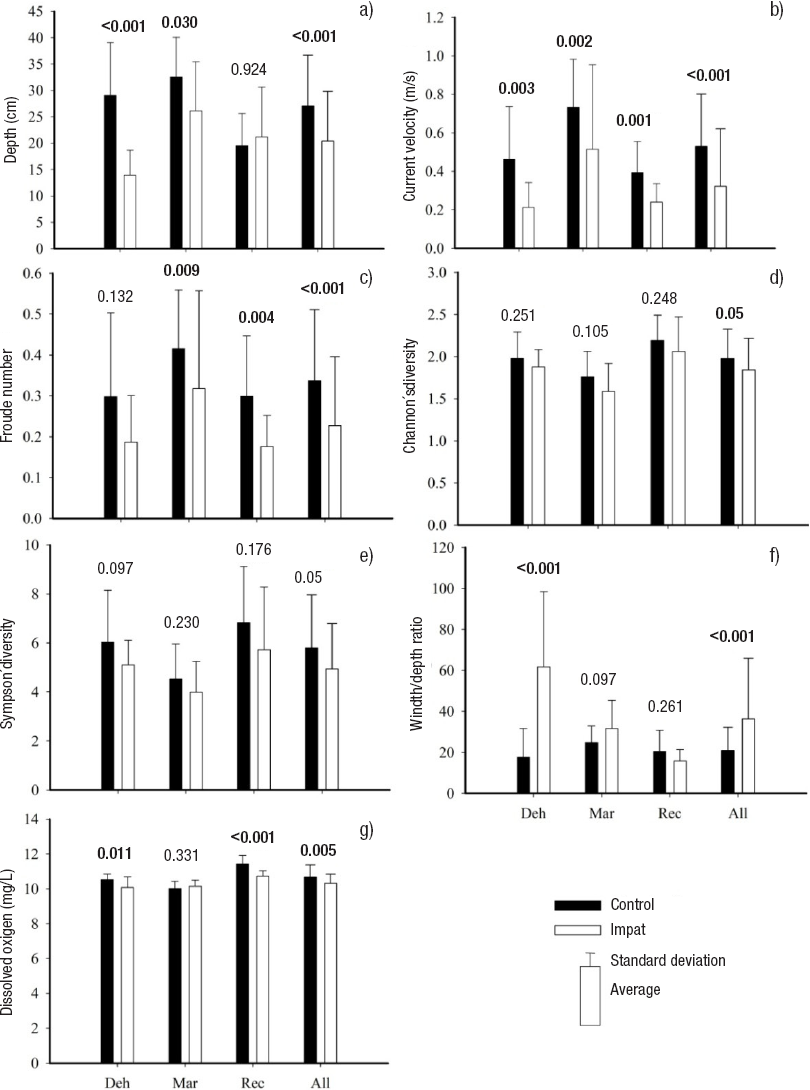
Figures 2a-g Habitat parameters and diversity indices with T test statistically significant differences (p<0.05) between control and impact reach sections in low fflow period on Itata basin, Chile. (Deh = Dehesa; Mar = Marchant; Rec = Recinto; All = aggregation of all the sites).
Comparing low and high flow sampling dates with ANOVA BACI design, only current velocity showed significant differences in 2 different sampling dates (October 2012 and March 2013). No statistical differences were detected for water temperature, wetted width, and hydraulic Froude number in December 2012 (Table 4). According to linear regression models, habitat and chemical parameters were good predictors of community assemblages; however, it was not possible to establish a significative model with the Simpson diversity index. The best significative model (R2 = 0.37) was determined for ChSIGNAL index (pH and DO), while the lower explanatory power of the models was recorded for Shannon diversity (R2 = 0.19) (Table 5).
Table 4 Summary of ANOVA test for BA x CI comparison in control and reach sections of all the sampling sites, in central area of Chile. (Itata basin).
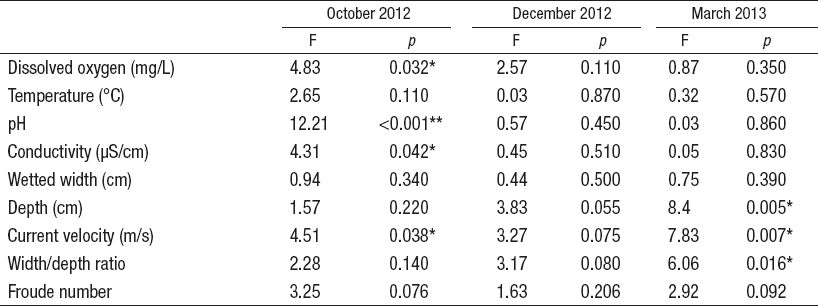
* = p <0.05. ** = p <0.01
Table 5 Multiple linear regression and single best parameter for all the sampling sites during low flow, in central area of Chile (Itata basin).
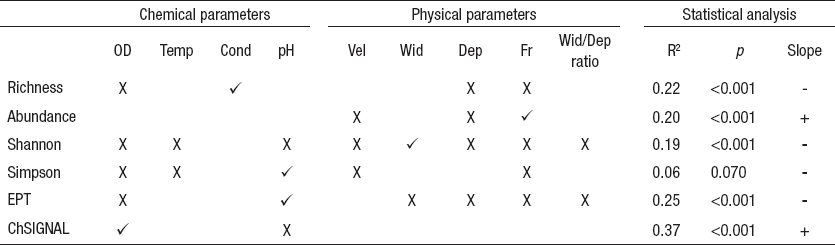
Note: Chemical parameters correspond to dissolved oxygen (OD), temperature (Temp), conductivity (Cond), and pH. Physical parameters are water velocity (Vel), wet ted width (Wid), depth (Dep), number of Froude (Fr), wetted width/depth ratio (WW/Dep). The value of R2 corresponds to the Adjusted R2. The statistical significance of the multiple regression model is denoted (p). The significant parameters of multiple regression are marked (X), as well as the best singular parameter ()
The total abundance of benthic macroinvertebrate was 41133 individuals (3173 individuals/m2), distributed in 10 orders and 49 families. The order with the highest abundance was Diptera (n = 16465), followed by Ephemeroptera (n = 7371), Trichoptera (n = 7122), and Plecoptera (n = 6486). The highest abundance of Baetidae, Leptophlebiidae (Ephemeroptera), Gripopterygidae, and Notonemouridae (Plecoptera) occurred during low flow. Significant differences in macroinvertebrate assemblage were established in October and December 2012 (low and high flow), while in March, differences were determined for the control and impact reach sections (Table 6). The IndVal test determined that Notonemouridae (Plecoptera; IndVal: 0.385, p: 0.004) was a good indicator for impact reach sections, while Diamphipnoidea (Plecoptera; IndVal: 0.630, p: 0.002) or Glossosomatidae (Trichoptera; IndVal: 0.394, p: 0.036) were a good indicator for the control reaches of all the sites.
Discussion
In this study, diversion structures in the rivers produced a contraction of the fluvial habitat, low variation of chemical characteristics, and changes in the macroinvertebrate community assemblage. To our knowledge, this is the first study that determines the impact of irrigation withdrawals in the Chilean rivers, although irrigation agriculture consumes 85% of available water resources and modifies modifies natural flow regimen (Figueroa et al., 2013).
The natural flow has direct influence in the water chemical composition of the rivers (Bunn & Arthington, 2002; Dewson et al., 2007a; Larned et al., 2010). Particularly, it has been reported that scarce water conditions produce temporal and spatial changes in nitrogen compounds (Caruso, 2002; Dahm et al., 2003; Von Schiller et al., 2011), therefore similar trends are expected in rivers with intense agricultural water withdrawals. However, in this study we detected no significant chemical changes in the nutrients that could be related to good river conservation in the area (Debels et al., 2005; Figueroa et al., 2007; Urrutia et al., 2009b). Nor could we find a traditional river damning method that does not promote changes in the ionic composition of the surface waters by anoxic conditions (Ahearn et al., 2005; Friedl & Wüest, 2002).
Dissolved oxygen was the only parameter that showed statistical differences between control and impact sampling sites. Several authors indicate that DO concentration decreases in river water withdrawals (Dewson et al., 2007b; McKay & King, 2006) related to reduced turbulence conditions or increasing heating exposure (Brown et al., 2012; Chessman et al., 2010; Dewson et al., 2007b). At the sampling sites, dense riparian forest protected the rivers from increased heating, and low depth/wide values can explain the lack of differences in the thermal regimen (Pedreros et al., 2013).
The principal indicator of turbulence perturbation in our study was the Froude number. The Froude number is closely related to current velocity and water depth, and is considered a good indicator of the hydraulic and microhabitat characteristics for the benthic macroinvertebrates (Brooks et al., 2005; Allan & Castillo, 2007). Although a high Froude number has been related to reduced macroinvertebrate abundance (Brooks et al., 2005), the positive relationship with the abundance in our sampling sites could be explained by the trade-off between the good oxygen conditions and the energy budget to keep oxygen suitable habitats for macroinvertebrates. In this sense, extreme current velocity during floods leads to movement of the river bed with direct influence in the macroinvertebrate community (Death & Zimmermann, 2005; Schwendel et al., 2010), but harsh hydraulic conditions do not promote the presence of high abundance of benthic macroinvertebrates.
According to Growns and Davis (1994), turbulent conditions are also necessary for the suspended food supply ingested by passive filterer macroinvertebrates. According to Walters and Post (2011) and Wills et al. (2006), a 90% reduction in the available flow has been correlated with a decrease of filter feeding macroinvertebrates and an increase of predatory species. Although we did not detected changes in the functional feeding groups in our sampling sites, we expected a similar tendency in the more arid zones of Chile.
In our sampling sites, temporal variation of water abstraction and high flow period promotes a reset opportunity as the macroinvertebrate community recolonizes the sampling sites. During high flow periods, the principal mechanism for site recolonization is active drifting, widely used for the environmental assessment of the flow disturbance and river health (Death et al., 2009; Dewson et al., 2007b; James et al., 2009). In Chile, active drifting behavior is related to a circadian cycle (Figueroa et al., 2000), but more information is required regarding this dispersal mechanism as a functional bioindicator technique.
In this study, we did not detect statistical differences in the abundance or family richness indicators. However, the presence of statistical differences in the Shannon and Simpson indices for the accumulative abundance of benthic macroinvertebrates could reflect some sensitive responses to low flow conditions. In this sense, EPT families were defined as a good bioindicator in controlled (Death et al., 2009) or non controlled water withdrawals (Wills et al., 2006). In contrast, other studies determined the importance of specific habitat characteristics in order to detect environmental impacts through the EPT index (McKay & King, 2006)
The use of biotic indices is a common practice to determine the environmental impacts on surface waters (Bonada et al., 2006; Rosenberg & Resh, 1993). In Chile, the CHSignal demonstrates sensibility to water pollution in the semiarid rivers of northern Chile (Alvial et al., 2012) or in the Chillán River, a tributary of the Itata River (Figueroa et al., 2007). Similar trends were determined for other biotic indices like RIVPACS (Armitage & Petts, 1992) or AUSRIVAS (Chessman et al., 2010).
We believe that the communities in the study area have suffered this type of impact for many years, so they have developed strategies to survive in adverse environmental conditions (Miller et al., 2007). In Mediterranean climate areas, seasonal predictable flow variations are closely related to macroinvertebrate biological or behavioral characteristics (Bonada et al., 2007), which give us the opportunity to record the magnitude of water abstraction (Dolédec & Statzner, 2010; Menezes et al., 2010). In contrast, Walters and Post (2011) indicated that trait and classical taxonomic approach have the same power to detect habitat changes in low flow condition, but they do not discard evaluating specific traits like high crawling or armoring and resistance characteristics of macroinvertebrates. In Chile, the scarcity of available biological and taxonomic information regarding several macroinvertebrates families limits the improvement of bioindicator techniques (Stark et al., 2009). For example, the Gripopterygidae family has 55% undetermined species (Vera & Camousseight, 2006), but Growns and Davis (1994) determined positive correlation of this family with the Froude number in Australian rivers. In addition, the conservation of endemic macroinvertebrates in low order rivers of Mediterranean Chile requires bioindicator techniques to be improved in accordance to the expected low rainfall and increased temperature (Figueroa et al., 2013; Pedreros et al., 2013).