Introduction
In tropical ecosystems, pioneer tree species are of particular interest for ecological restoration due to their capacity to compete with aggressive colonization of herbaceous plants that demand light; thus, these trees catalyze forest succession (Lamb et al., 2005). The Lacandon and Ch'ol Mayan indigenous groups of the tropical Lacandon Jungle of southern Mexico have long used certain tree species to promote natural regeneration of the high evergreen forest after it has been used for slash and burn agriculture. Specifically, they use the tree Ochroma pyramidale (Cav. Ex Lam.) Urb., Malvaceae, to accelerate recovery of degraded soil (Levy-Tacher & Golicher, 2004). This tree is native to the region, it is present in secondary vegetation, and the Mayan peasants intentionally plant it in their agricultural plots to regenerate soil as it requires minimal maintenance. It also used to counter invasion by bracken fern (Pteridium aquilinum) as it shades out and eventually kills the fern (Levy-Tacher et al., 2015).
Ochroma pyramidale is a pioneer tree species with a short life cycle, and the density of its wood is the lowest of that produced on commercial plantations (0.17 g cm−3). Its leaves have a rapid turnover rate and are the largest of all neotropical trees, generating a thick layer of leaf litter (Coley, 1983; Francis, 1991; Park & Cameron, 2008). The generation of this thick layer is likely the result of its high content of recalcitrant C (condensed tannins, lignin), negatively correlated with loss of litter mass (Hättenschwiler & Bracht, 2010). This species presents episodes of rapid growth, quickly reaching great heights and a large basal area (Aide et al., 2001; Cusack & Montagnini, 2004; Denslow & Guzman, 2000; Finegan 1996; Park & Cameron, 2008; Saldarriaga et al., 1988). The relatively open canopy of populations of O. pyramidale allows light to reach the understory, promoting establishment and growth of tree seedlings (Poorter, 1999; Whitmore, 1978).
The specific rate of production of the leaf litter of this species and those factors controlling its decomposition, such as its foliar chemistry and the activity of soil fauna, have not been quantified. Understanding these variables will in turn allow a better understanding of leaf litter accumulation.
This study had three objectives: 1) to quantify the rate of production of leaf litter; 2) to estimate how long the leaf litter layer persists in the soil and the depth layer in sites planted with O. pyramidale after three, eight, and 16 years; and 3) to estimate the effect of soil fauna on decomposition of this leaf litter and its chemical characteristics in an open site and in a plantation of O. pyramidale over the course of one year.
Despite the fact that O. pyramidale is a pioneer species (Pearson et al., 2003), its leaf litter does not disappear within few months; rather, it is likely that the chemical composition of its leaf litter prevents it from rapidly being consumed by soil fauna, allowing it to accumulate in a thick layer.
Materials and methods
Site description
This study was carried out in Lacanha Chansayab, Chiapas, Mexico (16( 47′ N; 91( 09′ W), which has an altitude of 350 m. a. s. l. Climate is warm-humid with a mean annual temperature of 24 °C and a mean annual precipitation of 2814 mm (Servicio Meteorológico Nacional-Comisión Nacional del Agua [SMN-Conagua], 2022). Monthly distribution of rainfall and temperature during the study period are shown in Figure 1. The predominant soil is humic acrisol (World Reference Base, 2015). While the predominant vegetation was previously tropical evergreen forest, currently the area is a mosaic of patches of secondary forest, mature vegetation, grassland, and milpas (diversified maize fields) (personal observation).

Source: Author’s own elaboration.
Figure 1 Ombrothermic diagram recorded 2005-2006 in Mexico’s Federal Electrical Commission’s Lacantun weather station, approximately 70 km from Lacanha Chansayab, Chiapas, Mexico.
The experimental site -which was last used for agriculture 35 years ago and later invaded by Pteridium aquilinum- was prepared for planting with O. pyramidale with the aim of controlling growth of ferns. The site included four 8 m x 8 m plots planted with O. pyramidale and four adjacent 8 m x 8 m control plots not planted with O. pyramidale (Levy-Tacher et al., 2015). Additionally, we selected three sites sown with O. pyramidale in different years by local peasants (Figure 2).
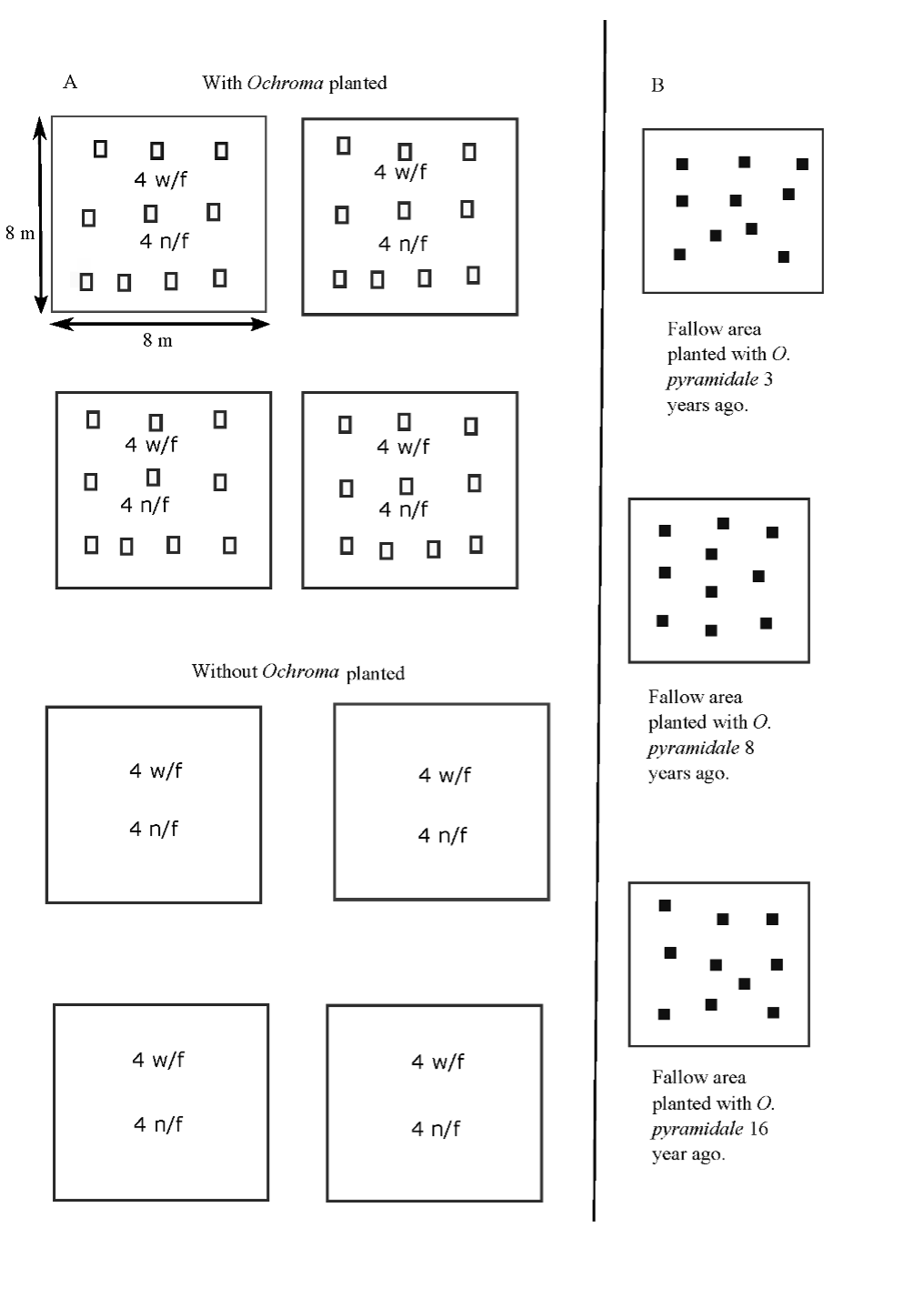
Source: Author’s own elaboration.
Figure 2 a) Schematic view of plots used to obtain net productivity of litter and to estimate decomposition of O. pyramidale leaf litter. Hollow squares = leaf litter traps; w/f nylon mesh bags which allow soil mesofauna to pass; n/f nylon mesh bags which do not allow soil mesofauna to pass. b) The three fallow areas were planted with O. pyramidale trees in different years. Solid squares = 1 m2 areas from which accumulated leaf litter was collected and depth of leaf litter layer was measured. Illustration is not to scale.
Production of leaf litter
a) Net productivity of leaf litter
Ten leaf litter traps measuring 1 m in height by 80 cm in diameter were placed at 10 points within each of the four 8 m x 8 m plots planted with O. pyramidale (Figure 2). In order to calculate the litter weight by month, the content of each trap was removed monthly and allowed to dry at ambient temperature until a constant weight was reached.
b) Biomass and depth of leaf litter layer in plots under recovery previously planted with O. pyramidale
Appart from the experimental plot described in section A, three fallow areas were selected -each one with three, eight, and 16 years of fallow-, which had previously been planted with O. pyramidale trees (Levy-Tacher & Golicher, 2004; Vleut et al., 2013). In each of these plots, the accumulated leaf litter was collected from ten 1 m2 randomly chosen areas (Figure 2). Leaf litter collected was dried at ambient temperature until a constant weight was obtained. Depth of the leaf litter layer was measured from the soil surface to the surface of the leaf litter with a measuring tape at 10 points within each 1 m2, and then it was recorded.
c) Decomposition and chemical characteristics of leaf litter
To estimate decomposition of O. pyramidale leaf litter, a complete balanced factorial design with three factors was used: presence or absence of soil mesofauna, presence or absence of O. pyramidale plants, and four dates of collection of the bags with leaf litter over the course of one year. Nylon mesh bags measuring 20 cm x 20 cm with 3 mm openings (which allow soil mesofauna to pass) and others with openings < 1 mm (which do not allow soil mesofauna to pass) were used, previously placing 10 g in dry weight of leaf litter from recently fallen leaves of O. pyramidale trees. Four bags containing the treatment with soil mesofauna and four bags containing the treatment without soil mesofauna were located on the soil surface of each of the four plots (repetitions of the treatment) planted with O. pyramidale and on those without it (Figure 2). Every three months, one bag was removed from each repetition of each soil mesofauna treatment. In the laboratory, the leaf litter was carefully extracted so that none of it could be lost, then it was weighed fresh, and later it was dried at ambient temperature until reaching a constant weight. A total of 64 bags were used: 32 for the control and 32 for the treatment planted with O. pyramidale.
At the start of the experiment, the content of carbon (C), nitrogen (N), phosphorus (P), calcium (Ca), magnesium (Mg), cellulose, and lignin was determined for the leaf litter collected in the bags. For each date of harvesting, an analysis was carried out of the lignin, cellulose, and ADF content of the remaining leaf litter.
For the C and N analyses, a LECO-1000 autoanalyzer (LECO Corporation, Michigan US) was used. The concentration of lignin, cellulose, and ADF was obtained through incineration at 550 (C, acid digestion, and oxidation with potassium permanganate according to the Van Soest method.
Data on remaining leaf litter biomass and concentration of macromolecules was analyzed through an Anova factorial analysis, and Shapiro-Wilk and Bartlett tests were used to test the suppositions of normality and homocedasticity, respectively. In the case of data for lignin and cellulose, the arcsine transformation was used to comply with the suppositions of the model. For statistical analysis, we used the 4.1.1 version of the R software (R Core Team, 2021). A linear model was applied to calculate the constant (k) for the annual rate of decay of the remaining dry mass, using the following equation:
where Xt is the remaining mass (g) at time t (days), X0 is the initial leaf mass, and k is the decomposition rate constant (yr-1) (Wider & Lang, 1982). To calculate the time it took for the leaf litter to decompose 50% and 99%, the following equations were used:
where t is time (years), and k is the decomposition rate constant (yr-1) (Olson, 1963). All data are presented in their original scale of measurement.
Results
Net productivity of leaf litter and biomass and depth of leaf litter layer in plots under recovery
Data for productivity were obtained over 11 months, resulting in an average of 1.1 kg/m2 (11 Mg/ha) in dry weight. Figure 3 shows the distribution of productivity throughout the year. The data for November and December are likely underestimated, as some leaf litter traps collapsed due to strong winds.
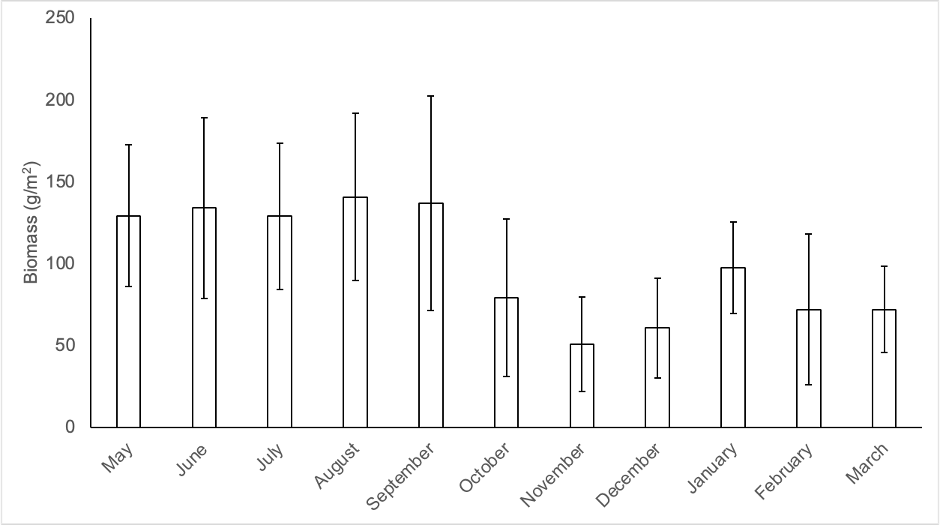
Source: Author’s own elaboration.
Data obtained from the plots previously planted with O. pyramidale are shown in Table 1.
Figure 3 Production of Ochroma pyramidale leaf litter biomass during the study period. Bars represent mean of n = 40 traps per month (1 SD.
Table 1 Biomass of accumulated leaf litter and depth of leaf litter layer in sites planted with Ochroma pyramidale three, eight, and 16 years before collection. Values are the mean of n = 10 ((1SD).
Age of fallow
(years) |
Accumulated
biomass of leaf litter (Mg/ha) |
Depth (cm) |
3 | 6.2 (2.43) | 22.5 (1.51) |
8 | 16.3 (2.7) | 16.1 (2.11) |
16 | 6.5 (1.59) | 9.4 (1.44) |
Source: Author’s own elaboration.
Decomposition and chemical characteristics of leaf litter
The results of the Anova factorial analysis did not indicate any interaction among the principal factors; time of permanence of leaf litter in the soil explained most of the variation (F = 11.89 with 3 and 58 degrees of freedom, p < 0.001), while soil mesofauna and presence/absence of O. pyramidale trees were not statistically significant. The greatest loss of biomass of O. pyramidale leaf litter occurred between the third and sixth month of the experiment; average loss was 37% and was almost constant after the first six months (Figure 4). The annual decay constant k value was 0.93 (R2 = 0.52).

Source: Author’s own elaboration.
Figure 4 Loss of biomass in experiment of decomposition of Ochroma pyramidale leaf litter over 11 months. Values at each point are the mean of n = 16 ( 1 SD.
The initial chemical composition of O. pyramidale leaves was: C = 47.1%, N = 1.02%, P = 0.2103 mg/kg, K = 7515.45 mg/kg, Mg = 891.82 mg/kg, and Ca = 3068.29 mg/kg.
With respect to the macromolecules making up the leaf litter, the Anova test indicated that the average amount of cellulose in the bags that allowed soil mesofauna to pass was less (10.6%) than that of those that did not allow soil mesofauna to pass (12.3%; F = 5.86 with 1 and 58 degrees of freedom, P = 0.018). The mesofauna found principally belonged to Acari and Collembola, and to a lesser extent to Isoptera. Concentration of cellulose also varied according to the time in which the bags were withdrawn from the soil (Table 2). Concentrations of lignin and ADF were not altered by the presence of soil mesofauna, nor did they vary according to the site where they decomposed. Only the time in which the bags remained in the soil explained changes in concentrations (Table 2).
Table 2 Concentration of lignin, cellulose, and ADF, and the value of the lignin/cellulose ratio during the experiment of decomposition of Ochroma pyramidale leaf litter. Values are the mean of n = 16 ((1SD) for each date. Different letters in the same row indicate statistically significant differences (p < 0.01).
Biomolecule % | Start1 | 3 months | 6 months | 9 months | 11 months |
Lignin | 42.07 | 52.00(3.9)a | 46.12(8.6)b | 55.02(4.7)a | 55.32(5.5)a |
Cellulose | 18.59 | 16.06(2.8)a | 9.01(2.9)b | 11.94(2.9)c | 8.88(2.2)b |
ADF | -- | 69.62(2.4)a | 57.77(9.8)b | 70.00(3.2)a | 67.18(4.7)a |
Lig/Cel | 2.25 | 3.34(0.6)a | 5.34(0.93) b | 4.89(1.32)b | 6.62(1.80)c |
1Initial data were not compared with the rest of the data.
Source: Author’s own elaboration.
Discussion
Annual leaf litter productivity of Ochroma pyramidale ranges from 6 Mg/ha to 11 Mg/ha, which concords with that found for other tropical species planted with the purpose of restoration (Goma-Tchimbakala & Bernhard-Reversat, 2006; Montagnini et al., 1993).
The time it takes for the leaf litter produced to be reincorporated into the soil depends on its rate of decomposition; in this case, the constant k value (0.93) falls within the range reported for other tropical and temperate species with a slow decomposition rate and is correlated with a high C:N ratio and high lignin content (Zhang et al., 2008). Table 3 compares the rate of leaf litter decomposition of the species studied with that of other tropical forest species. According to the calculations (t(0.5)) and (t(0.99)) of biomass loss, O. pyramidale will lose 50% of its leaf litter biomass in 270 days and will require approximately five years to lose 99% of its biomass. Due to its slow decomposition rate, O. pyramidale leaf litter tends to accumulate, forming a thick layer (Table 3), which requires many years to decompose.
Table 3 Comparison of biomass loss of leaf litter of different tropical tree species with that of O. pyramidale.
Tree species | Vegetation
type |
Time in which
biomass was decomposed (days) |
% decomposition
of leaf litter biomass |
C:N ratio | Successional stage |
Reference |
Ficus yoponenis Desv. | Tropical evergreen forest | 100 | < 200 | 17.5* | Pioneer | Álvarez-Sánchez & Becerra-Enríquez (1996) |
Poulsenia armata (Miq.) Standl | Tropical evergreen forest | 100 | < 200 | 4-5** | Non- pioneer | Álvarez-Sánchez & Becerra-Enríquez (1996) |
Pithecellobium dulce (Roxb) Benth | Tropical dry forest | 67 | 183 | 18.6 | Pioneer | Sánchez-Solis (2010) |
Ochroma pyramidale (Cav. Ex Lam.) Urb. | Tropical evergreen forest | 37 | 180 | 46.1 | Pioneer | This study |
Manilkara zapota (L.) P. Royen | Tropical semi-evergreen forest | 23 | 190 | 80 | Non- pioneer | Xuluc-Tolosa et al. (2003) |
*Estimated from protein and cell wall values reported by Milton (1979)
**Data from Kattge et al. (2020)
Source: Author’s own elaboration.
In general, litter decomposition is driven by climate, quality of litter and substrate, and soil fauna (Aerts 1997; Frouz et al., 2015); and when the litter is in the late decomposition stages, the lignin concentration has more influence on decomposition (Berg, 2000; Hättenschwiler & Bracht, 2010). Decomposition of biomass per unit of time is frequently greater in leaf litter with a high nutrient availability but is reduced with an increase in lignin or polyphenols (Preston et al., 2000; Trofymow et al., 2002), as would be the case of the species studied. O. pyramidale has an initial C:N ratio of 46.17, which indicates that its leaf litter has a slow rate of decomposition; leaf litter with a C:N ratio less than 20 is expected to have a rapid rate of decomposition (Cadish & Giller,1997). Considering this, and the conceptual model of transformation of litter into the soil organic matter proposed by Gunina & Kuzyakov (2022), the leaf litter of O. pyramidale may initially be used by microbes as an energy source (most likely during the first six months of decomposition) and later may serve as an energy deposit to be used by other microbial communities which consume nutrients stored in the soil organic matter.
Due to the C:N ratio (46.17) and the initial N content (1.02) of O. pyramidale, its leaves are slow to decompose, which could also be the case of other tropical pioneer tree species such as Cecropia obtusifolia Bertol (C:N = 37.7 and N = 1.0) (Wieder et al., 2009), as well as of secondary tree species; the leaves of secondary species also have greater concentrations of C and lignin, and less N than those of primary species, hence, decomposing more slowly (Vasconcelos & Laurance, 2005). It is likely that the chemical composition of O. pyramidale leaf litter prevents it from being consumed by degrading soil mesofauna, and possibly most of its decomposition is carried out by specialized groups of microorganisms such as fungi, which degrade lignin (Krishna & Mohan, 2017). Reduction in the percentage of cellulose (Table 2) may be partially attributed to the action of soil fauna as well as to the high level of solubility in water of cellulose as compared to lignin. In biomes dominated by herbs and grasses, soil fauna may consume up to 50% of the litter. However, in biomes dominated by trees, which produce litter with a high C:N ratio and a higher lignin content, the consumption rate decreases (Heděnec et al., 2020). As previously indicated, fungi could be a significant group of microorganisms decomposing O. pyramidale litter. Meanwhile, for other species with a high C:N rate, such as Quercus petrea (Matt.) Liebl., fungi are responsible for 35% of loss of litter dry mass (Steffen et al., 2007), and they have been reported as principal drivers of lignocellulose degradation (Wang et al., 2020).
The characteristics of O. pyramidale leaf litter and the results of the present decomposition experiment indicate that soil mesofauna do not alter loss of biomass, that they reduce the concentration of cellulose, and that they do not modify the concentration of other macromolecules of leaf litter. It is likely that abiotic factors (physical weathering) also play a role in decomposition of Ochroma leaf litter, as has been shown in the decomposition process of other species in different environments (Montaña et al., 1988). O. pyramidale leaf litter likely remains for over two years before being completely integrated into the soil, which contributed to explaining the biomass and thickness of the leaf litter layer (Table 1) in sites planted with this species in different years. These results complement those of other studies (Diemont et al., 2006; Douterlungne et al., 2010; Levy-Tacher & Golicher, 2004; Vleut et al., 2013) in which the O. pyramidale leaf litter layer was identified to be a selective physical barrier which allows for establishment of seeds of some species and prevents establishment of others. The large size of O. pyramidale leaves (13 cm × 13 cm to 35 cm × 35 cm), as well as its rapid growth, high rate of leaf turnover, and the chemical composition of its leaves as reported here, contribute to forming a thick layer of leaf litter, which may remain for over 10 years. This may impede the seeds of early succession plant species from reaching the soil surface, in turn impeding their colonization (Sayer 2006; Vázquez-Yanes & Orozco-Segovia, 1992). Late succession tree species have larger seeds with sufficient reserves to emerge and survive under conditions of little luminosity and, therefore, a thick layer of leaf litter -such as that observed and analyzed in the present study- could favor development of such species (Seiwa & Kikuzawa, 1996). Thus, species for which the chemical composition of their leaf litter result in slow decomposition, which are planted by peasants with the purpose of recovering soil fertility, could also facilitate succession.
Conclusions
After one year of decomposition, initial biomass of leaf litter of Ochroma pyramidale is reduced on average by 37%. This species’ low annual decay constant k value (0.93) -in conjunction with its high C:N ratio (46.17), low initial N concentration (1.02 %), high lignin content (42 %), and low rate of activity of mesofauna- explain their slow decomposition, which could explain the persistence of a deep layer of litter in plots planted with this species after five or more years. The chemistry of the leaf litter of O. pyramidale, which is unlike that generally expected for pioneer species, contributes to the formation of a thick layer of leaf litter and may indirectly facilitate germination of late succession tree species.
Conflicts of interest
The authors declare they have no conflicts of interest.