Introducción
Sesame (Sesamum indicum L.) (also known as ajonjoli, goma, sesame, til, and hu ma in different languages such as Spanish, Japanese, French, Hindi, and Chinese, respectively) is an erect annual herb belonging to the Pedaliaceae family, which was used as food in India 5000 years ago (Sharma et al., 2021). Sesame seed was an important oil crop in Asia and Africa, where it was cultivated for centuries due to its high-protein and edible oil content. Currently, this crop is cultivated in Sudan, Myanmar, India, China, the Mediterranean, Japan, Africa, USA, México, and other American countries (FAO-STAT, 2020). Lipids (45%-65%) is the principal component in sesame seed, followed by protein (19%-35%) and carbohydrates (14%-20%). The principal fatty acids in sesame oil were oleic acid (35%-50%), linoleic acid (31%-40%), palmitic acid (9%-10%), and stearic acid (7%-9%), with considerable unsaponifiable fractions with presence of sesamin, sesamolin, sterols, and tocopherol (Sharma et al., 2021). The protein fraction in sesame contains mainly α-globulin and β-globulin fractions (80%-90% of the total protein), presenting around 95% of 13S globulin, similar to soybean (Glycine max) 11S globulin, with more hydrophobic properties, limiting its use in food formulation such as beverages and fluids (Anilakumar et al., 2010; Sharma et al., 2021). According to this, protein modification is needed before using it in food production (Anilakumar et al., 2010). The sesame protein is rich in sulfur-containing amino acids, valine, and tryptophan, but it is deficient in lysine, showing a good essential and no-essential amino acid balance (Sharma et al., 2021). Also, sesame is rich in minerals like calcium, cooper, phosphorous, iron, magnesium, zinc, and manganese, as well as in vitamin B1 (Anilakumar et al., 2010). On the other hand, the presence of several anti-nutrients compounds such as saponins, lectin, enzyme inhibitors, tannins, and oxalates has been reported in sesame seed, which affect the human metabolism (Nikmaram et al., 2017; Sharma et al., 2021). However, these anti-nutritional compounds are reduced with the application of thermal process (Sharma et al., 2021). Some phenolic acid like ferulic, vanillic, cinnamic, protocatechuic, and gallic acids, as well as sesamin sesamol, sesamolin gucosides, and tocopherol have been reported in sesame seed. These biological compounds have been reported to show important biological activities like anti-inflammatory, anti-oxidative, anti-mutagenic, and others (Ben-Othman et al., 2015; Mohdaly et al., 2013; Sharma et al., 2021).
Sesame is used for oil extraction, since it has an excellent fatty acid profile, mainly the unsaturated type (Sharma et al., 2021). Derived from the oil extraction, a residual sesame paste (sesame by-product cake) is generated, which has good levels of nutrients such as proteins, vitamins, minerals, as well as phytochemicals, among which dietary fiber and phenolic compounds stand out. It is necessary to take advantage of this sesame by-product for the development of products of high nutritional and nutraceutical value (Anilakumar et al., 2010). Some authors reported the use of sesame by-product flour (defatted sesame flour) as an ingredient in foods formulations such as bread, where wheat flour was partially replaced until 20% by sesame by-product flour, finding an increase in the proximal (Shima et al., 2019) and physical (Ahemen et al., 2018) properties of breads, when sesame by-product was increased; cookies, where sesame by-product cookies showed similar sensorial properties and higher proximal composition than wheat cookies (Martínez et al., 2021); biscuits, where wheat flour were replaced by 50% of sesame by-product, finding an improve on protein, fat, mineral, and crude fiber, and showing similar values of acceptability as biscuit control (Prakash et al., 2018); kefir, where the addition of sesame by-product flour showed an increase of nutritional value and the stability of the product, which mean a high storage stability (Quin et al., 2021); expanded extrudates snacks, where sesame by-product were added until 20% to improve the nutritional properties of corn expanded snacks, finding that the proximal composition value increased, whereas physical properties of expanded extrudates snacks decrease with sesame by-product flour increment (do Nascimento et al., 2012); among others.
Extrusion technology is a quickly cooking process that improve the nutritional and nutraceutical composition of foods, enhancing the protein and starch digestibility, modifying the lipid content, increasing the bioactive compounds retention, inactivating microorganisms and enzymes, and modifying some volatile flavor components and dietary fiber content. Extrusion is an efficient process because the energy consumption and water pollution are minimized, being a highly efficient alternative technology to develop several food products such as ready-to-eat breakfast cereals, novel ingredients, functional foods, cereal-based snacks, among others (Grasso, 2020; Patil & Kaur, 2018). Extrusion process has the potential to develop flours with excellent techno-functional properties, with minimal degradation of nutrients and bioactive compounds, and with high digestibility of starch and protein; therefore, they can be used as ingredients for the development of functional foods (Gómez-Favela et al., 2021).
The aim of this work was to optimize the extrusion process to find the better combination of extrusion temperature (ET)/screw speed (SS) to produce optimized extruded sesame by-product flour (OESBF) with high values of water absorption index (WAI), water solubility index (WSI), in vitro protein digestibility (IVPD), total phenolic compounds (TPC), and antioxidant activity (AoxA).
Materials and methods
Materials
Whole sesame (Sesamum indicum L.) seeds were purchased in El Saladito, Elota, Sinaloa, México (geographic coordinates Latitude: 23.8833, Length: -106.85). This work was carried out in the Chemical and Biochemical Laboratory of Universidad Politécnica del Mar y la Sierra and in the Bioprocess and Functional Foods Laboratory from Facultad de Ciencias Químico Biológicas of Universidad Autónoma de Sinaloa. The seeds were cleaned and stored in airtight containers at 4 °C until use.
Production of extruded sesame by-product flours (ESBF)
The extruded sesame by-product flours (ESBF) were prepared according to León-López et al. (2019) for extruded defatted chia flour with modifications. First, sesame seed were defatted before extrusion process using a screw press, obtaining the sesame by-product flour (It is due to that high moisture and oily materials that sesame seed cannot be processed by the extruder used in this work). The sesame seed was twice passed through the screw press to reduce the lipid content to the maximum level allowed by the extruder. Then, portions of 250 g of sesame by-product flour were mixed with distilled water until 23% moisture content was reached; this value was obtained by preliminary studies using as reference some values reported by Gómez-Favela et al. (2021), Félix-Medina et al. (2020), León-López et al. (2019), and Espinoza-Moreno et al. (2016). The conditioned sesame by-product flours were stored (4 °C-8 °C/12 h) to homogenize the moisture content of the sample and were tempered (25 °C/1 h) to prevent that the sample could stick to the barrel wall of extruder. The portions of conditioned sesame by-product flours were fed to the extruder and underwent various combinations of extrusion temperature/screw speed (Table 1). A single screw extruder (20 DN, model 8-235-00, Brabender, Duisburg, Germany) with 19 mm screw diameter, 20:1 length to diameter ratio, 1:1 nominal compression ratio, and 4 mm dice-opening was used to obtain the extruded samples. The extruder features three heating zones which were maintained at the same extrusion temperature in accordance with the experimental design. The extruded, obtained from each of the 13 treatments, were cooled, ground in a hammer mill with a millimeter mesh, packed in plastic bags, and stored (4 °C). WAI, WSI, IVPD, TPC, and AoxA were evaluated in the ESBF as response variables.
Table 1 Experimental design (13 treatments) and experimental results of water absorption index (WAI), water solubility index (WSI), in vitro protein digestibility (IVPD), total phenolic compounds (TPC), and antioxidant activity (AoxA) from extruded sesame by-product flour (ESBF).
Process variables1 | Response variables2 | ||||||
Treatment | ET (°C) | SS (rpm) | WAI | WSI | IVPD | TPC | AoxA |
1 | 68 | 78 | 1.40235 | 9.44888 | 74.5002 | 474.071 | 1940.842 |
2 | 152 | 78 | 1.31281 | 10.1368 | 78.4643 | 562.712 | 2062.192 |
3 | 68 | 212 | 1.36781 | 9.73308 | 76.5564 | 360.835 | 1811.280 |
4 | 152 | 212 | 1.35436 | 9.94636 | 73.45 | 674.450 | 1972.999 |
5 | 50 | 145 | 1.39111 | 9.21368 | 75.472 | 436.180 | 1830.897 |
6 | 170 | 145 | 1.33226 | 10.0187 | 75.3956 | 683.270 | 1908.501 |
7 | 110 | 50 | 1.34274 | 9.92736 | 77.3004 | 440.042 | 2118.919 |
8 | 110 | 240 | 1.38247 | 10.3211 | 75.3423 | 429.022 | 1931.903 |
9 | 110 | 145 | 1.37003 | 10.2464 | 77.5101 | 567.232 | 1928.337 |
10 | 110 | 145 | 1.35504 | 10.236 | 76.9585 | 597.205 | 1887.978 |
11 | 110 | 145 | 1.36697 | 10.2061 | 77.56 | 623.330 | 1895.018 |
12 | 110 | 145 | 1.36483 | 10.0752 | 77.2166 | 545.703 | 1877.782 |
13 | 110 | 145 | 1.35561 | 10.2409 | 77.3000 | 570.046 | 1847.279 |
1ET = Extrusion temperature; SS = Screw speed; 2WAI = Water absorption index (g of gel/g sample); WSI = Water solubility index (%); IVPD = In vitro protein digestibility (%); TPC = Total phenol compounds (mg of gallic acid equivalents [GAE]/100g sample); AoxA = Antioxidant activity (µmol of Trolox equivalents [TE]/100g sample).
Source: Author's own elaboration.
Water absorption index (WAI) and water solubility index (WSI)
Water absorption index and water solubility index were evaluated according with Milán-Carrillo et al. (2012). A flour sample (2.5 g) was mixed with 30 ml of distilled water at room temperature (25 °C inside laboratory) in a centrifuge tube previously weighted; the mixture was shaken for 30 min and then centrifuged at 3000xg/25°C/10 min. The supernatant was decanted in a glass vessel and the precipitated was weighted. WAI was calculated by dividing the grams of gel formed by the 2.5 g of sample used, resulting in the amount of gel that can be formed from 1 g of sample. The supernatant obtained after water absorption index assay was decanted in a crystal vessel, evaporated in a stove at 80 °C/12 h, and weighted. WSI was expressed as percentage of dry solid, as indicated by the following equation:
In vitro protein digestibility (IVPD)
The IVPD assessment was developed according with León-López et al. (2019). An aliquot (5 ml) of a multi-enzyme solution (pancreatic trypsin, bovine pancreatic chymotrypsin, porcine intestinal peptidase, and distilled water, pH = 8.0) was mixed with 50 ml of the protein suspension (6.25 g of protein/L, pH = 8.0) employing constant stirring in a water bath at 37 °C. The pH was measured after 10 min, and the following equation was used to evaluate IVPD:
where X = pH after 10 min. The pH assay was carried out in triplicate.
Extraction of free and bound phytochemicals
The extractions were made according to Rahman et al. (2017), with slight modifications, where acetone and diethyl ether solvents were replaced by ethanol and ethyl ether in the extraction of free and bound phytochemicals, respectively; also, ultrasound technique was not used in extraction. Extraction of free phytochemicals was performed twice with 80% ethanol, and the recovered supernatant was rotary evaporated, collected with 2 ml of methanol and stored at -20 °C until use. The bound phytochemicals were carried out with ethyl acetate from the precipitate of the ethanol extraction; after that, it was digested with 4 M NaOH/4 h, acidified with 2 ml of concentrated HCl, and the lipids were removed with 10 ml of hexane. Finally, the extraction of bounds phytochemicals was extracted four times using 10 ml of ethyl acetate each time. The recovered supernatant with the bound phytochemicals was evaporated in a rotary evaporator until dryness. Then, 2 ml of methanol was used to obtain the dry phytochemicals and stored at -20 °C until its use.
Antioxidant activity (AoxA)
The antioxidant activity (AoxA) was evaluated from phytochemicals present in free and bound extracts in each sample. The method of ABTS (2,2′-Azino-bis [3-ethylbenzothiazoline-6-sulfonic acid] diammonium salt) radical scavenging reported by Félix-Medina et al. (2020) was used to evaluate the antioxidant activity (AoxA). Aliquots of 20 µL of free or bound extracts were mixed with 980 µL of ABTS radical, and after 5 min rest, the absorbance of reaction was measured using a spectrophotometer (UV‐GENESYS model 10, Series AQ7-2H7G229001, Thermo Electron Scientific Instruments LLC, Madison, Wisconsin, EE.UU.) at 740 nm. The AoxA was expressed as μmol Trolox equivalents (TE)/100 g sample (dw).
Total phenolic compounds (TPC)
The TPC of free and bound extracts from the flours were quantified using the methodology of Gómez-Favela et al. (2021). Aliquots of 20 µL of free or bound extracts were oxidized with 180 ml of Folin-Ciocalteu reagent, forming a blue color after 90 min rest, and the absorbance of this reaction was measured in a spectrophotometer (UV-GENESYS model 10, Series AQ7-2H7G229001, Thermo Electron Scientific Instruments LLC, Madison, Wisconsin, EE.UU.) at 760 nm. The TPC was expressed as mg Gallic acid equivalents (GAE)/100 g sample (dw).
Experimental design
A rotatable central composite experimental design (response surface methodology) with two factors (process variables) (Extrusion temperature [X1 = ET, 50 °C-170 °C]/screw speed [X2 = SS, 50 rpm-240 rpm]) and five levels of variation (2 factorial [coded: -1, +1], 2 axials [coded: -1.682 (-α), +1.682 (+α)], and 1 central [coded: 0]) was used to obtain the process variables combination throwing 13 treatments (Table 1). These process variables were selected from literature according with Gómez-Favela et al. (2021), Leon-López et al. (2019), and Espinoza-Moreno et al. (2016), among others, who used extrusion temperature (50 °C-170 °C) and screw speed (50 rpm-240 rpm) as independent variables, reporting that these had a significant effect on several properties of studied materials. The desirability function was applied for the optimization of the extrusion process for sesame by-product flour. Water absorption index (WAI), water solubility index (WSI), in vitro protein digestibility (IVPD), total phenolic compounds (TPC), and antioxidant activity (AoxA) were selected as response variables. The extrusion process was optimized to find maximum values of WAI, WSI, IVPD, TPC, and AoxA.
Optimization
The stepwise regression procedure was applied, and non-significant terms (p > 0.1) were deleted from a second-order polynomial. A new polynomial was used to develop a predictive model for each response variable. The desirability method of the response surface methodology (RSM) was applied to determine the best combination of extrusion process variables. The five fitted models for the five response variables (WAI, WSI, IVPD, TPC, and AoxA) were evaluated on one point (X = X1, X2, X3, X4, X5) of the experimental zone, obtaining five values (Ŷ1[X1], Ŷ2[X2], Ŷ3[X3], Ŷ4[X4], Ŷ5[X5]). Each Ŷi (Xi) was transformed into a value di(X) (individual desirability), which falls in the range (0, 1) and measures the desirability degree of the response about the optimum value intended to be reached. An acceptable value for global desirability (D) should be between 0.6 and 0.8, being D = 1 the ideal optimum value. The D value was determined from the individual desirabilities of each one response variables through the following mathematical function: D = [(d1)(d2)(d3)(d4)(d5)]1/5. The statistical software Design Expert 7.0.0 was used for the RSM analyses.
Results
Table 2 shows the regression coefficients and the analysis of variance (Anova) for each response. In all cases, significant (p < 0.01) quadratic models without lack of fit (p > 0.05) were employed, and R2 adjust ≥ 0.87 as well as coefficient of variation (CV) ≤ 4.69% values were obtained. The experimental values of WAI, WSI, IVPD, TPC, and AoxA varied between 1.31-1.40 g of gel/g sample, 9.21%-10.32%, 73.45%-78.46%, 360.83 mg-683.27 mg of gallic acid equivalents (GAE)/100 g db, and 1811.28-2118.92 µmol of Trolox equivalents (TE)/100 g db, respectively (Table 1).
Table 2 Values of calculated regression coefficients, Anova results of the second-order polynomial models, and effects of the process factors on response variables a.
Coefficient | Water Absorption Index (YWAI) | Water Solubility Index (YWAI) | In Vitro Protein Digestibility (YIVPD) | Total Phenolic Compounds (YTPC) | Antioxidant Activity (YAoxA) |
Interception | |||||
β0 | 1.36 | 10.16 | 77.31 | 577.04 | 1881.04 |
Lineal | |||||
β1 | -0.023*** | 0.25*** | 0.094NS | 93.96*** | 49.10*** |
β2 | 7.9x10-3** | 0.081** | -0.72*** | -2.14NS | -60.40*** |
Interaction | |||||
β12 | 0.019*** | -0.12** | -1.77*** | 56.24*** | NS |
Quadratic | |||||
β11 | NS | -0.30*** | -0.97*** | NS | NS |
β22 | NS | NS | -0.53*** | -67.18*** | 70.05*** |
Pmodel | <0.0001 | <0.0001 | <0.0001 | <0.0001 | <0.0001 |
Lack of fit | 0.3282 | 0.1701 | 0.5176 | 0.8025 | 0.4554 |
R2 | 0.9178 | 0.9410 | 0.9842 | 0.9580 | 0.9081 |
R2adjust | 0.8904 | 0.9116 | 0.9728 | 0.9371 | 0.8775 |
R2pred | 0.7521 | 0.7787 | 0.9400 | 0.9121 | 0.8118 |
CV (%) | 0.58 | 1.00 | 0.31 | 4.69 | 1.59 |
*Significant (p = 0.05-0.1); **Significant (p = 0.01-0.05); ***Significant (p < 0.01); NS = No significant.
a β0 = Intercept; Lineal: β1 = Extrusion Temperature (ET), β2 = Screw Speed (SS); Interaction: β12 = ET-SS; Quadratic: β11 = (ET)2, β22 = (SS)2.
Source: Author's own elaboration.
Prediction models for response variables (WAI, WSI, IVPD, TPC, AoxA)
Water absorption index (WAI)
WAI is a parameter related with the modification level of the macromolecules present in thermally processed foods such as starch and fiber (Tiwari & Jha, 2017). The Anova showed that WAI significantly (p < 0.001) depended on lineal terms ET (p < 0.01) and SS (p < 0.05), as well as on their interaction (ET*SS, p < 0.01). The predicted quadratic model for WAI of extruded sesame flours (ESF) was the following:
Figures 1a and 2a show the contour and response surface graphics for WAI, respectively, where it can be observed that the higher WAI values were found at ET<80 °C and SS<190 rpm. In the WAI response, a saddle behavior was obtained (Figures 1a and 2a), and the stationary point was located at 92 °C and 228 rpm (WAI = 1.37 g of gel/g sample). In Figures 1a and 2a, it can be observed that the surface curves up in two directions from the stationary point: in direction of low ET and low SS and in direction of high ET and high SS; and it also curves down in two directions from the stationary point: in direction of high ET and low SS and in direction of low ET and high SS. The highest value of WAI (1.42 g of gel/ g sample) was observed at 50 °C and 50 rpm (Figures 1a and 2a).

Source: Author's own elaboration.
Figure 1 Contour graphics showing the effect of process variables (extrusion temperature and screw speed) on (a) water absorption index (WAI), (b) water solubility index (WSI), (c) in vitro protein digestibility (IVPD), (d) total phenolic compounds (TPC), and (e) antioxidant activity (AoxA) of extruded sesame by-product flours (ESBF).
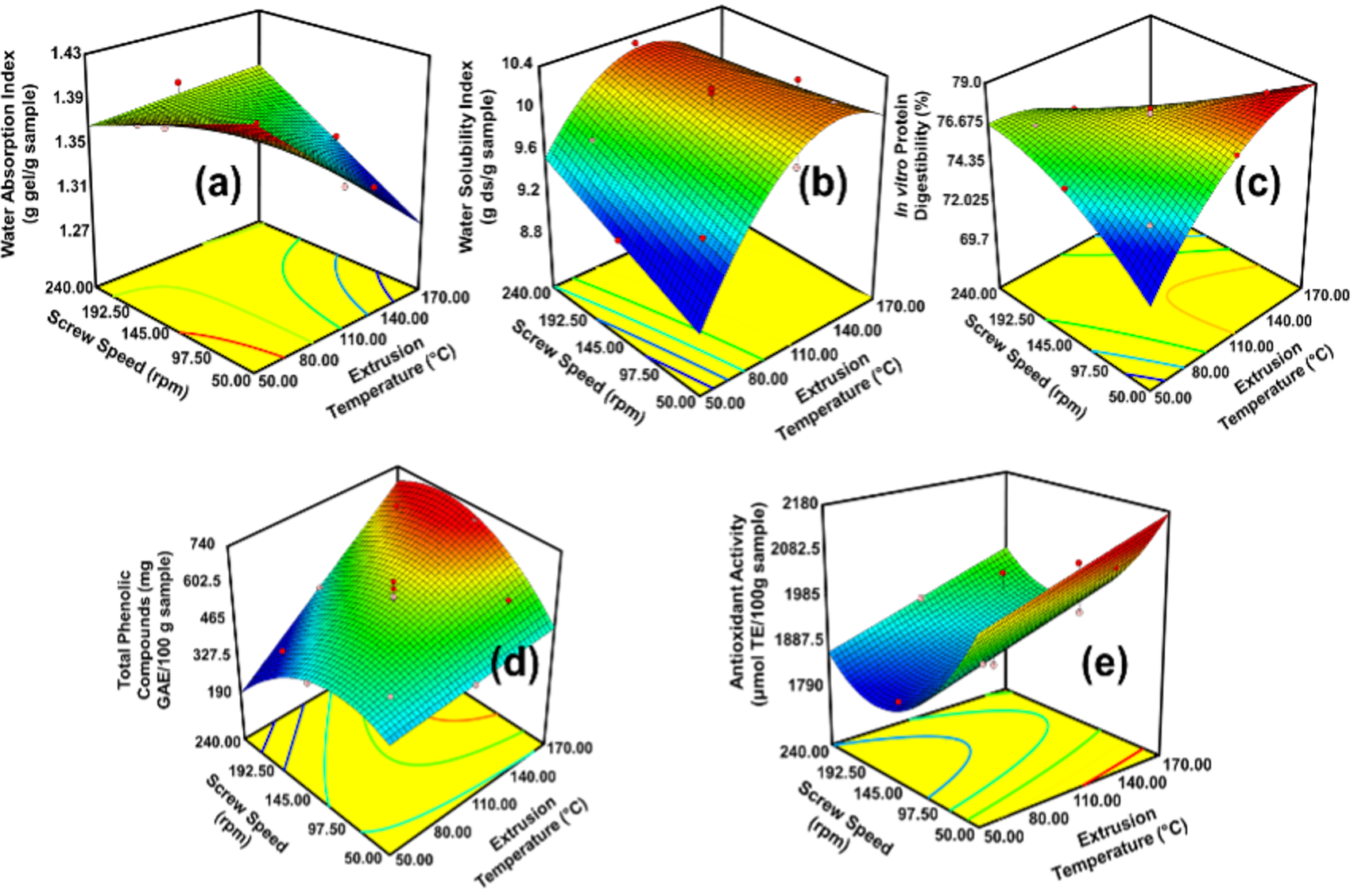
Source: Author's own elaboration.
Figure 2 Response surface graphics showing the effect of process variables (extrusion temperature and screw speed) on (a) water solubility index (WSI), (b) water solubility index (WSI), (c) in vitro protein digestibility (IVPD), (d) total phenolic compounds (TPC), and (e) antioxidant activity (AoxA) of extruded sesame by-product flours (ESBF).
Water solubility index (WSI)
The Anova showed a significant (p < 0.001) model for WSI. Also, WSI significatively (p < 0.01) depended on extrusion temperature (ET) both in its linear and quadratic terms (ET, p < 0.01, ET2, p < 0.01), as well as linear term of screw speed (SS, p < 0.05) and its interaction (ET*SS, p < 0.05). The prediction quadratic model for WSI of ESF was the following:
Figures 1b and 2b show the contour and response surface graphics, respectively, for WSI, where it can be observed that the higher WSI values were found at highest extrusion temperatures (ET > 110 °C) and all screw speed range (SS 50 rpm -240 rpm). In the WSI response, a saddle behavior was also obtained (Figures 1b and 2b), and the stationary point was located at 139 °C and 57 rpm (WAI = 10.2%). In Figures 1a and 2a, it can be observed that the surface curves up in SS direction from the stationary point, and it curves down in ET direction from the stationary point. The highest value of WSI (10.3%) was observed at 116 °C and 240 rpm (Figures 1b and 2b).
In vitro protein digestibility (IVPD)
Likewise, Anova showed a significant (p < 0.001) quadratic model on IVPD (Table 2). This response variable significantly depended on the quadratic term of extrusion temperature (ET2, p < 0.01), both in linear and quadratic terms of screw speed (SS and SS2, p < 0.01), as well as on its interaction (ET*SS, p < 0.01). The prediction quadratic model of IVPD from ESF was the following:
Figures 1c and 2c present the contour and response surface graphics (respectively) for IVPD, showing the effect of variables process (ET and SS) on this response variable. The highest IVPD values were found at high ET (>110 °C) and low SS (<145 rpm). Similar to WAI and WSI responses, in the IVPD response, a saddle behavior was also obtained (Figures 1c and 2c), and the stationary point was located outside the experimental region; it was located at 56 °C and 243 rpm (IVPD = 76.7%). In Figures 1c and 2c, it can be observed that the surface curves up in direction of high ET and low SS from the stationary point and curves down in two directions from the stationary point: in direction of low ET and low SS and in direction of high ET and high SS. The highest value of IVPD (79%) was observed at 166 °C and 50 rpm (Figures 1c and 2c).
Total phenolic compounds (TPC)
The Anova showed a significant (p < 0.001) quadratic model for TPC. Besides, this analysis revealed that TPC content from ESF significantly depended on the linear term of extrusion temperature (ET, p < 0.01) and the quadratic term of screw speed (SS2, p < 0.01), as well as on both factors’ interaction (ET*SS, p < 0.01). The prediction quadratic model for TPC from ESF was the following:
Figures 1d and 2d show the contour and response surface graphics, respectively, observing that the highest TPC values were found at high extrusion temperatures (ET > 130 °C) and high screw speed (SS > 100 rpm). Similar to the previous response variables, in the TPC response, a saddle behavior was also obtained (Figures 1d and 2d), and the stationary point is located well outside the experimental region at temperatures and screw speeds well below 50 °C and 50 rpm. In Figures 1d and 2d, it can be observed that the surface curves up in direction of high ET and high SS from the stationary point, and it curves down in direction of low ET and high SS. The highest value of TPC (732 mg of GAE/ 100 g, db) was observed at 170 °C and 184 rpm (Figures 1c and 2c).
Antioxidant activity (AoxA)
The Anova showed a significant (p < 0.001) quadratic model for AoxA. AoxA significantly depended on linear terms of extrusion temperature (ET, p < 0.001) and screw speed (SS, p < 0.001), as well as on the quadratic term of screw speed (SS2, p < 0.001). The prediction model for AoxA was the following:
Figures 1e and 2e show the contour and response surface graphics, respectively, where the effect of the process variables on this response can be seen. The highest AoxA values were found at middle-high extrusion temperatures (ET > 90 °C) and low screw speed (SS < 95 rpm). These figures also indicated that AoxA of ESF increased with ET until reaching maximum values at 170 °C. The increase of AoxA with ET in the full range of values of ET showed a linear behavior. In the figures mentioned above, a contradictory effect of SS on AoxA can also be observed. The values of this response first decreased with SS in the range of low to intermediate screw speeds, and then they increased with SS at screw speeds greater than intermediates. This behavior of AoxA with SS is because the quadratic effect of this process variable on this response was significant. The highest value of AoxA (2175 µmol of TE/100 g, db) was observed at 170 °C and 184 rpm (Figures 1e and 2e).
Optimization
The overall desirability value (D) obtained during the optimization of the extrusion process to produce optimized extruded sesame by-product flour (OESBF) was 0.812 (Figure 3). Desirability values in the range of 0.7 to 1.0 provide a good and acceptable product (Reyes-Moreno et al., 2012). Overall, desirability was used to determine the best combination of process variables (optimal conditions) for the production of optimized extruded sesame by-product flour (OESBF) with high WAI, WSI, IVPD, TPC, and AoxA values.

Source: Author's own elaboration.
Figure 3 Global desirability graphic with the optimal combination of extrusion variables as well as the prediction values for response variables evaluated.
Overall, desirability values correspond to the following process conditions: ET = 139 °C/SS = 80 rpm. With this combination of variables, the design predicted values of WAI = 1.3257 g of gel/g sample, WSI = 10.20%, IVPD = 78.28%, TPC = 543.74 mg of gallic acid equivalents (GAE)/100 g sample (db), and AoxA = 2037.38 µmol of Trolox equivalents (TE)/100 g sample (db). The experimental values obtained to validate the predicted values from optimization were the following: WAI = 1.3112 g of gel/g sample ± 0.05 g of gel/g sample, WSI = 9.85% ± 0.58%, IVPD = 79.9% ± 2.41%, TPC = 544.23 mg GAE/ 100 g sample (bs) ± 13.71 mg GAE/ 100 g sample (bs), and AoxA = 2047.45 µmol TE/100 g sample (db) ± 63.61 µmol TE/100 g sample (db).
Discussion
Water absorption index (WAI)
WAI is a measure of the volume occupied by starch after swelling in excess water (Ye et al., 2018). Some macromolecules such as amylose and amylopectin from starch may be modified during extrusion process (Logié et al., 2018). WAI is strongly correlated with the gelatinization degree and dextrinization of starch during extrusion process. At a higher degree of gelatinization, the starch granules hydrate, swell, and turn into a paste. A simultaneous melting of crystals and an unwinding of the double helix of the starch polymers contribute to the collapse of the granule structure, breaking the hydrogen bonds and, consequently, increasing the absorption of water (Sandrin et al., 2018).
Ye et al. (2018) carried out a review on the effect of extrusion on starch properties. The authors mention that, during the extrusion process, the starch gelatinizes, breaking its crystalline structure, where the inter and intramolecular hydrogen bonds are broken, exposing the hydroxyl groups to form hydrogen bonds with the water, which can diffuse more easily in the extruded starch than in the extruded starch in the native. Thus, WAI values are higher after extrusion but decrease when dextrinization or starch melting prevails over gelatinization, depending on the extrusion conditions used. When high moistures are used, the WAI values decrease because of the reduction in shear stress and starch degradation, due to the plasticizing effect of water. On the other hand, the WAI values increase when extrusion temperature increases due to the formation of an expandable matrix, caused by the separation of the amylose and amylopectin chains; and whereas the screw speed increases, the WAI values decrease.
Extruded flours with high WAI values are desirable for the production of bakery products and instant foods such as pasta, soups, and porridge, since they allow the addition of more water, improving the handling characteristics of the dough and avoiding dehydration of the products during storage (Ye et al., 2018). The WAI values obtained in the present work agree with the behavior mentioned above, finding that the optimal extrusion conditions caused an increase in the WAI content with respect to the unprocessed sesame by-product flour (1.3112 g gel/g sample vs. 1.253 g gel/g sample, respectively). This is due to the fact that the moisture content is at a medium value, reducing the protective plasticizing effect of water in the extrudate and allowing the extrusion temperature to increase its effect on the starch by separating the amylose and amylopectin chains, generating the expandable matrix.
Water solubility index (WSI)
WSI is considered a molecular degradation index caused in the starch granules and fiber during the extrusion process (Udachan & Sahoo, 2017). This behavior could be due to a high degradation of high molecular weight macromolecules such as starch and fiber, which generate compounds of lower molecular weight and, therefore, more soluble in water. According to Ali et al. (2017), an increment in pressure and shear force into extruder barrel is caused by the increment of extrusion temperature and screw speed, which intensify gelatinization and depolymerization of starch, resulting in an increment of WSI value. Froes-Gomes et al. (2015) reported that WSI values from any product (e.g., flours) depend on its chemical composition, and that the interaction between its chemical constituents and water highly depend on proteins and starch. According to these authors, the protein-water interaction is more relevant than amylose/amylopectin-water ratio for WSI, since they found that flours with higher protein levels gradually presented greater solubility.
The WSI measures the amount of soluble components released from starch after extrusion, being an indicator of the degree of conversion and molecular degradation of starch. Like the WAI, the WSI of the extrudates depends on the extrusion conditions. The use of high moistures results in low WSI values due to the reduced shear force and starch degradation during extrusion due to the plasticizing effect of water. On the other hand, WSI increases due to a marked shear force in the extrudate when high temperatures are used, presenting the same effect when high screw speeds are used. Therefore, extrudates with high WSI values are obtained when using low humidity and high screw speeds. The extruded flours obtained under these conditions tend to have low viscosity and stickiness, making them suitable for making food and drinks for children such as instant porridge, soups, and desserts (Ye et al., 2018). Like the WAI, the WSI value increased in the extrudate compared to the unprocessed sesame by-product (9.85% vs. 9.34%, respectively). This behavior indicates that the optimal extrusion temperature caused a degradation of the starch present in the study material.
In vitro protein digestibility (IVPD)
Generally, protein digestibility benefits from thermal denaturation. Proteins can lose their strongly folded structure, leading them to a greater accessibility of the peptide chain for hydrolytic enzymes, depending of the process severity and the type of protein (Joye, 2019). Arribas et al. (2017) mentioned that an IVPD increment could be due to both the protein denaturation and inactivation of some anti-nutritional factors such as protease inhibitors, which difficult the digestion. Also, the mechanical shear forces play an important role during extrusion cooking on the protein bodies alteration, enhancing its digestibility. Omosebi et al. (2018) observed an increment in protein digestibility after extrusion cooking of an infant formula consisting of maize protein, soybean protein concentrate, and cassava starch. The changes in proteins as well as the changes of in vitro protein digestibility during extrusion process are due to an interaction of the effects of the independent variables such as temperature, barrel moisture, and screw kneading block, along with the specific mechanical energy which depend of input variables (Zhang et al., 2017).
Sánchez-Magaña et al. (2022), Gámez-Valdez et al. (2021), and León-López et al. (2019) reported an increase in IVPD after extrusion of quinoa, amaranth, and defatted chia flour, respectively, under optimal conditions, and they mentioned that IVPD values may increase or decrease during food processing by extrusion. They attributed the IVPD increase (most reported phenomenon) to destruction of lectins, trypsin, and chymotrypsin inhibitors (antinutritional factors) as well as to protein denaturation, as an effect of the moisture, temperature, and shear force conditions used during processing; whereas the decrease of IVPD can be due to the protein binding by phenolic compounds, dietary fiber components, or Maillard reaction products formed during extrusion.
Dalbhagat et al. (2019) did a review on the effect of extrusion processing on physicochemical, functional, and nutritional characteristics of rice and rice-based products. They mentioned that proteins are affected by extrusion due to their heat labile characteristic, where alkali-soluble proteins are more affected at temperatures above 120 °C. Also, the moisture content helps protein aggregation and reduces their interaction, since high moisture content causes protein aggregates to form by crosslinking disulfide bonds, modifying the protein-starch morphology in the extruded product. Hardness, crispness, and brittleness of extruded products are correlated to protein content when using constant feed moisture. Likewise, molecular interactions such as protein-lipid-starch interaction can occur during extrusion, as well as the formation of a matrix encapsulating starch granules, delaying interaction with enzymes, causing a reduction in protein solubility. In the present study, the IVPD value was higher in extruded sesame by-product flour compared to unprocessed sesame by-product flour (79.9% vs. 68.5%, respectively). This increase can be attributed to the denaturation of the proteins present in the flour, losing its functional structure when in contact with high temperatures and being more exposed to the attack of digestive enzymes.
Total phenolic compounds (TPC)
Bioactive phytochemicals exist in free, soluble-conjugated, and bound forms. The bound phytochemicals are difficult to digest in the small intestine; however, the bacteria of the colon microbiota can digest them, thus providing beneficial effects such as the reduction of the incidence of colon cancer (Sánchez-Magaña et al., 2022). Phenolic compounds are present in vegetarian foods, with a structure characterized by the attachment of the hydroxyl group to a phenyl ring. These compounds have important functions in nutrition, oxidation stability, and sensorial quality of food products, apart from having the ability of scavenging reactive oxygen and nitrogen species, thus, deleting their oxidation cycle (Hashempour‐Baltork et al., 2018; Leonard et al., 2020). The TPC increment could be due to the release of phenolic bound to the cell wall membrane (Leonard et al., 2020). Hirth et al. (2015) reported that the residence time can decrease until 50% when screw speed is increased during the extrusion process; shorter processing time may have a greater retention of thermolabile compounds, like some phenolic compounds. Moreover, it has been reported that the extrusion process has two effects on phenolic compounds from cereals. First, high temperature causes the degradation and polymerization of thermolabile phenolic compounds; and second, the thermal treatment promotes the disintegration of cell wall matrix and break the covalent bonds in polyphenolic complex of high molecular weight, enhancing the accessibility of phenolic compounds (Ortiz-Cruz et al., 2020).
Gámez-Valdez et al. (2021) reported an increase in TPC in amaranth and blue corn after extruding them under optimal conditions. They mention that extrusion technology has the potential to develop functional foods due to the retention or increase of TPC content with antioxidant activity. Sánchez-Magaña et al. (2022) also reported an increase in TPC in defatted quinoa and chia flour extruded under optimal conditions. They mention that this increase may be due to (1) the breakdown and release of conjugated phytochemicals, (2) the prevention of enzymatic oxidation, and (3) the formation of colored compounds (products of the Maillard reaction) with antioxidant activity. For their part, León-López et al. (2019) reported that the increase in TPC in extruded defatted chia flour was due to the destruction of the cell wall, releasing phenolic compounds that were trapped (bound) in it, and to the formation of Maillard reaction products (MRP), which are quantified as phenolic compounds. These MRP react with the Folin-Ciocalteu reagent, oxidizing and absorbing at the same wavelength as the phenolic compounds. The results obtained for TPC agree with the behavior reported by the authors. The content of TPC in the extruded sesame by-product flour was 544.23 mg GAE/100 g dry sample, while for the unprocessed sesame by-product flour it was 502.35 mg GAE/100 g dry sample. This increase in TPC content could be due to the arguments mentioned in the literature above.
Antioxidant activity (AoxA)
Antioxidant activity shows the ability from a bioactive compound to maintain cell structure and function, effectively deleting the free radicals, inhibiting lipid peroxidation reactions, and preventing other oxidative damages (Zou et al., 2016). Generally, it is thought that heat treatments tend to decrease the content of antioxidant compounds in study materials. However, different investigations have reported increases in the antioxidant activity of extruded products (Gómez-Favela et al., 2021; León-López et al., 2019). According to Espinoza-Moreno et al. (2016), the increase in antioxidant activity could be due to the release of antioxidant phenolic compounds during the extrusion process, to the prevention of oxidation of phenolic compounds in the extruded product by enzymatic inactivation during processing, and to the presence of Maillard reaction products. The presence of amino acids and reducing sugars in raw materials, and the use of high temperatures during processing, allows the formation of high molecular weight complexes (e.g., melanoidins), which have been associated with antioxidant properties. Some authors have reported the presence of melanoidin precursor products in extrudates (Félix-Medina et al., 2021; Ying et al., 2017; Žilić et al., 2014).
Sánchez-Magaña et al. (2022), Gámez-Valdez et al. (2021), and León-López et al. (2019) reported an increase in AoxA after extrusion in quinoa, amaranth, and defatted chia, respectively. They attributed this increase in AoxA to the release of antioxidant phenolic compounds during the extrusion process. Bon-Padilla et al. (2022) reported an increase in AoxA in extruded chickpea flour. They attributed this increase to the release of TPC, to enzymatic inactivation preventing the oxidation of phenolic compounds in the extrudate, and to the presence of Maillard reaction products (MRP), which are generated during the extrusion process by reaction of free amino acids and reducing sugars. The antioxidant mechanism of melanoidins (MRP) is based on the ability to trap positively charged electrophilic species, to eliminate oxygen radicals, or to carry out metal chelation forming inactive complexes (León-López et al., 2019).
The extruded sesame by-product flour presented a higher value than the unprocessed sesame by-product flour (2047.45 µmol TE/100 g dry sample vs. 1924.87 µmol TE/100 g dry sample, respectively). This increase in AoxA is correlated with the increase in TPC content, as mentioned above.
Optimization
Generally, in the food industry, researchers are interested in optimizing several responses concurrently, which is complicated compared to optimizing processes with only one response. For this purpose, a desirability function is used, which is a multi-criteria methodology. This methodology makes a desirability function (DF) for each individual response. The desirability function approach is one of the most widely used methods in the industry for the optimization of multiple-response processes. It is based on the idea that the quality of a product or process which has multiple quality characteristics, with one of them outside of some desired limits, is completely unacceptable (Yolmeh & Jafari, 2017). The DF differentiates and creates a function for each response and then determines the global function for desired responses by selecting the optimum values for variables and considering the interaction between them (Iftikhar et al., 2020). Reporting the desirability of responses includes the identification of DF for each response (WAI, WSI, IVPD, TPC, and AoxA). Desirability is scaled in the range of 0-1, with 0 representing undesirable and 1 representing very desirable. This scale is used to find a global function (the individual desirabilities are then combined using the geometric mean, which gives the overall desirability) that should be amplified by an effective selection and optimization of variables (Iftikhar et al., 2020; Yolmeh & Jafari, 2017). In the materials and methods section, the desirability function procedure was described as an optimization technique. In this research work, the DF for different responses were selected as the maximum WAI, WSI, IVPD, TPC, and AoxA in the range of the observed values.
The desirability ranges from 0 to 1, showing minimum to maximum desired results, respectively. In Figure 3, the graph of global desirability was plotted as a function of the extrusion process variables (ET, SS). The blue region is the area of 0 desirability, showing that in the combination of variables that lie in blue area cannot produce the desired results. The region that shades from green to orange includes the combination suitable for acquiring desired results, and as the extrusion temperature increases, the desirability also increases. A global desirability of 0.812 can be achieved by combining the factors (139 °C/80 rpm) shown in Figure 3.
Measurement of response under the predicted optimum conditions and comparison of the observed responses with the responses predicted through the model is another important point. The difference between observed and predicted responses must be as low as possible; this means that the predicted optimal conditions are valid (Yolmeh & Jafari, 2017). In this work, optimized extruded sesame by-product flour (OESBF) was produced applying the best combination of extrusion process variables; the experiment using optimal conditions was replicated three times. The experimental values of WAI, WSI, IVPD, TPC, and AoxA of OESBF were similar to the predicted values shown in Figure 3, indicating that the optimal conditions of extrusion were appropriated and reproducible. The application of food processes such as extrusion, under optimal conditions, when it has been optimized to obtain the maximum values of some properties such as WAI, WSI, IVPD, TPC, and AoxA, allows to obtain mainly extruder flours with high values of these properties (Bon-Padilla et al., 2022). Extruded flours are suitable for developing new functional foods with healthy benefits.
In this work, the predicted (WAI = 1.3257 g of gel/g sample, WSI = 10.20%, IVPD = 78.28%, TPC = 543.74 mg GAE/100 g dry sample, AoxA = 2037.38 µmol TE/100 g dry sample) and the experimental (WAI = 1.3112 g of gel/g sample, WSI = 9.85%, IVPD = 79.9%, TPC = 544.23 mg GAE/100 g dry sample, AoxA = 2047.45 µmol TE/100 g dry sample) values were similar between them, indicating that the optimal conditions of the extrusion process were appropriate and reproducible. There was no significant difference between the values predicted by the model and the real values obtained in the validation. Therefore, the models used in each response are valid to find the optimal processing conditions to obtain OESBF with high values of WSI, IVPD, TPC, and AoxA. These values obtained in OESBF were higher than unprocessed sesame by-product flour (USBF), where values of these responses were WAI = 1.25 g of gel/g sample, WSI = 9.34%, IVPD = 68.5%, TPC = 502.35 mg GAE/100 g dry sample, and AoxA = 1924.87 µmol TE/100 g dry sample. The OESBF, due to values obtained, could be used to elaborate functional foods such as porridges, beverages, cookies, soups, and others, which could provide health benefits.
Conclusions
The best combination of extrusion process variables to produce extruded sesame by-product flour with high values of water absorption index, water solubility index, in vitro protein digestibility, total phenolic compounds, and antioxidant activity was ET = 139 °C/80 rpm. The experimental values from validation of response variables were very close to the predicted values. The optimized extrusion process increases the values of WAI, WSI, IVPD, TPC, and AoxA of sesame by-product flour. The extrusion process is an efficient technology to improve the functional, nutritional, and nutraceutical characteristics of foods. This extruded flour under optimal conditions could be used as an ingredient to elaborate beverages and/or functional foods. It is necessary to carry out more studies on the characterization of the sesame by-product flour and the effect of the optimal conditions of the extrusion process on the other chemical, physicochemical, techno-functional, nutritional, and nutraceutical properties of this flour. In addition, some functional foods could be developed, and their effect in vivo could also be evaluated.
Conflict of interest
The authors declare no conflict of interest.