Introduction
In ancient Mesoamerican cultures (as in many other parts of the world), salt was a vital, essential mineral for human consumption, as well as for food preservation and fabrics dying (Williams, 2010). In Mexican pre-Hispanic societies, salt was produced mainly in saltworks located in coastal zones, but inland production was also important, as has been documented for Lake Cuitzeo and Lake Texcoco (Williams, 2010).
Saline and brackish lakes (athalassohaline) are characterized for their high content of sodium carbonate (or complexes of this salt), achieved by concentration through evaporative processes (Grant & Sorokin, 2011). Lakes of this origin can be found in USA, Mexico, Venezuela, Hungary, India, China, Ethiopia, and Chad, among other countries around the world (Grant & Sorokin, 2011).
In Mexico, the largest saline (athalassohaline) lake was Lake Texcoco, originally located in the Valley of Mexico, at 2240 m above sea level (Luna-Guido et al., 2000). pH in this waterbody was high (>10), and different pre-Hispanic food resources thrive in these waters, being the most important the cyanobacteria Spirulina, which was harvested and consumed for the Aztecs. This lake was desiccated some centuries ago but the water table is very shallow and, at present, it is possible to observe natural water outcrops in some areas of its original basin, easily recognizable by its reddishbrown coloration.
In former Lake Texcoco basin, salinity of natural water outcrops can reach up to 80 g/L to 90 g/L in the dry season, so salt crusts covering extended areas can be observed (Alcocer & Williams, 1996). These crusts are called tequesquite (from nahuatl tetl: stone, and quixquitl: sprout, sprouting stone), a mineral complex that has been used in Mexico since pre-Columbian times to cook and flavor foods like corn, cactus pads (nopal), and beans. Nowadays it is still commercialized in some local and regional markets as little stones (Kennedy, 1998). Importance of tequesquite in other parts of ancient Mexico is mentioned by Williams (2010), in Lake Cuitzeo margins, where this mineral was also collected and commerce for human consume.
Tequesquite is an amorphous and solid material, crystallized or in powder form, that depicts varying colors ranging from white to dark-grey, depending on the degree of purity and clay content. It is classified in five categories with the following local denominations: confitillo, cascarilla, espumillo, polvillo, and dark tequesquite, depending on the collecting season and place of origin (Flores, 1918).
The only formal reports about tequesquite’s chemical composition are from the first half of the twentieth century (Flores, 1918; Galván-Moreno, 1945; Navarro-Almanza, 1925), contained in the Mexican Geologic Institute Annals, and in undergraduate theses. Since then, no more detailed analytical studies have been performed and no publications are available about the detailed composition of this mineral complex, in spite of the current accessibility to more sophisticated information-retrieval systems. In that studies, it was determined that tequesquite is a mix containing 81% salts, being predominant sodium bicarbonate (45%) and sodium chloride (34%). Based on the aforementioned, the aim of the present study was to identify chemical and elemental composition of tequesquite and to determine the influence that clays and sandy insoluble material could have on its composition (as part of impurities accompanying it). In addition, we aimed at discarding possible presence of toxic elements that could harm human health through consumption of foods prepared with tequesquite.
Material and methods
Samples acquisition and preparation of control solution
The mineral complex, tequesquite, was acquired from local markets; all of them have the same origin: outcrops from water table of former Lake Texcoco collected in different hamlets in that area. Samples were identified as: A and B (with differences in the visual aspect, both obtained from two different suppliers from La Merced market in Mexico City), C (from Cocotitlan market in Estado de Mexico), and D (purchased in Chalco market, Estado de Mexico). These samples were stored in dry conditions and protected from light.
Tequesquite from Cocotitlan (C) was dissolved in deionized water until solution became salt saturated (salinity greater than 70 psu, measured with a salinity-conductivity meter YSI-30). This solution, abundant in clay-sandy sediments, was left to settle for about 12 h, afterward it was decanted and, finally, vacuum-filtered to obtain a clear, red-brownish colored, free of particles solution. This was denominated as the “tequesquite solution free of insoluble material” (TSFIM). Sample C was used to prepare TSFIM as it exhibits a medium quality (as will be confirmed later) that corresponds to the usual type of tequesquite found in most of local markets throughout the year.
In TSFIM pH was measured using a Thermo potentiometer (Orion 3 STAR). NO3- content was quantified with cadmium reduction method (method 8039), and PO4 3- was determined with molybdovanadate method (method 8114), both on a HACH DR/2000 spectrophotometer. HCO3- and CO3 2- concentrations were determined with a titration method using phenolphthalein and methyl orange as indicators. Na and K content were quantified by means of atomic spectroscopy (Variant Spectraa 220). Furthermore, a sample of TSFIM was evaporated to dryness at 95 °C. Crystallized salt obtained through this procedure was identified as “E”, and analyzed as described below.
Elemental composition and chemical compounds identification with X-ray diffraction
All tequesquite samples (including sample E) were pulverized on a mortar with a pestle and uniformly mixed. Afterward they were dehydrated at 90 °C for 24 h and stored in glass-lid vials for analytical determinations.
Elemental composition was determined by means of scanning electron microscopy (SEM) equipped with a FEI™ analytical system of X-ray dispersive energy (EDXRA) (model Quanta™ 3D FEG) in the Centro de Nanociencias y Micro y Nanotecnologías of the Instituto Politécnico Nacional. Micrographs were obtained for each sample. With this technique all elements with an atomic number greater than 5 can be detected.
Additionally, chemical compounds in each sample were identified by X-ray diffraction (XRD). Patterns were obtained employing a PANalytical XRD equipment (model X’PERT Pro MRD).
Results
Table 1 shows chemical composition determined in sample E (crystalized form of the TSFIM). Sodium ion was the most abundant followed by potassium, carbonates, and bicarbonates, at similar concentration, and trace amounts of phosphates and nitrates were also determined. Remainder tequesquite content is represented mainly by insoluble particles (clays and sand) and moisture, but some other elements like sulfur and chlorine were also present (as seen afterward).
Table 1 Chemical composition of tequesquite solution free of insoluble material (TSFIM), obtained from sample E. Average values ± standard error limits.

Source: Author's own elaboration.
Alkaline pH (9.83) is due to presence of hydroxide ions, carbonates, and bicarbonates, conditions that prevailed in the brackish waters of former Texcoco Lake.
Figure 1 shows images obtained with SEM (1000x). Samples a, b, c, and D have a similar morphology, being remarkable the higher amount of lumpy agglomerates and some elongated rods, whereas morphology of sample E is notably different: lump agglomerates are found in a lower amount and a surface with less reliefs is observed. In higher magnifying micrographs, sharppointed crystals morphology can be seen (figure 1f).
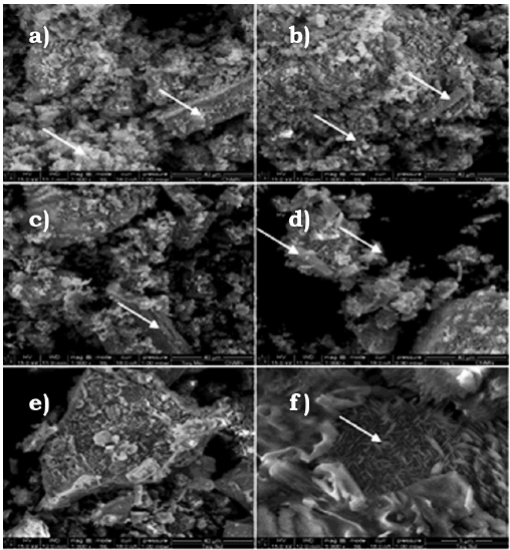
Source: Author’s own elaboration.
Figure 1 Scanning electron microscope micrographs of different samples of tequesquite (a: sample D, b: sample C, c: sample B, d: sample A; e and f: sample E; a, b, c, d, e: 1000x; f: 5000x 15.0 kV). Arrows indicate the types of morphology observed.
Elemental composition of crystal morphology types previously described is included in table 2. Two elemental composition analyses on selected spots were conducted on lumpy agglomerate-type morphology because this was the most abundant morphology contributing to tequesquite’s ultrastructure. In all cases, carbon, oxygen, sodium, magnesium, aluminum, silicon, sulfur, chlorine, and potassium were detected. On samples D, C, and B iron was detected in all morphology types. In lumpy agglomerate-type morphology of D and A samples a reduced phosphorus amount was detected. According to average values of elemental selected spots analysis, oxygen and carbon were predominant elements, except in D sample, where oxygen and sodium were the most abundant. The third more abundant element in samples A, B, and C was sodium. Elemental composition was a function of morphology type. Excluding A sample, all samples had a high amount of carbon and less silicon in rod-type morphology than in agglomerate morphology types.
Table 2 Elemental composition percentage (weight) of tequesquite raw samples (A, B, C and D). Percentages are shown for each kind of morphology and the average is for each element; limits are for the standard error values.

n/d = Not detected elements. Source: Author's own elaboration.
Elemental analysis on selected spots of sample E is shown in table 3 indicating presence of carbon, oxygen, sodium, silicon, sulfur, chlorine, and potassium. As in raw tequesquite samples, elemental composition was also a function of morphology type. In agglomerate and crystal-type morphologies (the latter was a remarkable morphology for this sample), the most abundant element was oxygen, and carbon predominated in the rod morphology type. In this sample no magnesium, aluminum, phosphorus neither iron were detected, and silicon was only detected in rodtype morphology in a smaller proportion than in the rest of the samples. In addition, it can be observed that amounts of sodium and sulfur in this sample were considerably higher as compared to raw samples. This can be explained by the fact that these two elements are conjugated with other elements, forming water soluble compounds (chemical compounds that remain unaffected by the separation processes) that became concentrated in TSFIM preparation.
Table 3 Elemental composition percentage (weight) of sample E. Percentages are shown for each kind of morphology and the average (± standard error limits) for each element.
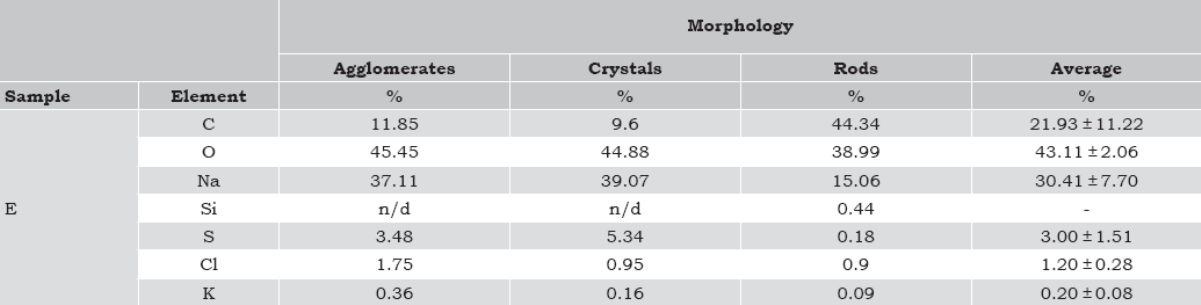
n/d = Not detected Source: Author's own elaboration.
The high amount of sulfur here detected could be related with TSFIM color of solution, once non-soluble particles were discarded (red-brownish).
Sample E corresponds to a concentrated tequesquite solution that was subjected to separation and crystallization processes by which it became clay- and sand-free (insoluble material), besides, due to these processes, no aluminum, magnesium, or iron were detected in this sample and only a very reduced amount of silicon. All these elements belong to the insoluble material (clay and sand).
Diffraction patterns of tequesquite samples can be seen in figure 2. All raw samples have a very similar diffraction pattern, indicating that different sources of tequesquite samples have the same chemical “footprint”, namely, no variation exists among their chemical compound diversity but in their relative abundance. This is closely related with clay and sand content, because a higher amount of these “impurities” reduce proportionally other chemical compounds.

Source: Author's own elaboration.
Figure 2 X-ray diffraction patterns of raw tequesquite samples (A, B, C and D) and sample E (obtained through solubilization in water, decantation, and crystallization of sample C, for removal of impurities and insoluble materials).
As can be seen in figure 2, sample E’s diffraction pattern differs from other samples due to removal of insoluble clay-sand, which allows getting a higher resolution of other chemical compounds.
From diffraction patterns it was possible to identify four chemical compounds in samples A, B, C, and D: NaCl, trona (sodium sesquicarbonate), SiO2, and Na2CO3. Contrariwise, in sample E, SiO2 was not detected but two other chemical compounds different from trona and NaCl were distinguished: KCl and K2SO4. All identified compounds agree with elemental analysis previously described, with exception of hydrogen owing to reason explained under methodology.
Different micrographs of tequesquite samples are shown in figure 3 that correspond to rod-type crystal morphology. It can be seen that in sample E this structure has a higher resolution than in the rest of samples.
Discussion
Traditional use of tequesquite in Mexico is mainly in food cooking, after its solubilization in water to eliminate clays, sand and other impurities that could negatively affect the quality of the processed food. According to information in tables 1 and 3, tequesquite's solution contributes with sodium and potassium ions, responsible of salt taste otherwise added as NaCl during cooking. Concentration of bicarbonate and carbonate and high pH value (related with NaOH and KOH content), act as softener of hard seeds and cactus and help to preserve a vivid green color in vegetables.
Presence of NaCl and Na2CO3 in tequesquite samples agrees with incomplete composition reported previously (Garritz-Ruiz & Chamizo, 1989; Stoner, Millhauser, Rodríguez-Alegría, Overholtzer & Glascock, 2013) and explains (at least partially) the reason for its culinary use in Mexico. As is shown in tables 1 and 2, no toxic elements were detected that could question culinary use of this saline complex.
As shown in table 2 and figure 2, composition of tequesquite was similar in all analyzed samples. Nevertheless, quality of tequesquite is a function of the amount of clays and insoluble material content. Samples containing a higher amount of these compounds are of lower quality and price. Tequesquite purity can be easily detected by coloration of raw product: white color means a higher purity degree (in soluble salt content terms) and less amount of organic matter, clays, and other insoluble materials. Quality of studied samples here is as follows (based on sum of amounts of aluminum, silicon, iron, and magnesium): B (12.27%) < D (11.53%) < C (9.91%) < A (4.33%). This order is consistent with qualitative, visual aspect order, as A sample was the whitest, samples B and D had a brown coloration due to a high content of clays, and finally C sample had white stone fragments but most of them depicted also a brown coloration (image not shown).
SiO2 is the most abundant chemical compound that is found in sand (Armstrong-Altrin et al., 2012; Brems et al., 2012; Hasdemir, Tugrul & Yilmaz, 2012) and in clays (Dondi, 1999; El-Mahllawy, Sharara, Hassaan & Abdel-Haleem, 2013; Galos, 2011), hence it was no detected in sample E (table 3). Solubilization of tequesquite eliminates all insoluble materials (clays and sand), and produces the purest mineral sample after crystallization of this solution. (table 3, figure 3e). It is important to point out that clay-sandy content variations of each tequesquite commercial sample depend on place and weather conditions during collection of this product (Flores, 1918).
Trona is a double-hydrated carbonate and bicarbonate salt that can be found in some places around the world (Gärtner & Witkamp, 2007) with chemical formula Na2CO3 ∙ NaHCO3 ∙ 2H2O from which Na2CO3 can be obtained. Trona is formed by evaporation and concentration of high Na+ and HCO3- containing waters (Earman, Phillips & McPherson, 2005), conditions that are supposed to have prevailed in the former Texcoco Lake subsoil (Gärtner & Witkamp, 2007).
Trona SEM observations were carried out by Cho, Keener & Khang (2008) and they observed similar structures to rod-type morphology detected in the present study. Occurrence of trona (an innocuous mineral) in tequesquite is for the first time reported. However, it is necessary to determine quantity of this mineral in tequesquite because its abundance depended on sample quality, as shown in figure 3.
Other chemical compounds like Na2SO4, P2O5, and CaO could also be present in samples in a low amount, as reported by Flores (1918), but were undetectable in the present study. Probably, because their presence is a function of place and time of collection as mentioned above.
The former Lake Texcoco, located at the bottom of the endorheic basin of the Valley of Mexico, supported important economic activities, such as collecting Spirulina (classified at present as Arthrospira maxima) and insects for human consumption (Parsons, 2010), but this alkaline and saline waters were also used for salt production, as has been documented by Apenes (1944), and addressed by Williams (1999).
Parsons (1990) investigated salt production in San Cristobal Nexquipayac, a rural community located in the northeastern shoreline of the former Lake Texcoco, concluding that this is an ancestral activity whose procedures could be essentially the same applied since A. D. 1200. This activity comprises the following steps: 1) collection of saline soils, encrusted with salts produced by solar evaporation of water-table outcrops; 2) soils are mixed in proper proportions to produce some of the four final products (white salt, black salt, yellow salt, or saltpeter); 3) mixed soils are compacted in clay-lined pits and freshwater is percolated to concentrate salts in a brine solution; 4) brine solution is boiled in large metal pans to crystalize the salts; 5) salt crystals are dried; and 6) salts are ready to be commercialized.
Salt obtained from Lake Texcoco’s shorelines was destined to ordinary people, whereas urban Aztec elite consumed the fine, white salt obtained as tributes from Western provinces (Parsons, 1990). Tequesquite could be categorized as a coarse type of salt not used as table salt but for some foods cooking. Use of this mineral resource for meals preparation is ancestral, and has persisted up to present, although its detailed chemical composition remained unknown.
According to chemical composition determined herein, and due to alkaline conditions that solubilization of tequesquite produces in water, its use in maize “nixtamalization” process and in the procedure to “soften” other hard grains (fresh or dried) can be understood. These softened grains have been consumed traditionally as foods since pre-Hispanic times by both ancient and modern Mesoamerican cultures. Possible risks to human health by presence of toxic metals can be discarded, as our results confirm.
Finally, salinity and alkalinity of water solution of tequesquite enable its use in algal biotechnology, as basis for formulation of a medium for Arthrospira maxima cultivation. This cyanobacterium thrives in former Lake Texcoco and is cultured in synthetic high cost media to produce biomass used in industry and for human nutrition at present.
Conclusions
It was found that mineral complex known in Mexico as tequesquite has a high amount of aluminum, silicon, magnesium, and iron that are conjugated with other elements forming clays and insoluble sand, but which became undetectable after applied separation processes (solubilization-decantation-filtration-crystallization procedure). NaCl, trona, SiO2, and Na2CO3 were identified in clay-sandy tequesquite samples and NaCl, trona, KCl, and K2SO4 in insoluble material-free sample. SEM-XRADE allowed us to discard presence of toxic elements that could be of concern regarding its culinary use in Mexico. Tequesquite acquired from different sources has the same chemical compounds, differing only in clay and sand content that can be considered as impurities.