INTRODUCTION
Fluoride is a chemically active non-metallic element. It bonds directly with most elements and indirectly with nitrogen, chlorine, and oxygen, and is released from the majority of compounds that contain fluorides. Calcium fluoride (CaF2) can be found specifically related to some important areas at the Central and North of Mexico (Guanajuato, San Luis Potosí, Aguascalientes, Jalisco, Zacatecas, Chihuahua, Sonora) and is among the most stable chemical compounds. Fluoride is also found in seawater, rivers, and mineral springs, and in the stems of certain herbs, bones, and in animal teeth (Craig, Lutz, Berry & Yang, 2015; Fordyce, 2011; Tripathy, Bersillon & Gopal, 2006). The concentration of fluoride ions in water varies according to their origin. Surface waters tend to contain lower concentrations of this ion in comparison with the groundwater because the latter is in contact with deposits of the mineral fluorite and other minerals that contain fluoride (Thompson, 2012; Maheshwari, 2006).
Water is an essential natural resource for sustaining life on the earth. However, the chemical composition of the surface or subsurface is one of the prime factors upon which the quality of water for domestic, industrial, or agricultural purpose depends. According to World Health Organization (WHO) guidelines and NOM-127-SSA1-1994, the fluoride concentration in drinking water should not exceed 1.5 mg/L (Maliyekkal, Shukla, Philip & Nambi, 2008; Vences-Álvarez, Velázquez-Jiménez, Chazaro-Ruiz, Díaz-Flores & Rangel-Méndez, 2015). Several techniques have been used for the removal of fluoride from drinking water by ad-sorption, ion exchange, electrodialysis, electrocoagulation and precipitation processes. In those methods for removal of fluoride ions, the adsorption onto a solid surface (activated alumina, activated charcoal, zeolites, etc.) is a simple, versatile, and appropriate process for treating a drinking water system, especially for small communities. Adsorption can remove ions over a wide pH range and to residual concentrations lower than precipitation, and it is an economical technique (Guzmán, Nava, Coreño, Rodríguez & Gutiérrez, 2016; Rafique, Awan, Wasti, Qazi & Arshad, 2013; Sujana & Anand, 2010). Today, there is a need to focus greater attention on the potential future damage to human health and the environmental impact caused by the fluoride ion, which has been found in drinking water (Mohapatra, Anand, Mishra, Giles & Singh, 2009; Valdez-Jiménez, Soria-Fregozo, Miranda-Beltrán, Gutiérrez-Coronado & Pérez-Vega, 2011).
The objective of this work is to compare the removal of fluoride ions from drinking water using activated γ-Al2O3 and activated charcoal at varying pH (5 and 7) levels, which are adjusted with hydrochloric acid.
Experimental procedure
We compared the effectiveness of two different materials in the removal of fluoride from potable well water, the first fibrillar gamma alumina nanopowder (γ-Al2O3), and the second a commercial activated charcoal powder (CA). The first material, γ-Al2O3, was prepared by homogeneous precipitation. A basic aluminum sulfate (BAS) was synthesized from a mixture of aluminum sulfate and ammonium bisulfite solutions. The BAS was then neutralized in a solid/liquid reaction with ammonia solution to transform it to aluminum hydroxides. Then, hydroxides were oven dried at 110 °C to obtain pseudoboehmite which was in turn used as a γ-Al2O3 precursor since it transforms to this phase at 450 °C. (Sugita, Contreras, Juárez, Aguilera & Serrato, 2001; Zamorategui, Soto & Sugita, 2012a). Purity of the second material, commercial charcoal (Karal), is described in Table 1. Specific surface area of both adsorbent materials was determined by single-point Brunauer, Emmett and Teller (BET) method (ASAP, 2010 Micromeritrics Instrument Corp., USA). Morphology and particle size was examined by Field Emission Scanning Electron Microscopy (FE-SEM; JEOL, JSM 7401F). Zeta potential (pZ), particle size of the γ-Al2O3, and dispersed activated carbon in water were measured by electroacoustic technique with a particle size analyzer (AcoustoSizer II, ESA; Colloidal Dynamics, USA) using a 10 mM solution of potassium chloride (KCl). Remaining fluoride ion in the final treated water was measured using a fluoride ion selective electrode.
Samples were prepared with concentrations of 5 ppm (mg/L), 10 ppm (mg/L), 25 ppm (mg/L), 50 ppm (mg/L), 100 ppm (mg/L), 125 ppm (mg/L), 150 ppm (mg/L) and 200 ppm (mg/L) of fluoride ion in deionized water. We used 0.3 grams of adsorbent material, and the pH (5 and 7) was adjusted with hydrochloric acid. Samples were left for five days at room temperature (28 °C). After five days, solution was filtered and final concentrations of fluoride ions were determined. It was considered that equilibrium is reached when the fluoride concentration obtained was the same or that there was a difference of 5% over the earlier recorded period.
RESULTS AND DISCUSSION
Particle size and morphology
Figure 1a shows the FE-SEM image of the activated γ-Al2O3 powder prepared by homogeneous precipitation. The powder consists of nanofibers about 100 nm in length that tend to form amorphous agglomerates due to their high surface energy, therefore generating high porosity. In comparison with the morphology of the activated charcoal powder (Figure 1b), we can see that the powder exhibits a higher degree of agglomeration and consequently has a lot of small pores.
Adsorption/desorption of N2, BET analysis
Figure 2 shows the adsorption-desorption isotherms of the γ-Al2O3 and CA. The γ-Al2O3 isotherm shows a type IV according to the International Union of Pure and Applied Chemistry (IUPAC) definition, which is characteristic of a mesoporous material (Kim, Lee, Jun, Park & Potdar, 2007; Park & Jeong, 2008). Irregular shape isotherms with the hysteresis loop type E indicate that the pores in the material have an inkwell-type shape. The γ-Al2O3 synthesized by homogeneous precipitation has a smaller specific surface area (332 m2 • g-1) than that determined for the CA (601 m2 • g-1) (Lavado-Meza, Sun-Kou & Recuay-Arana, 2012). The smaller area presents an isotherm type I, according to the IUPAC classification, which is characteristic of processes where micropore filling occurs. The major consumption of N2 in the adsorption-desorption isotherm of the CA occurred at a low relative pressure (< 0.2) and reached a plateau at high relative pressure. In agreement with the porosity observed by FE-SEM, and the above-mentioned pore distribution, the CA and γ-Al2O3 are micro and mesoporous materials respectively (Figure 2b).
Zeta potential and particle size
Figure 3a shows the effect of pH variation on zeta potential. Profile of zeta potential vs. pH corresponds to changes in particle surface charge and takes the characteristic shape and isoelectric point typical for γ-Al2O3. Its isoelectric point (IEP) was found to be at pH 8.5, which is consistent with reported data (Bowen, Carry, Luxembourg & Hofmann, 2005). As can be seen in Figure 3a, potential in the γ-Al2O3 suspension is high, either below or above the IEP, i.e., for negatively and positively charged particles and these changes in potential are related to the flocculation phenomena and the neutralized surface charge (Zamorategui, Surgita, Zárraga, Tanaka & Uematsu, 2012b). In contrast with the CA, surface charge of the γ-Al2O3 is always positive at low zeta potential. This result can have an effect on the removal of the fluoride ion (F-). Furthermore, the particle size of γ-Al2O3 is smaller than that of the CA (Figure 3b) which is related to their zeta potential.
Langmuir isotherm
Removal of an ion is mainly attributed to the inter -action of surface groups and the ion through various mechanisms, such as: donor-acceptor reactions of electrons and/or formation of metal complexes such as COOH-M (Elmouwahidi, Zapata-Benabithe, Carrasco-Marín & Moreno-Castilla, 2012; Mor, Ravindra & Bishnoi, 2007). Activated charcoal and γ-Al2O3 are well-known as adsorbents of substances interacting by van der Wall's forces.
Adsorption isotherms are important to describe how the adsorbate interacts with the adsorbent, and it is important to establish the most appropriate correlation for the equilibrium curves. Thus, several isotherms (namely, Langmuir, Freundlich, Temkin, and Dubinin-Radushkevich) have been studied. Adsorption isotherms for γ-Al2O3 and activated charcoal are shown in Figure 4a and these are in agreement with the Langmuir equation which is represented as:
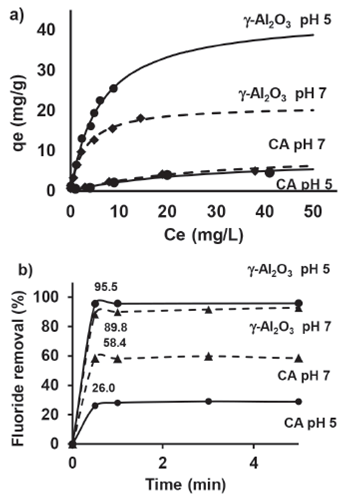
Source: Author's own elaboration.
Figure 4 Graphs a) Langmuir isotherms and b) Percent removal curves of the γ-Al2O3 and CA.
Where qe is the amount of fluoride ion adsorbed per gram of adsorbent, Qmax is the maximum amount of fluoride ion per unit weight of absorbent to form a complete monolayer on the surface (mg • g-1), k is the Langmuir constant, and Ce is the concentration of fluoride ion remaining in the solution (Ghorai & Pant, 2005). Table 1 summarizes the parameters of the Langmuir equation at pH 5 and pH 7.
In order to determine the optimum pH for the maximum removal of fluoride ions, equilibrium adsorption of fluoride ions was carried out at pH 5 and pH 7. It is clear that the adsorption by mesoporous γ-Al2O3 and microporous CA is dependent on the initial pH of the solution, and the maximum uptake is obtained at pH 5, approximately 35 mg of F-/g and 8 mg of F-/g, respectively. Results show that γ-Al2O3 possesses a much higher adsorption capacity than the CA at both pH 5 and pH 7.
Removal curves of the γ-Al2O3 and CA at pH 5 and pH 7 are shown in Figure 4b. In this study, solutions of 50 ppm of fluoride ion were used. As can be seen, maximum rate of fluoride removal was during the first minute and the γ-Al2O3 is the better adsorbent (95.5%) in comparison with the CA (26%) at pH 5 and at pH 7 (89.8% and 59.4%).
Results indicate that the adsorption of fluoride ions occurs on the outermost surface of the adsorbent material, and this is not affected by the difference in porosities. Adsorption process is controlled by the diffusion of the fluoride ion in the liquid immediately adjacent the outer surface adsorbent material. Subsequently, fluoride ion must diffuse into the particle of adsorbent, which is the reason why the amount of fluoride ion adsorbed is less for the activated charcoal than for the activated alumina, since the phenome-non is influenced by the size of the pores through which the fluoride ion is transported.
CONCLUSION
γ-Al2O3 synthesized by the homogeneous precipitation method has good properties as an adsorbent material, which can be used to remove the fluoride ion present in drinking water. This material can remove up to 96% of fluoride ions in drinking water at pH 5 and 90% at pH 7. A higher zeta potential is observed than that for the activated charcoal, an increase in the adsorption due to the positive surface charge obtained at low pH, far away the isoelectric point (8.5). Activated carbon improved the behavior of fluoride ion removal slightly at pH 7 due to the increased surface charge. Removal process of the fluoride ion occurs on the outermost surface of the adsorbent material, and this is not affected by the difference in porosities. Thus, in this case study, activated charcoal does not appear to have the sufficient properties for the fluoride ion removal process. Although 58.6% of fluoride ions were removed at pH 7 from potable well water by the activated charcoal, it is not enough to comply with the Mexican Oficial Norm NOM-127-SSA1-1994 (1.5 mg • L-1).