INTRODUCTION
Only a small part of the generated organic fraction of urban solid wastes (OFUSW) and citrus wastes (CW) is currently recycled, composted or incinerated, and the unprocessed part is sent directly to sanitary waste disposal sites (Šan and Onay 2001). In Mexico City, citrus wastes are up to 10 % of the OFUSW (SEDEMA 2018), including orange, lemon, grapefruit, and tangerine, representing 60, 27, 8 and 7 % of the total CWs, respectively (SAGARPA-SIAP 2018). The juice manufacturing industry generates CW comprising the peel and pressed pulp, which usually accounts for 50-60 % of the whole fruit when processed for juice extraction (Ruiz and Flotast 2014). Some features of the CW can inhibit anaerobic digestion (AD); for instance, low pH (3-4), high total fiber content (570 g/kg peel dry weight), and essential oils (6.0 g/kg peel dry weight). Ninety percent of these essential oils is limonene, which has strong antimicrobial activity (Forgács et al. 2012, Ruiz and Flotast 2014). Two-stage AD has been proposed as an alternative method for the degradation of the organic fraction of OFUSW (Rodríguez et al. 2015). Hydrolysis is the first step in the overall AD process and is the rate-limiting step, especially if the substrate is in a solid form as in the case of OFUSW mixed with citrus wastes. To improve waste hydrolysis, various pre-treatments have been applied with the aim of facilitating the digestion process, making the substrate organic content more easily accessible to the microbial community (Moon and Song 2011). Solid-state fermentation (SSF) is widely used to grow filamentous fungi in processes that produce metabolites on various substrates that act as inducers during the production of enzymes (Darah et al. 2015). SSF is suitable for enzyme production with filamentous fungi since the low water availability reduces the possibility of contamination with bacteria or yeast (Attri et al. 2016). Some authors have obtained a fungal mass (FM) with high enzymatic activity using SSF with Aspergilllus CW (Biz et al. 2016). The enzymes produced by SSF on orange peels are more stable and efficient at the hydrolysis of orange peel than commercial enzymes, which in addition to their low production cost makes SSF the most feasible option (Awan et al. 2013, Biz et al. 2016).
The enzymatic hydrolysis of cellulose involves the sequential action of a group of enzymes known as cellulases, which belong to the family of glycosylhydrolases, so called because they catalyze the hydrolysis of the glycosidic acid between two and more carbohydrates and a hydrate. In general, the glycosidic link hydrolysis is catalyzed by two amino acids in the catalytic site, generally aspartate, and glutamate (Biz et al. 2016).
Pectinases are a group of enzymes of various types responsible for breaking down pectin to its elemental monomeric compounds. The enzymatic activities that act on the pectin backbone comprise hydrolases, which act on α-1,4-glycosidic bonds. Given their mode of action they can be endotypes if they act randomly along the main chain or exotypes if they act on the non-reducing ends of the oligogalacturonides (Awan et al. 2013).
Xylan, one of the major structural components of the plant cell wall and hemicellulose, is composed of 1,4-β-D-xylopyranose residues. Xylanases constitute a repertoire of hydrolytic enzymes that facilitate the complete hydrolysis of xylan (Antier et al. 1993). Exo-1,4-β-D-xylosidase (EC 3.2.1.37) catalyzes the hydrolysis of 1,4-β-D-xylo-oligosaccharides by removing successive D-xylose residues from the non-reducing end. The endoxylanases reported to release xylose during hydrolysis of xylan do not have any activity against xylobiose, which could be easily hydrolyzed by β-xylosidases. Proteases or peptidases are long known as enzymes that catalyze the rupture of peptide bonds into protein and peptide (Loera et al. 1999).
Research into the production of fungal enzymes from CW has focused primarily on orange, grapefruit, and tangerine peels separately. There is limited information regarding the hydrolysis of CW mixtures with the organic fraction of urban solid waste (SAGARPA-SIAP 2018, SEDEMA 2018). The aim of this work is to improve both the hydrolysis and acidogenesis rate of the OFUSW in an anaerobic hydrolytic leach bed (AHLB) reactor. This included a pretreatment stage of SSF using Aspergillus niger C28B25 directly on the citric wastes mix as a substrate to produce hydrolytic enzymes but also to reduce inhibitory essential oils such as limonene. The efficiency of this pretreatment was determined in the AHLB reactor whose configuration allows the drainage of hydrolysis and fermentation products.
MATERIALS AND METHODS
For this research, two types of waste were collected. Citrus waste was collected in the juice stands installed in the streets near the Universidad Autónoma Metropolitana, campus Iztapalapa (UAM-Iztapalapa), in Mexico City, and the OFUSW was collected from the waste of the lunch hour of the university cafeteria. Both residues were characterized at the beginning and end of each treatment in the pH, volatile solids (VS), total solids (TS) and chemical oxygen demand (COD).
Cultivation of the fungal seed
The fungus Aspergillus niger C28B25 has a generally regarded as safe (GRAS) status (Antier et al. 1993) and belongs to the UAM-Iztapalapa culture collection. The fungus was maintained on potato dextrose agar (PDA) medium at 4 ºC before use. This strain produces hydrolytic enzymes in SSF (Antier et al. 1993, Loera et al. 1999, Loera and Córdoba 2003). The fungus was reactivated in Czapek Dox Agar medium, in Petri dishes, and incubated at 30 º C for 14 days. After this time, the produced conidia were collected and transferred to Erlenmeyer flasks to further produce conidia for solid-state fermentation. The strain was cultured in 500 mL Erlenmeyer flasks, with 50 mL sterile Czapek Dox Agar culture medium incubated at 30 ºC for 7 days (Yamato, IC603CR incubator). After the incubation period, sterile 0.01 % Tween 80 solution and a magnetic stirrer were added to the flasks to harvest the conidia. The flasks were placed in a shaking rack to release conidia and obtain the conidia solution, which was filtered with gauze to remove medium residues. Conidia were counted in Neubauer chambers after 10- and 100-fold dilutions of the suspension (Loera and Córdoba 2003).
Sampling of citrus waste and solid-state fermentation
CW was collected in the juice stands installed in the streets near the University. The residues were taken to the laboratory and the mesocarp, skin, and seeds were manually removed. Only peel was used because it is where the highest concentration of essential oils and limonene may be found (Darah et al. 2015). The residues were weighed according to the urban proportion found as CW, as detailed below. Fresh, unsterilized waste was immediately ground in a mill (Torrey brand) to a particle size of 0.5 cm., and then it was mixed in proportions representative of the OFUSW, namely 60 % orange, 27 % lemon, 7 % grapefruit, and 6 % tangerine (SAGARPA-SIAP 2018). The pH, soluble COD, and total and volatile solids were determined in accordance with APHA-AWA-WPCF (2017); fat and fiber, limonene, cellulases, pectinases, xilanases, and proteases were determined following Mertens (2002, 2003), Davidowski and DiMarco (2009), Meraz et al. (2012), Darah et al. (2015), Beauchemin et al. (1996), and Lee et al. (1998), respectively. The substrate for SSF was the mixture of fresh and unsterilized CW, then SSF was performed in aerobic conditions using 500 mL Erlenmeyer flasks in triplicate. Each flask contained 16 g of fresh, non-sterile CW mixture. This quantity was chosen to avoid the problems of heat distribution and air flow. A single flask was used for each test carried out. Flasks were inoculated with 2 × 108 conidia per gram of dry matter (g/DM) and incubated at 30 ºC for 7 days, as this fungus has shown a maximum of enzyme production between days 5 and 7 (Antier et al. 1993, Patil and Dayanand 2006, Biz et al. 2016).
Enzymatic extraction and assays
Fifty mL of distilled water were added to each flask for enzyme recovery, then shaken with a magnetic stirrer at 200 RPM in an ice bath for 30 min. Enzymatic extracts were filtered with gauze and centrifuged at 8000 RPM in a Beckman J2-HS centrifuge for 10 min at 4 ºC to obtain a clear liquid. These extracts were assayed for enzymatic activities (Ordaz et al. 2016).
Cellulase and pectinase activities were measured as reducing sugars were released according to the dinitrosalicylic acid (DNS) method (Miller 1959) (1 g glucose/L, r = 0.998 [r is the Pearson’s correlation coefficient]) reported by Meraz et al. (2012) and Darah et al. (2015). Xylanase activity was measured using oat xylan as a substrate with the technique proposed by Beauchemin et al. (1996) (0.01 g caboximethylcellulose [CMC]/L, r = 0.999). Proteolytic activity was assayed as described by Lee et al. (1998). Enzymes were reported in units per grams of initial dry matter (U/gDM). The unit of enzyme activity (U) is defined as the amount of enzyme that catalyzes the conversion of 1 µmol of substrate in 1 min.
Extraction and quantification of limonene in CW
One gram of the CW mixture collected after removing the mesocarp, skins, seeds, and pulp residues, was ground in a mill (Torrey brand) to a particle size of 0.5 cm. Afterwards it was placed in a 7 mL vial with 5 mL of methanol and vortexed vigorously for 5 min (Davidowski and DiMarco 2009). Aliquots taken from the supernatant were filtered with a Millipore Sigma SX0001300 Swinnex syringe through a GSWP01300 MF-Millipore plain white mixed cellulose ester membrane, diluted 1:10 with methanol to determine the amount of limonene present in the CW mixture. Independent samples were analyzed in triplicate. The limonene was quantified using gas chromatography (Hewlett Packard Series II 5890) with a flame ionization detector and Agilent MS5 column. A standard curve was generated with 99 % pure reference limonene (Sigma-Aldrich) in concentrations from 0.03 to 1 g/L (r = 0.998). Measurement operating conditions consisted of a 275 ºC detector temperature, 275 ºC injector temperature, 3-min ramp (5 ºC/min) and a temperature increase of 45 ºC/min up to 275 ºC. Nitrogen was used as a carrier gas at a rate of 1 mL/min.
To evaluate the effect of the fungus on the limonene concentration during SSF, CW samples were collected at both the beginning and end of each of the cultures. For this purpose, 1 g of CW was collected after removing the mesocarp, skins, seeds, and pulp residues, and grounded in a mill (Torrey) to a particle size of 0.5 cm. At the end of the culture, 1 g of the CW with the fungus was also analyzed. The samples were processed in triplicate using the methodology described in the section Extraction and quantification of limonene in CW.
Sampling and characterization of hydrolysis and acidogenesis of the organic fraction of urban solid waste
The OFUSW was collected from UAM- Iztapalapa’s cafeteria at lunchtime. The pH, VS, TS, and total COD were determined at the beginning and end of each experiment (Choi et al. 2013, Ramírez et al. 2014, Rodríguez et al. 2015, Calabrò et al. 2016, 2017).
The AHLB reactors in figure 1 consisted of a cylindrical glass column with a total volume of 0.2 L and a useful volume of 0.15 L, with a supporting acrylic mesh in the bottom that allowed the passage of leachates into a conical reservoir for collection and analysis from the reactor. The upper part of the AHLB reactor is closed with a rubber plug and Tygon connections. Feeding of the solid sample is allowed, as well as leaching of liquid and separation of gases produced during the acidic hydrolytic process. All exits were perfectly sealed to preserve the anaerobic conditions (Jiménez et al. 2020).
The solid phase packed in the AHLB reactors were monitored at the beginning and at the end of the 14 days, to quantify the efficiency of COD removal and reduction of VS. The reactors were packed with 150 g of waste in the proportions described in table I. The R treatments were controls containing only OFUSW. The RC treatment columns contained OFUSW plus CW without fungal pretreatment and the RCA treatment contained OFUSW and FM (fungal mass, CW pretreated) as indicated in table I. Each treatment was performed in triplicate. To avoid inhibition, due to the accumulation of the produced volatile fatty acids and other soluble compounds, water was added as a draining liquid through the upper part of the reactor to drag the fermentation products out of the reactor in leachate form. The generated leachate had a high amount of soluble organic matter and was drained as previously reported (Rodríguez et al. 2015, Jiménez et al. 2020). The runoff liquid was 5 mL of tap water to promote waste degradation. The leachates produced during the hydrolytic step in each AHLB reactor were analyzed for pH, soluble COD, and volatile fatty acids (VFA) (Ramírez et al. 2014) as fermentation products to follow up on the hydrolytic process (Francoise et al. 2007).
TABLE I WASTE MIXTURES PACKED IN THE AHLB.
AHLB reactors | OFUSW (%) | CW (%) | Pretratment |
R | 100 | 0 | OFUSW |
RC | 90 | 10 | CW without pretreatment |
RCA | 90 | 10 | CW with pretreatment (FM) |
AHLB: anaerobic hydrolytic leaching bed reactor; OFUSW: organic fraction of urban solid waste; CW: citrus waste; FM: fungal mass; R: treatment with 100 % OFUSW; RC: treatment with 90 % OFUSW + 10 % CW without pretreatment; RCA: treatment with 90 % OFUSW + 10 % CW with fungal pretreatment.
Letters a, b, c indicate standard deviation between treatments (α = 0.05).
Treatment means were compared using one-way analyses of variance (ANOVA) with post-hoc Tukey comparisons. In all analyses the significance level was α = 0.05. All statistical analyses were carried out with the Number Cruncher Statistical System 19 (NCSS) software (Kaysville, Utah, USA).
RESULTS AND DISCUSSION
Quantification of enzymes produced by Aspergillus niger C28B25 on CW
The enzymatic activities of pectinases, cellulases, xylanases, and proteases at the end of the SSF are shown in table II. Xylanases had the highest activity, while proteases had the lowest. Pectinase activity (37 U/gDM) was similar to the one reported by Biz et al. (2016), who studied A. niger on citrus pulp. A higher pectinase activity was obtained (79 U/gDM) with grapefruit peels as the sole substrate by a wild strain of A. niger under optimal culture conditions (30 ºC, 6 days of fermentation, 1 × 108 conidia/gCW inoculum and particle size of 0.75 mm) (Darah et al. 2015). Grapefruit residues and other citrus fruits are substrates rich in lignocellulosic materials, including pectin, which act as inducers of pectinase production (Darah et al. 2013). In this work, nutrient enriched solutions were not added to the substrate, which is the main difference regarding other studies.
TABLE II ENZYMATIC ACTIVITIES OF Aspergillus niger C28B25 IN SOLID SUBSTRATE FERMENTATION ON CITRUS WASTE MIX.
Enzyme group | Enzymatic activity (U/gDM) |
Pectinases | 36 ± 7 |
Cellulases | 33 ± 6 |
Xylanases | 374 ± 54 |
Proteases | 0.215 ± 0.001 |
Cellulolytic activity was measured as 33 U/gDM, above the 9 U/gDM activity reported on orange peels in similar conditions (M’hiri et al. 2015). In another study, the production of cellulases was significantly higher (129 U/gDM), with orange peels supplemented with Mandels saline solution at pH 6 and 28 ºC after 7 days (Attri et al. 2016). Pectinase and cellulase activities in this study fell within the range reported by other authors. In fact, the low pH and the components of the CW favored production of xylanases in this work, wich was even higher than that reported by Janati et al. (2012) for orange peels alone (98 U/gDM). Some authors obtained multienzymatic complexes of pectinases (135 U/gDM), cellulases (66 U/gDM), and xylanases (79 U/gDM) (Choi et al. 2013). The differences between those results and this work were mainly in the optimization of conditions, while we used a mixture of fresh CW without supplementation. There are studies indicating that CW is a poor substrate to produce proteases (Maller et al. 2011). Nevertheless, this value is greater than observed here (0.2 U/gDM). This result is a key advantage since a high concentration of proteases can break down other enzymes formed during SSF (Soares et al. 2015). The activity produced by enzymes can vary depending on the microbial strain, the substrate, and the culture conditions, as well as the time used to produce the enzymes (Mamma et al. 2008, Ahmed et al. 2016). Table III shows the effect of SSF before and after the CW mix. The pH value in the mixture was in ranges reported by some authors who have worked with citrus peels separately. The pH of the mix was 4.8 while that of orange peels alone was between 3.5 and 4.3 (Abubakar and Oloyode 2013, Kanimozhi and Nagalakshmi 2014). Grapefruit peel has a pH value between 3.6 and 3.9 (Soares et al. 2015), lemon peel has a pH of 3.4 (Indulekha et al. 2017) and the pH of tangerine has not been measured before (Siles et al. 2016). The initial pH of this experiment was 4 before the SSF and 5.2 after the process, so these values allow enzymes to produce good activity. In general, the optimum pH is between 4.5 and 6 (Soares et al. 2015, Biz et al. 2016, León et al. 2017).
TABLE III COMPOSITION OF THE CITRUS WASTE MIX BEFORE AND AFTER THE SOLID SUBSTRATE FERMENTATION ON CITRUS WASTE (CW) MIX.
Parameter | TS (g/kg CW) | VS (g/kg CW) | Moisture (%) | Fiber (g/kg CW) | Fat (g/kg CW) |
Fresh waste | 280 ± 4 | 230 ± 1 | 72 ± 1 | 540 ± 6 | 0.5 ± 0.05 |
Fermented waste | 270 ± 4 | 250 ± 3 | 73 ± 3 | 437 ± 13 | 0.27 ± 0.02 |
TS: total solids; VS: volatile solids; CW: citrus waste.
The moisture value in the mixture was similar to that reported by other authors (75-83 %) (Abubakar and Oloyode 2013, Soares et al. 2015, Siles et al. 2016). Fiber is a substantial component of peels. It is a variety of non-starch polysaccharides including cellulose, hemicellulose, pectine, and lignin. These molecules will be degraded by hydrolytic enzymes from bacteria to obtain sugars for the formation of VFA. Fiber represents 540 g/kg in orange and 510-620 g/kg in lemon (Janati et al. 2012, Ruiz and Flotats 2014). Similarly, fiber in grapefruit peel makes up between 390 and 560 g/kg, though it has not been quantified in tangerine. Only two authors have studied the fat content in peels: Soares et al. (2015) in orange (0.8 %), and Indullekha et al. (2017) in lemon peels,, including mesocarp (4.8%). Values obtained for fat and fiber contents after SSF are presented in table III. SSF with A. niger favored a significant removal of fiber (20 %) and fat (46 %), which could in turn ease the hydrolysis of the CW mix. Fiber is broken down into various chemical compounds: gases (hydrogen, carbon dioxide and methane) and short-chain fatty acids (acetate, propionate, and butyrate), which is mainly reflected in an increase in the production of VFAs as part of the solubles of the hydrolitic process (Dahiya et al. 2015). Moon and Song (2011) observed that both the addition of enzymes and the AD in two stages are strategies that promote hydrolysis of OFUSW, obtaining more COD soluble per gram of SV. These authors showed that the mixture of lipases, cellulases, and proteases increases by more than 100 % the reduction in VS, which is mainly reflected in an increase in the production of VFAs as part of the solubles of the hydrolitic process, compared to the control experiment without addition of enzymes. Both experiments, with and without addition of enzymes, were carried out at a constant pH of 4.5 adding NaOH.
Limonene removal
A major limitation of using CW in biological processes relates to the high contents of essential oils, primarily D-limonene, a well-known cytotoxic that inhibits the activity of microorganisms involved in the fermentative processes (Loera et al. 1999). Some authors establish that the half-maximal inhibitory concentration (IC50) of limonene in batch experiments is 423 mg/kg, and its minimum inhibitory concentration, measured as limonene concentration in a reactor with orange peels and inoculated with sludge from a large-scale biogas production plant, is about 200 mg/kg (Ruiz and Flotast 2016). Figure 2 shows the removal of limonene in the mixture of the CW, evaluated at the end of the SSF (the black column represents limonene concentration without pretreatment and the gray column with pretreatment). The concentration of limonene decreased from 7.9 × 104 mg/kg CW to 0.3 × 104 mg/kgCW, corresponding to a 97 ± 0.2 %.
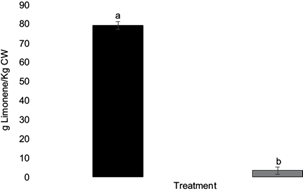
Fig. 2 Limonene concentrations in the citrus waste (CW) at the start and end of the solid state fermentation (SSF) with Aspergillus niger C28B25. Different letters above error bars of the standard deviation represent significant differences (α = 0.05). Black column is fresh citrus waste and gray column is fungal mass.
In the RCA reactors that were packed with a mixture of OFUSW and FM, initial limonene concentrations were 350 mg/kg of mixture; the process ended with 150 mg/kg of mixture, which represents a 57 % loss of limonene in the hydrolytic reactor. Meanwhile, initial limonene concentration in the RC treatment was 2.1 g/kg of mix and after 14 days it was 1.5 g/kg of mix, which represents a decrease of 28 %. In some laboratory a significant decrease in the limonene concentration (< 90 %) was observed after the sterilization of the CW; therefore, in this work fresh CW unsterilized was used to preserve the natural concentration of essential oils.
The limonene removal achieved in this work is two times higher than that obtained with orange peel (55 %) subjected to SSF with a mixture of Sporotrichum sp., Aspergillus sp., Fusarium sp., and Penicillium sp. (Srilatha et al. 1995). High value products such as biogas or ethanol are recoverable from CW, but the high content of limonene inhibits the process due to the antibiotic effect on microbial consortia (Mamma et al. 2008, Indulekha et al. 2017). This work shows that SSF, using a fresh CW substrate mixture, produced hydrolytic enzymes and reduced limonene, fiber, and fat content from the CW. Although the mechanism of action of the enzymes produced by A. niger is not completely clear, it has been seen that there is a decrease of limonene concentration in a fermentation processes in solid medium (Akao et al. 1992). Noma et al. (1992) observed the transformation of this compound by introducing a functional carbonyl group at carbon 3 of the limonene, forming less toxic compounds such as isopiperitenone (19 %), perililic alcohol (12 %), and (+)-cis-carveol (5 %) (Duetz et al. 2003).
Hydrolysis and acidogenesis of OFUSW in an AHLB reactor, adding fungal mass as a source of enzymes
Table IV presents the results of kinetics before and after 14 days of OFUSW fermentation for each of the treatments, including only treatments R, RC, and RCA, together with their effect on limonene concentration. There were significant differences between treatments R (100 % OFUSW) and RC (90 % OFUSW + 10 % CW without pretreatment), while RCA (90 % OFUSW + 10 % with FM) was significantly different from treatments without fungal mass (R and RC).
TABLE IV RESPONSE VARIABLES OF OFUSW WITH CW BEFORE AND AFTER 14 DAYS FERMENTATION IN AN AHLB.
Treatment | Time (days) | pH | TS (g/kg) | COD (g/kg) | Grease (g/kg) | Limonene (g/kg CW) | Reduction of TS (%) | Efficiency COD (%) | Reduction of grease (%) | Reduction of limonene ( %) |
R | 0 | 5.0 ± 0.05a | 224 ± 2a | 211 ± 3a | 18 ± 1a | -- | 12 ± 4a | 7 ± 2a | 6 ± 0.5a | -- |
14 | 3.5 ± 0.03 a | 197 ± 1a | 196 ± 3a | 17 ± 2b | -- | |||||
RC | 0 | 4.8 ± 0.03b | 241 ± 1b | 202 ± 8b | 19 ± 1a | 2.1 ± 0.6a | 7 ± 2b | 4 ± 2b | 0b | 28 ± 2b |
14 | 3.5 ± 0.03b | 217 ± 2b | 193 ± 8b | 19 ± 1b | 1.5 ± 0.4a | |||||
RCA | 0 | 4.5 ± 0.01c | 265 ± 6c | 181 ± 5c | 21 ± 3a | 0.35 ± 0.4b | 24 ± 5c | 23 ± 8c | 28 ± 2c | 57 ± 9a |
14 | 3.7 ± 0.01c | 181 ± 1c | 139 ± 5c | 16 ± 1c | 0.15 ± 0.04b |
COD: Chemical oxygen demand, TS: Total solids
TS: total solids; COD: chemical oxygen demand; CW: citrus waste; R: treatment with 100 % OFUSW; RC: treatment with 90 % OFUSW + 10 % CW without pretreatment; RCA: treatment with 90 % OFUSW + 10 % CW with fungal pretreatment.
Letters a, b, c indicate standard deviation between each of the treatments (α=0.05).
Due to the diversity of diets in different countries, OFUSW composition may vary; however, most researchers consider that the characteristic values of the OFUSW are the same as those obtained in this study in terms of TS, COD and pH (Kim et al. 2006, 2011, Zhang et al. 2007, Bo and Pin-Jing, 2014, Rodríguez et al. 2015). The high values of COD and volatile solids allow OFUSW to be a good substrate for AD processes. In the hydrolytic stage of anaerobic digestion there are diverse communities of anaerobic bacteria which produce exoenzymes that perform the hydrolytic step (Adekunle and Okolie 2015); thus, this stage is rate-limiting for solid residues (Patinvoh et al. 2017). Many studies have investigated pretreatment methods that accelerate the velocity of hydrolysis and result in suitable by-products (Ariunbaatar et al. 2014).
In this context, an enzymatic pretreatment step is required to reduce substrate limitations before AD (Brémond et al. 2018). The quantity of limonene into reactors after SSF with and without fungal pretreatment is shown in table IV. Even if in this work the concentration of limonene was 140 mg /kgCW at the beginning of the OFUSW fermentation, a value below that considered inhibitory (200 mg/kg CW mixture), the limonene concentration in the mixture of CW residues without fungal treatment was up to 4700 mg/kg CW mixture, one order of magnitude greater than the maximum value recognized as inhibitory (423 mg/kg) (Ruiz and Flotats 2016).
In the hydrolysis and acidogenesis stages there is no significant production of methane, due to pH conditions (Adekunle and Okolie 2015). The efficiencies of volatile solids (VS) and COD reductions were greater in the AHLB reactor that contained the FM (Aspergillus niger C28B25 plus fungal enzymes), showing that SSF favored hydrolysis of compounds present in the OFUSW. Moon and Song (2011) observed that by applying a mixture of enzymes, a decrease of more than 50 % in VS and an increase in COD could be achieved compared to experiments without the addition of enzymes. The low concentration of limonene in the RCA mix (350 mg/kg) improved the hydrolysis of the OFUSW. In contrast, the efficiencies of reducing VS and removing COD were very low in the treatment containing 10 % of CW without pretreatment (RC). The initial limonene content was 2.1 g/kg mix and at the end of the 14 days it was 1.5 g /kg of mix. This validates the harmful effects of compounds such as limonene that inhibit microbial activities in the OFUSW. Additionally, the raw fiber in the CW hindered the hydrolysis. The fungal mass treatment (RCA) significantly improved all variables, e.g., a seven-fold reduction in VS with respect to the RC treatment. The same behavior was observed with the removal efficiency of total COD, which was five-fold greater than with the RC treatment. Some authors suggest that it is necessary to improve hydrolysis by means of pretreatments such as hydrolytic enzymes, for instance when using citrus wastes, since these pretreatments contribute to the digestion of hard materials (Ruiz and Flotast 2014, Biz et al. 2016). SSF is a viable option to obtain these enzymes. In addition to the low-cost of the SSF process, the fermented substrates can be added directly to the reaction mixture, which avoids the complex step of extracting the enzymes (Biz et al. 2016, Brémond et al. 2018). Some studies aiming to improve the hydrolysis of OFUSW have reported that adding hydrolytic enzyme extracts and anaerobic sludge reduced VS up to 50 % (Kim et al. 2006, Moon and Song 2011, Kiran et al. 2015). AHLB reactors in these studies were not inoculated; however, adding hydrolytic enzymes to the OFUSW mixtures improved the action of native microorganisms present in the waste.
Leachate composition from the different treatments
The design of the AHLB reactor used to carry out the fermentation allowed the solubilization of organic matter present in the solid waste, which in fact was drained as leachate. Soluble COD in the leachate is an indicator of the products of hydrolysis and acidogenesis, released by the enzymes added with the FM and by the microorganisms present in the OFUSW. The released sugars are easily fermented to VFAs and other low molecular weight compounds (Francois et al. 2007, Ramírez et al. 2014, Rodríguez et al. 2015). Figures 3 and 4 present the changes in soluble COD and VFA in the leachate from the different treatments. The values of these changes were significantly greater in the RCA treatment due to a better hydrolysis of solid residues, the solubilization of organic matter and the fermentation of sugars to VFAs. Thus, when FM was added after the SSF pretreatment, there was a higher rate of COD change and VFAs.

Fig. 3 Production rates of chemical oxygen demand for each treatment. White column: R treatment (100 % organic fraction of solid urban waste [OFUSW]); gray column: RC treatment (OFUSW + 10 % citrus waste [CW] without pretreatment); black column: RCA treatment (OFUSW + 10 % CW with fungal pretreatment). Different letters above error bars of the standard deviation represent significant differences (α = 0.05).
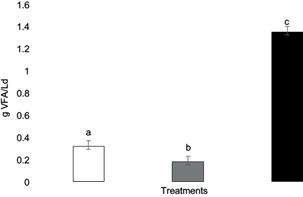
Fig. 4 Production rates of volatile fatty acids (VFA) for each treatment. White column: R treatment (100 % organic fraction of solid urban waste [OFUSW]); gray column: RC treatment (OFUSW + 10 % citrus waste [CW] without pretreatment); black column: RCA treatment (OFUSW + 10 % CW with fungal pretreatment). Different letters above error bars of the standard deviation represent significant differences (α = 0.05).
Some authors have performed the AD of OFUSW using anaerobic sludge as inoculum in the AHLB reactor, obtaining lower VFA production values (0.21 g VFA/Ld) (Ramírez et al. 2014, Rodríguez et al. 2015). Experiments carried out on anaerobic hydrolysis of the OFUSW under alkaline thermophilic conditions had a greater VFA production rate (0.75 g VFA/Ld) than under mesophilic conditions and acidic pH (0.4 g VFA/Ld) (García et al. 2017). Jiménez et al. (2020) obtained 0.82 gVFA/Ld in the hydrolysis of aerobically pretreated OFUSW. VFA production rates in this work were greater in the RCA treatment (R = 0.3 g VFA/Ld, RC = 0.1 g VFA/Ld, and RCA = 1.4 g VFA/Ld). The significantly different results in COD production show the effect of pretreatment. The best result was obtained with the RCA (4.8 g/Ld), while values of the other treatments were lower (R = 1.3 g/Ld; RC = 0.8 g/Ld). López and Espinosa (2008) obtained an 18 % improvement in COD production after waste pretreatment. Jiménez et al. (2020) observed that the addition of enzymes in the hydrolytic process produced a significant two-fold increase in the hydrolysis compared to control experiments (77 and 44 g/L, respectively). The above results suggest that under appropriate pretreatment, complex organic matter becomes more biodegradable by roughly changing chemical structure. The pretreatment may enhance the surface area available for further microbial enzymatic attack to release organic matter.
CONCLUSIONS
The results obtained in this work show the benefits of SSF carried out with fresh citrus peels without sterilization and the addition of buffer solutions or supplements. The increase in the hydrolysis and acidogenesis of the OFUSW is explained by the effectiveness of this pretreatment based on SSF, due to the fact that Aspergillus niger C28B25 simultaneously produced hydrolytic enzymes and removed limonene, which in turn increased the soluble material available for other microorganisms and then improved the reduction of solids and the efficiency of COD removal during the fermentation of OFUSW. The results of VS and fiber degradation were associated with greater amounts of COD and VFA in the leachate. The AHLB reactor operated well without any inoculant addition, representing an important innovation for AD of the OFUSW. The leachate containing high COD and VFA concentrations make it an ideal material for biogas production in high-rate reactors. In the methanation process, it is expected that the leachates produced by the RCA treatment will produce more methane than the leachates produced by reactors without pretreatment.