INTRODUCTION
The exact amount of synthetic organic dyes produced in the world is unknown, but more than 1 million tons per year (Ali 2010) are used in the textile, leather-tanning, paper production, food technology, photoelectrochemical cell, and hair coloring industries.
Azo, anthraquinone, sulfur, indigoid, triphenylmethyl (trityl), and phthalocyanine derivatives represent the most commonly used dyes on the industrial scale (Forgacs et al. 2004). Due to their large-scale production and extensive application, these synthetic dyes can cause considerable environmental pollution (Nawaz and Ahsan 2014) and serious health risks (Forgacs et al. 2004, de Jager et al. 2014).
Indigo blue dye is the main raw material used in the process of denim dyeing. This dye contains a ketonic group (C=O), and is water-insoluble. Wastewater containing indigo blue dye is characterized by a dark blue color due to the presence of the residual dye that was not fixed to the fiber during the dyeing process (Albuquerque et al. 2013) and the presence of unreactive hydrolyzed dye in the dyebath (Pearce et al. 2003). Dye loss in the effluent can range from 2 % to 50 % depending on the dye type (Pearce et al. 2003, Forgacs et al. 2004, Martínez-Huitle and Brillas 2009, Punzi et al. 2012, Khandegar and Saroha 2013). The poor biodegradability of denim wastewater is caused by the presence of color, nutrients (nitrogen and phosphorus), inorganic salts, refractory organics (Karthikey et al. 2011, de Jager et al. 2014), total suspended solids (TSS), chemical oxygen demand (COD), variable pH (2 to 12), and toxic compounds such as surfactants, heavy metals, and chlorinated organic compounds (Raghu et al. 2009, Khandegar and Saroha 2013, Blanco et al. 2014, Manenti et al. 2014). It is estimated that 280 000 t of textile dyes are discharged in industrial textile effluent every year worldwide (Ali 2010).
Various physical, chemical, biological, enzymatic, and electrochemical technologies have been widely used to treat textile effluents (Martínez-Huitle and Brillas 2009). Among these, ferrate oxidation is considered to be an environmental friendly technique for pollution control and an efficient tool for dye degradation. The oxidizing power of ferrate is higher than those of common oxidants such as permanganate, ozone, and hypochlorite (Li et al. 2005, Eng et al. 2006, Han et al. 2018, Rai et al. 2018).
During the oxidation of dyes, ferrate is reduced to Fe(III); therefore, ferrate exhibits coagulating properties as a consequence of ferric hydroxide (Fe(OH)3) formation. This coagulation can greatly enhance the aggregation and settling processes (Eng et al. 2006, Alsheyab et al. 2009, Rai et al. 2018, Shin et al. 2018), and is considered beneficial for water treatment processes. A variety of industrial wastewater pollutants have been treated by ferrates, including inorganic contaminants, nitrogen-containing pollutants, organosulfur compounds, nutrients, some metals, emerging pollutants, and microorganisms (Eng et al. 2006, Nikolić-Bujanović et al. 2011, Villanueva-Rodríguez et al. 2012, Han et al. 2018).
There are two basic methods for ferrate production: chemical and electrochemical methods. The chemical methods are based on combining iron compounds, such as iron (III) nitrate and iron oxide, with an oxidizing material in an alkaline environment. In contrast, electrochemical methods usually involve the use of a sacrificial iron anode in an electrolysis cell containing a strongly alkaline solution such as NaOH or KOH. A direct current is applied to oxidize the Fe0 to Fe(VI) (De Koninck and Bélanger 2003, Alsheyab et al. 2009, Mácová et al. 2009, Villanueva-Rodríguez et al. 2012).
On this basis, the use of electrochemically synthesized sodium and potassium ferrate was proposed for the treatment of indigo blue aqueous solutions and denim wastewater. The electrochemical synthesis was carried out using a batch cell and a Fe-BDD (boron doped diamond) anode/cathode. The synthesis of ferrate ions by electrochemical means depends strongly on the applied current density, electrode material, active area, temperature, reactor design, iron concentration, and electrolyte used. These parameters were evaluated using a 24 factorial design. It is important to note that while the great majority of the studies using sodium or potassium ferrate have been carried out only in an aqueous solution, in this work, the ferrate compounds were applied to real wastewater, giving this work additional significance.
MATERIAL AND METHODS
Electrochemical synthesis of ferrate
The electrosynthesis was accomplished according to the 24 factorial shown in table I. The variables of temperature, current density, and the type and concentration of the electrolyte were analyzed and contrasted using the Yates algorithm and the “F” test to determine which had the main effect on the electrosynthesis of ferrate. The experiments were carried out in an electrochemical batch cell using iron as the anode and BDD as the cathode; the anodic surface area was 12 cm2. 30 mL of the electrolyte was placed in the electrosynthesis reactor, and the current intensity was controlled using a power supply and a digital multimeter. The concentration of ferrate was determined by measuring the absorbance at 505 nm using a Varian Cary E1 spectrophotometer, and applying a molar absorption coefficient of 1150 1/M/cm (Lee et al. 2005, Ramseier Peter et al. 2011, Sharma 2013).
Characterization of ferrate (VI)
Ferrate(VI) was characterized by three analytical techniques: a) infrared spectroscopy (IR) to observe the characteristic functional groups at 324 and 800 1/cm; b) cyclic voltammetry (CV), an electroanalytical technique used for the determination of compounds that are oxidized or reduced electrochemically, and c) ultraviolet-visible spectroscopy (UV-Vis), which provides information via the characteristic absorption peak at 505 nm.
Indigo blue aqueous solutions
Indigo blue dye was obtained from an industrial dyeing operation located in the State of Mexico, and was characterized using IR spectroscopy and UV-Vis spectroscopy in a previous report (Almazán-Sánchez et al. 2016). For this, 10, 30, 40, 50, and 100 mg/L aqueous solutions of the dye were prepared (pH = 8.0), and the concentration was determined by UV-Vis spectrometry at 591 nm, based on the previous results.
Textile wastewater sample
A wastewater sample from the effluent of an industrial textile operation located in the State of Mexico was collected in plastic containers. The following parameters of the sample were analyzed: temperature, biochemical oxygen demand (BOD5), chemical oxygen demand (COD), total organic carbon (TOC), color (Pt-Co units), turbidity, pH, sulfates (SO4 2-), ammonia nitrogen (NH4 +), total suspended solids (TSS), methylene blue active substances (MBAS), nitrates (NO3 -), nitrites (NO2 -), total phosphorus, total solids (TS), total dissolved solids (TDS), oil and grease, residual chlorine, magnesium, sodium, and calcium, according to standard methods and procedures (APHA 2012). The sample was also analyzed using IR spectroscopy and UV-Vis to determine the mechanism of dye removal. The same parameters were analyzed in the treated wastewater in order to evaluate the efficiency of ferrate.
Treatment of the aqueous solutions and wastewater by sodium and potassium ferrate
A jar test was used to determine the optimum time and ferrate dose for the treatment of the aqueous solutions and denim wastewater. Batch experiments were carried out by combining 8 mL of the indigo blue aqueous solutions with different initial concentrations (10, 30, 40, 50, or 100 mg/L) with 25, 50, 100, 200, or 300 mg/L of previously synthesized potassium and sodium ferrate. The solutions were mixed rapidly (250 rpm) for 1 min, and then at a lower rate (50 rpm) for 26 h. Aliquots were collected at different times (1, 2, 3, 4, 5, 6, 7, and 26 h) and the concentration of indigo blue was evaluated in order to calculate the removal percentage at the end of the treatment. For the denim wastewater, the treatments were carried out under the same conditions, and the color removal efficiency was evaluated after 1 h. All experiments were carried out in triplicate.
RESULTS AND DISCUSSION
Electrochemical synthesis of ferrate
The effect of different variables in the electrosynthesis of ferrate was evaluated using a 24 factorial design. The effect of each factor was analyzed separately in order to determine its influence on the process. Table II shows the optimal operational conditions for the electrochemical processes. According to the results, the best ferrate yield was obtained in experiment 15, using 20 M NaOH, and applying a current density of 100 mA/cm2 at room temperature (25 ºC).
TABLE II RESULTS OF FERRATE SYNTHESIS, ACCORDING TO 24 FACTORIAL DESIGN. STUDIED VARIABLES: A = ELECTROLYTE TYPE (NaOH OR KOH), B = ELECTROLYTE CONCENTRATION (14 AND 20 M), C = CURRENT DENSITY (30 AND 100 MA/CM2), D = TEMPERATURE (4º C AND ROOM TEMPERATURE)
Experiment | Interaction | Factors | (FeO4)2- (g/L) | Energy consumption (kWh/kg) | |||
A | B (M) | C (mA/cm2) | D (ºC) | ||||
1 | M* | NaOH | 14 | 30 | 4 | 0.20 | 10.86 |
2 | A | KOH | 14 | 30 | 4 | 0.04 | 44.86 |
3 | B | NaOH | 20 | 30 | 4 | 1.42 | 1.53 |
4 | AB | KOH | 20 | 30 | 4 | 0.05 | 37.81 |
5 | C | NaOH | 14 | 100 | 4 | 0.81 | 17.03 |
6 | AC | KOH | 14 | 100 | 4 | 0.15 | 90.43 |
7 | BC | NaOH | 20 | 100 | 4 | 0.58 | 12.50 |
8 | ABC | KOH | 20 | 100 | 4 | 0.33 | 27.24 |
9 | D | NaOH | 14 | 30 | RT | 1.32 | 1.65 |
10 | AD | KOH | 14 | 30 | RT | 0.25 | 5.69 |
11 | BD | NaOH | 20 | 30 | RT | 2.31 | 0.63 |
12 | ABD | KOH | 20 | 30 | RT | 0.20 | 6.89 |
13 | CD | NaOH | 14 | 100 | RT | 1.38 | 4.43 |
14 | ACD | KOH | 14 | 100 | RT | 0.42 | 21.94 |
15 | BCD | NaOH | 20 | 100 | RT | 6.89 | 2.01 |
16 | ABCD | KOH | 20 | 100 | RT | 0.31 | 44.49 |
* M is the standard order. It refers to the experiment in which all the variables are in the low level, RT = room temperature
Figure 1 shows that the highest production of ferrate was achieved by applying a current density of 100 mA/cm2, with the yield of ferrate reaching 6.89 g/L (41.54 mM). At a current density of 30 mA/cm2, only 2.31 g/L (13.92 mM) of ferrate was synthesized using NaOH as the electrolyte, as shown in table II. Many studies have reported the synthesis of ferrate under different experimental conditions, some of which obtained 2.03, 1.91, and 1.74 mM of ferrate in 20 M NaOH media with current densities of 4.55, 3.08, and 1.47 mA/cm2, respectively (Barışçı et al. 2014). Alsheyab et al. (2010) achieved concentrations in the range 0.54-3.25 mM by applying current densities from 1.8 to 18.1 mA/cm2 in 14 M NaOH. Other authors achieved yields of 7.3 and 1.5 mM at 3.3 and 0.5 mA/cm2 in 16 M NaOH (Ding et al. 2004). Finally, a concentration of approximately 0.23 mM was achieved at 125 mA/cm2 using 14 M KOH (Sánchez-Carretero et al. 2010).

Fig 1 Current density effect. a) 100 mA/cm2, b) 30 mA/cm2. Electrolyte type (A), electrolyte concentration (B), current density (C) and temperature (D)
Different mechanisms have been proposed to explain the process of ferrate production in the electrochemical cell; equations 1-7 (Mácová et al. 2009) describe anodic dissolution and involve the formation of a passivating layer over the electrode, reducing the ferrate yield. This film consists of FeOOH and Fe(OH)2 (Eq. 2-3), which block the flow of electrons. Additionally, Fe3O4 (Eq. 1) can act as a barrier against further iron dissolution and prevent the formation of ferrate; it also competes with oxygen evolution (Eq. 7). The hydroxyl ions promote the reaction by dissolving the oxide/hydroxide layer, as shown in equations 4-6. Hydrogen gas is produced at the cathode in the global reaction (Barışçı et al. 2014). The electrochemical reactions generated at the anode and cathode are shown in figure 2 (Zou and Chin 1988, Jiang and Lloyd 2002, Alsheyab et al. 2010). The sacrificial iron anode is oxidized from iron (0) to iron (VI) in the presence of hydroxyl ions, and simultaneous oxygen evolution and gas production take place. The energy consumption of the reaction was 2.01 KWh/kg, which was in agreement with the report of Barışçı et al. (2014).
The type of electrolyte used affects iron dissolution, the formation of the oxide/hydroxide layer, and the solubility of the dissolution products. Some researchers have suggested that NaOH is the best electrolyte for the electrochemical preparation of ferrate (Bouzek et al. 1999, Bouzek and Bergmann 1999, Ding et al. 2004, Xu et al. 2009, Barışçı et al. 2014), while others have reported that better electrosynthesis results were obtained using KOH (He et al. 2005). Na2FeO4 remains soluble in saturated aqueous NaOH solutions, but K2FeO4 is insoluble in a saturated KOH solution (Jiang and Lloyd 2002). In this study, NaOH was found to be the best alkaline medium, as shown in figure 3a and 3b. When KOH was used, only 0.42 g/L of ferrate was produced.
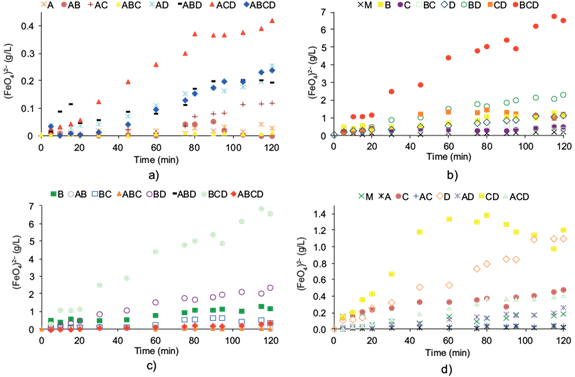
Fig 3 Electrolyte effect. a) KOH (concentration of electrolyte: 14 and 20 M, current density: 30 and 100 mA/cm2, T= 4 ºC and room temperature), b) NaOH (concentration of electrolyte: 14 and 20 M, current density: 30 and 100 mA/cm2, T= 4 ºC and room temperature), c) 20 M (electrolyte: NaOH and KOH, current density: 30 and 100 mA/cm2, T= 4 ºC and room temperature), d) 14 M (electrolyte: NaOH and KOH, current density: 30 and 100 mA/cm2, T= 4 ºC and room temperature). Electrolyte type (A), electrolyte concentration (B), current density (C) and temperature (D)
According to the previous equations, ferrate production increases with increasing HO- ion concentration. A concentrated medium increases the solubility of lower-valence iron species and promotes ferrate stability (Zou and Chin 1988, He et al. 2006, Mácová et al. 2009). When KOH is used at high concentrations (over 500 g/L), the oxides and hydroxides formed crystalize on the anode surface (Bouzek et al. 1999). In this research, concentrations of 14 M and 20 M were evaluated. Using 20 M NaOH, the maximum ferrate concentration of 6.89 g/L was obtained (Fig. 3c), while a concentration of 1.38 g/L was obtained using 14 M NaOH (Fig. 3d).
Changing the temperature could induce the depassivation of the anode surface. The influence of temperature on electrochemical ferrate production has previously been reported by several researchers (Beck et al. 1985). For instance, Barışçı et al. (2014) studied the effect of using temperatures of 20, 30, 50, and 65 ºC with high-purity iron anodes. The highest production rate after 75 min was obtained at 65 ºC, and the lowest production rate was observed at 20 ºC. They concluded that higher temperatures led to better production in ferrate synthesis using high-purity iron anodes. In this work, similar behavior was observed; the maximum ferrate concentration was achieved at room temperature (25 ºC), whereas at 4 ºC, only 1.42 g/L was obtained (Fig. 4).

Fig 4 Temperature effect. a) 4ºC, b) room temperature. Electrolyte type (A), electrolyte concentration (B), current density (C) and temperature (D)
The results were validated using the Yates algorithm to explain the synergic effect of all the operational variables. The Yates equation obtained is as follows:
Where:
A (type of electrolyte) = -1.63 g/L, B (concentration of electrolyte) = 0.98 g/L, C (current density) = 0.54 g/L, and D (temperature) = 1.09 g/L.
Based on the electrolyte type parameter (A), changing the electrolyte from NaOH electrolyte to KOH reduced the ferrate concentration. When the concentration of electrolyte (B) was increased from 14 to 20 M, the synthesis of ferrate was enhanced. Increasing the current density (C) from 30 to 100 mA/cm2 had a positive effect on the electrochemical process, improving the production of ferrate. Increasing the temperature (D) from 4 ºC to room temperature (25 ºC) also enhanced the concentration of ferrate.
Table III shows the effects calculated by the Yates algorithm; through these data, the sum of squares, estimated coefficients, standard deviation and the “F” test were determined. Variables B, C and D have effects on both levels in the electrochemical synthesis of ferrate (VI); while the variable A (type of electrolyte) is optimal with NaOH at the low level.
TABLE III STATISTICAL ANALYSIS IN THE ELECTROCHEMICAL SYNTHESIS OF FERRATE
Interaction | Effect | Sum of squares | Estimated coefficients | Standard deviation | “F” Test |
M | 1.03 | 0.44 | 2.96 | ||
A | -1.64 | 10.73 | -0.82 | 0.44 | -1.86 |
B | 0.93 | 3.47 | 0.47 | 0.44 | 1.06 |
AB | -0.97 | 3.76 | -0.48 | 0.44 | -1.10 |
C | 0.60 | 1.43 | 0.30 | 0.44 | 0.68 |
AC | -0.48 | 0.90 | -0.24 | 0.44 | -0.54 |
BC | 0.41 | 0.66 | 0.20 | 0.44 | 0.46 |
ABC | -0.33 | 0.44 | -0.17 | 0.44 | -0.38 |
D | 1.15 | 5.29 | 0.58 | 0.44 | 1.31 |
AD | -1.04 | 4.35 | -0.52 | 0.44 | -1.19 |
BD | 0.65 | 1.71 | 0.33 | 0.44 | 0.74 |
ABD | -0.70 | 1.94 | -0.35 | 0.44 | -0.79 |
CD | 0.63 | 1.60 | 0.32 | 0.44 | 0.72 |
ACD | -0.61 | 1.51 | -0.31 | 0.44 | -0.70 |
BCD | 0.91 | 3.32 | 0.46 | 0.44 | 1.04 |
ABCD | -0.81 | 2.63 | -0.41 | 0.44 | -0.92 |
In conclusion, the maximum experimental concentration of ferrate produced in 180 min was 6.89 g/L, using 20 M NaOH, a current density of 100 mA/cm2, and room temperature (25 ºC).
Ferrate characterization
Ferrate (VI) has a characteristic purple-violet color, which corresponds to a visible and near-infrared absorption spectrum from about 450 to 600 nm. Figure 5a shows the UV/Vis spectra of electrogenerated Na2FeO4 and K2FeO4 in 20 M media. The spectra of both ferrates showed maximum absorption at 505 nm. These spectra were compared with that of a commercial ferrate, and were found to contain the same features. These results agree with those of Licht et al. (2001), Jiang and Lloyd (2002) and Barışçı et al. (2014), who all observed the same wavelength for ferrate.

Fig 5 Ferrate characterization: a) ultraviolet visible spectra, b) infrared spectra, c) cyclic voltammetry (“a” NaOH 20 M, “b,” “c,” and “d”: 1, 2 and 3 mL of ferrate were added)
In addition, ferrates have also been characterized using Fourier transform infrared (FTIR) spectra, in which they display characteristic absorption bands at 925 and 523 cm-1 in the infrared region with triplet splitting (Fig. 5b; Sharma 2013).
Finally, the cyclic voltammetry (CV) technique was used to determine the redox behavior of the different compounds. Figure 5c shows the CV obtained at a scan rate of 100 mV/s using Ag/AgCl as the reference electrode, iron as the working electrode, and carbon paste as the counter electrode. Curve “a” shows only the 20 M NaOH. In curves “b,” “c,” and “d,” different concentrations of ferrate were added, and four peaks were obtained; the peaks numbered 1 and 2 correspond to the redox pair Fe2+/Fe3+. This pair exhibits a quasi-reversible one-electron charge transfer, while peak 3 is associated with the ferrate ion formation at -1.36 V (Licht et al. 2001, de Koninck and Bélanger 2003, Barışçı et al. 2014).
Aqueous solution treatment (Jar test)
The dye removal percentages for the aqueous indigo blue solutions using Na2FeO4 and K2FeO4 are presented in figure 6. The maximum dye removal percentages (over 77 %) were achieved using Na2FeO4 (300 mg/L dose) for all dye concentrations, while only 94 and 96 % dye removal was achieved for 20 and 30 mg/L dye concentrations at a dose of 200 mg/L. Na2FeO4 showed the better results at high doses only (Fig. 6a). Based on these results, the removal percentage strongly depends on the dose of ferrate. Very high doses of ferrate proved to be the most effective in reducing the organic concentration, because for a given volume of solution, proportionally increasing the quantity of the reactants could promote the probability of collision between the target pollutant and the oxidant, leading to an increase in the degradation efficiency (Jiang 2007, Han et al. 2013).

Fig 6 Removal percentage. Aqueous solution: a) sodium ferrate, b) potassium ferrate. Denim wastewater: c) sodium ferrate, d) potassium ferrate
As shown in figure 6b, K2FeO4 was superior at low dye concentrations (10, 20, and 30 mg/L) using intermediate doses. When the dye concentration was 10 mg/L, doses of 25, 50, and 100 mg/L of K2FeO4 gave the best dye removal results (76.9, 79.5, and 87.5 % respectively). Using dye concentrations of 20 and 30 mg/L and a ferrate dose of 200 mg/L, around 70 % color removal was achieved. When the ferrate dose was increased to 300 mg/L, 90 % color removal was achieved. Li et al. (2009) treated a 50 mg/L aqueous solution of an azo dye (orange II) with 10 mg/L K2FeO4 and 62 % color removal was obtained. Xu et al. (2009) treated the azo dye X-3B red in aqueous solution (initial concentration 0.08 mM) with 25 mg/L K2FeO4, achieving 95 % color removal at pH 10.
Ferrate ion is a strong oxidant over the entire pH range: Its redox potentials are 2.20 and 0.72 V in acidic and basic media (Eqs. 9-10), respectively.
The pH has a great effect on the decolorization of indigo blue dye by ferrate (Li et al. 2009, Xu et al. 2009) due to its effect on the redox potential and ferrate stability. The oxidation potential of ferrate decreases with increasing pH, because although ferrate is a more powerful oxidant at pH < 6, it is also highly unstable under these acidic conditions. Conversely, it becomes more chemically stable but has a weaker oxidizing ability at pH > 9. Thus, the optimum pH range is 6-9; the removal efficiency is low above and below this pH. In the experiments, the pH was increased to 11-12, as increasing the pH can cause the hydrolysis of Fe compounds to Fe(OH)3 (aq) or colloids, which are capable of adsorbing dye molecules and removing them from the solution.
In the current research, testing was carried out at room temperature. However, in accordance with the report of Han et al. (2013), increasing the temperature of the reaction medium would enhance the probability of collision between the target pollutant and oxidizing agent, and thus accelerate the reaction rate.
Figure 7 shows the possible mechanisms for the oxidation of denim blue by ferrate. In the first step, the destabilization of the first N-H bond occurs in the presence of ferrate, free radicals are formed, and ferrate is reduced from Fe(VI) to Fe(V). In the next step, the destabilization of the second N-H bond occurs through FeO4 -, and a conjugated double bond is formed. Then, the decomposition of FeO4 - to Fe(OH)3 and O2 in the basic media (OH-) occurs, and the interaction between oxygen and water breaks the conjugated bond, regenerating the basic media. Finally, organic by-products are formed. Oxidation and coagulation are thus the main mechanisms of the dye removal process.
Denim wastewater
Denim wastewater characterization
An effluent sample was collected from an industrial textile operation located in the State of Mexico, which operates in batch processes with an effluent volume of 11 600 L/d. The most significant parameters were determined before treatment and are shown in table IV. The wastewater generated by the textile industry includes cleaning wastewater, process wastewater, noncontact cooling wastewater, and storm water (Verma et al. 2012). The effluent from the denim processing is discharged into sewers and flushed into a local lagoon. The presence of nitrites in the water indicated biochemical and bacterial contamination. The high NH4 +, residual chlorine, and phosphate ions (PO4 3-) concentration contribute to lagoon eutrophication and toxicity; the presence of grease reduces the dissolved oxygen and can cause fish death. According to Mexican regulations, the PO4 3-, oil and grease, and TSS contents exceeded the allowed limits (30, 25, and 125 mg/L, respectively), and these levels reduced the visibility and increased the turbidity of the water. The BOD5/COD index was 0.2. Finally, the NO3 -, PO4 3-, TSS, and Mg2+ levels were higher than the typical values given by Ciabatti et al. (2010). The chemical species diagrams were analyzed to determine the form of the chemicals in the denim wastewater, taking into account the concentration of the majority ions. The high concentrations of the ions Na+, PO4 3-, Mg2+, SO4 2-, NO3 -, and Ca2+ promote the formation of MgHPO4, MgSO4, CaSO4, and Ca5(PO4)3OH(s) at the initial pH, while Na+, K+, and NO3 - remain in solution.
TABLE IV Raw and treated denim wastewater characterization and comparison with typical values from textile wastewater
Parameter (mg/L) | Initial | Ciabatti et al. (2010) | Final | Removal percentage |
Temperature | 18.2 | --- | 18 | --- |
pH | 6.37 | 6.5-8.5 | 11.47 | --- |
Turbidity (NTU) | 178 | 15-200 | 7.09 | 96.02 |
Nitrates | 2.95 | 0.5-1.5 | 1.48 | 50.16 |
Total organic carbon | 680 | 243.92 | 64.13 | |
Chemical oxygen demand | 734.13 | 550-1000 | 320.9 | 56.29 |
Sulfates | 118.73 | 400-600 | 89.99 | 24.83 |
Biochemical oxygen demand | 151.91 | --- | 315.30 | |
Phosphates as P | 52.5 | 1-20 | 42 | 20 |
Ammonia nitrogen | 40 | --- | 19.8 | 50.5 |
Color (Pt-Co) | 590 | --- | 75 | 92.51 |
Nitrites | 3.33 | --- | 0.52 | 94.39 |
Methylene blue active substances | 0.09 | --- | 0 | 100 |
Total suspended solids | 405 | 100-400 | 233 | 42.49 |
Total dissolved solids | 1638 | --- | 1454.82 | 11.19 |
Total solids | 2043 | --- | 1687.82 | 17.39 |
Sodium | 89.30 | --- | 27.81 | 68.86 |
Calcium | 9.42 | 40-100 | 5.09 | 45.97 |
Magnesium | 20.20 | 10-15 | 0.87 | 95.7 |
Residual chlorine | 1.3 | --- | 0 | 100 |
Oil and grease | 26.8 | --- | 0 | 100 |
Denim wastewater treatment
The denim wastewater experiments were carried out using the same ferrate doses as those used for the aqueous solution. The maximum color removal was achieved during the first hour. The initial pH of the wastewater was 6.3. According to Ciabatti et al. (2010), the optimum pH range for the ferrate treatment of textile wastewater is 6.5-8.5, because the pH influences the molecular structure of dyes; that is, the dyes become protonated in acidic solution, which would induce the degradation process (Han et al. 2013). When the pH is higher, the oxidizing power of ferrate (VI) is lower, but the oxidation of water is much slower, which favors the oxidation of chemical species such as organic matter in the wastewater (Ciabatti et al. 2010).
As shown in figure 6b and 6d, the optimum dose was 200 mg/L K2FeO4, at which 92.51 % color removal efficiency was reached in 30 min. When the same dose of Na2FeO4 was added, the efficiency was only 72.85 %. Few works have reported the application of ferrate to real wastewater. Ciabati et al. (2010) treated textile wastewater using 70 mg/L K2FeO4, and achieved removal efficiencies of 62 % for COD, 70 % for TSS, and 85 % for turbidity. Gombos et al. (2013) applied 2-15 mg/L Na2FeO4 to a municipal secondary effluent and observed that the organic matter reduction was a function of ferrate concentration; efficiency removals of 15-60 % of COD and 2-31 % of TOC were obtained. Since ferrate ions will be reduced to Fe(III) ions or Fe(OH)3 during the oxidation process, Na2FeO4 and K2FeO4 can act as coagulants. When the ferrate salts are dissolved in water, oxygen is evolved and ferric hydroxide precipitates from the solution; this makes the ferrate solution unstable (Eq. 9). Hence, this compound represents a unique dual function (oxidant and coagulant) chemical reagent that can be an effective alternative to current approaches for denim wastewater treatment.
K2FeO4 could be regenerated in water by the reaction of Fe(OH)3 with KOH and residual chlorine, as shown in equation 11. In the denim wastewater, 1.3 mg/L of residual chlorine was detected. For this reason, K2FeO4 was more efficient than Na2FeO4.
In addition, the stability of ferrate in the effluent is significantly influenced by the initial ferrate concentration, pollutant concentration, pH, temperature, and the concentrations of aqueous constituents, such as chloride, sulfate ions (SO4 2-), carbonate, and NO3 - (Schroyer and Ockerman 1951, Sharma et al. 2010). The stability of ferrate solution increases with the presence of KCl, KNO3, PO4 3-, alkalinity, Cl-, SO4 2-, and/or high pH values (Wagner et al. 1952). This could explain the differences in the treatment time required for the aqueous solution (26 h) and the denim wastewater (1 h).
According to the results shown in figure 6d, K2FeO4 showed considerately improved color removal efficiency compared to Na2FeO4, due the difference in solubility. The potassium ion has an ionic radius of 1.33 Å, while that of the sodium ion is 1 Å. As the cation size increases in a compound with the same anion, the solubility decreases. Thus, the concentration of K2FeO4 was lower but its chemical activity and reaction capacity were higher; Na2FeO4 had a higher concentration and solubility but lower chemical activity in the solution.
The removal efficiencies achieved are shown in table III. An increase in the BOD was observed, which in turn caused an increase in the biodegradability index (0.21 to 0.98). This confirms that the biorefractory compounds were oxidized/removed, and that the treatment could prevent damage to the aquatic environment.
Finally, after treatment by K2FeO4, UV-Vis spectra of the wastewater before and after 30 min of treatment were obtained in order to verify the removal of the dye from denim wastewater using the peak 661 nm. The results shown in figure 8 confirmed that the maximum removal was achieved using 200 mg/L of K2FeO4. This coincided with the previously obtained results.
The sludge obtained after treatment with K2FeO4 was analyzed by infrared spectroscopy, and its spectrum was compared with that of the untreated denim wastewater. As shown in figure 9 and table V, different bands associated with different functional groups were observed in the two spectra. Analysis of these bands indicated that some of the aromatic organic compounds present in the wastewater were oxidized to aliphatic compounds.
TABLE V ASSOCIATED FUNCTIONAL GROUP ACCORDING TO INFRARED SPECTRA
Sludge | Denim wastewater | ||
Wavelength (cm-1) | Functional group | Wavelength (cm-1) | Functional group |
3325-3278 | N-H | 2916 | C aromatic |
2920,1369, 1311 | C aromatic | 2584 | C-H aliphatic |
2846-2353 | C-H aliphatic | 1843 | C=O |
1712 | C=O | 1616 | C-C |
1624 | C=C | 1384 | Fe-O |
1319, 1242, 1157 | C-N | 1365 | Fe-O |
1099 | C-CO-C | 1002, 972 | C-H aliphatic |
875, 794, 756 | N-H, C aromatic | 829, 702 | C aromatic |
694, 644 | Organochlorine compounds |
The signal of the N-H group disappears after the treatment, as well as some of the bands corresponding to aromatic groups, confirming the partial oxidation of the samples. The denim wastewater contained organochlorine compounds that were partially oxidized by the treatment; the corresponding signal disappeared from the spectrum. The indigo dye in the wastewater was oxidized to various degradation byproducts (isatin, carboxylic acids, carbon dioxide, and ammonia). These results allow a possible mechanism to be determined, as shown in figure 10. The iron sludge (hydroxide and oxyhydroxides) formed during the treatment could remove aromatic compounds and promote the coagulation process.
According to the chemical species diagrams (Medusa program), SO4 2- and PO4 3- possibily formed several complexes, solid compounds and species in solution: Ca5(PO4)3OH(s), MgFe2O4(cr), KSO4 -, and MgPO4 -.
CONCLUSIONS
Ferrate (VI) was found to be a good oxidant to remove dye from aqueous solution and a textile wastewater sample, and the treated water exhibited good physical and chemical qualities. The optimal operational conditions for the electrosynthesis of ferrate were determined to be room temperature (25 ºC), a current density of 100 mA/cm2 and NaOH 20 M, yielding 6.89 g/L of ferrate. Sodium and potassium ferrate were synthesized at room temperature and showed high stability due to the positive effect of the BDD cathode on the production of ferrate.
The maximum dye removal percentages were achieved using sodium ferrate at a dose of 300 mg/L for all the dye concentrations evaluated (10-100 mg/L of indigo blue dye), while efficiencies of 94 and 96 % were obtained for dye concentrations of 20 and 30 mg/L dye concentration, respectively, using a dose of 200 mg/L. On the other hand, potassium ferrate showed better color removal efficiency than sodium ferrate in denim wastewater. The optimum dose was 200 mg/L K2FeO4 at 30 min. When the same dose of Na2FeO4 was added, the color removal efficiency reached only 72.85 %. The treatment time for the textile wastewater was lower than that of the aqueous indigo blue solutions due to the presence of inorganic ions.
After the denim wastewater treatment, the biodegradability index of the wastewater showed a significant increase (0.21 to 0.98), which confirmed that the biorefractory compounds were oxidized/removed, and thus that the treatment could prevent damage to the aquatic environment.