Servicios Personalizados
Revista
Articulo
Indicadores
-
Citado por SciELO
-
Accesos
Links relacionados
-
Similares en SciELO
Compartir
Investigaciones geográficas
versión On-line ISSN 2448-7279versión impresa ISSN 0188-4611
Invest. Geog no.111 Ciudad de México ago. 2023 Epub 04-Mar-2024
https://doi.org/10.14350/rig.60696
Articles
Distribution and Characterization of the Mixteca Alta-UNESCO Geopark Dikes as Evidence of an Extensional Tectonic Regime in Western Oaxaca, Mexico
*Facultad de Química, Universidad Autónoma del Carmen (UNACAR), Ciudad del Carmen, Campeche, México. Email: ikarench12@gmail.com
**Instituto de Investigaciones en Ciencias de la Tierra, Universidad Michoacana de San Nicolás de Hidalgo, Santiago Tapia 403, 58000, Morelia, Michoacán, México.
***Facultad de Química, Universidad Autónoma del Carmen (UNACAR), Ciudad del Carmen, Campeche, México. Email: jsilis@pampano.unacar.mx
¶Departamento de Geografía. Facultad de Filosofía y Letras. Universidad Autónoma de Madrid (España). Calle Francisco Tomas y Valiente, 1. Campus de Cantoblanco. 28049. Madrid, España. Email: jalcalar@ghis.ucm.es
§Departamento de Arte y Ciencias del Territorio, Universidad de Extremadura-UEX, Av. de las Letras, s/n, 10003. Cáceres, España. Email: gonfermar@comunidad.unam.mx
‡Facultad de Química, Universidad Autónoma del Carmen (UNACAR), Ciudad del Carmen, Campeche, México. Email: apmarin@pampano.unacar.mx
∆Facultad de Química, Universidad Autónoma del Carmen (UNACAR), Ciudad del Carmen, Campeche. Tel: +52 1 938 105 3128; alejandromdov@gmail.com
The Mixteca Alta UNESCO Global Geopark (GMUMA) is in the western portion of the State of Oaxaca and stands out for having a variety of geological and geomorphological features, some of which represented by intrusive igneous bodies. Among them are the exposed dikes in the towns of Santo Domingo Tonaltepec, Santo Domingo Yanhuitlán, and San Pedro Añañé. These tabular bodies cut unconformably through the subsurface rocks and often develop as swarms of individual dikes with parallel, linear, or radial orientations under the influence of other tectonic events or geological structures.
In this research, the dikes present in the GMUMA were identified and mapped through the analysis of satellite images and taking into account field observations, where their presence was compared, and the associated geological-structural features were identified. Likewise, with the help of the Stereo32 1.0.1 program, a total of 66 directions of the dikes were graphed and rose diagrams were elaborated to visualize the preferential direction of the dikes and relate it to the tectonic regime of the region. Three groups of dikes were identified with longitudinal traces that vary from a few meters to 1 km and with approximate thicknesses of between 4 and 5 m, with a preferential direction towards the northwest. The dikes exhibit three types of morphologies: planar stepped and braided, whose interpretation and directions allowed us to establish that the dikes are associated with an extensive tectono-magmatic event that remained active during the Eocene, with a preferential direction towards the northwest-southeast, like the orientation of the Tamazulapan and Cieneguilla faults.
Keywords: Mixteca Alta UNESCO Global Geopark; dikes; Tamazulapan fault; Cieneguilla fault
El Geoparque Mundial UNESCO Mixteca Alta (GMUMA), localizado en la porción occidente del estado de Oaxaca, al sur de México, se destaca por una variedad de rasgos geológicos y geomorfológicos, algunos representados por cuerpos ígneos intrusivos. Entre ellos están los diques expuestos en las localidades de Santo Domingo Tonaltepec, Santo Domingo Yanhuitlán y San Pedro Añañé. Estos cuerpos tabulares cortan discordantemente las rocas del subsuelo, y suelen desarrollarse en enjambres de diques individuales con orientaciones paralelas, lineares o radiales bajo la influencia de otros eventos tectónicos o estructura geológicas.
En este trabajo se identifican y cartografían los diques del GMUMA a través del análisis de imágenes satelitales, complementado con observaciones en campo donde se cotejó su presencia y se identificaron los rasgos geológico-estructurales asociados. Así mismo, con ayuda del programa Stereo32 1.0.1, se graficaron un total de 66 rumbos de los diques y se elaboraron diagramas de rosas para visualizar la dirección preferencial de los diques y relacionarlo con el régimen tectónico de la región.
Se identificaron 3 grupos de diques con trazas longitudinales que varían desde unos pocos metros hasta 1 km y con grosores aproximados de entre 4 y 5 m, con una dirección preferencial hacia el noroeste. Los diques exhiben tres tipos de morfologías: planares, escalonados y trenzados, cuyas interpretaciones y direcciones permitieron establecer que los diques están asociados a un evento tectono-magmático de tipo extensivo que se mantuvo activo durante el Eoceno, con una dirección preferencial hacia el noroeste-sureste, similar a la orientación de las fallas Tamazulapan y Cieneguilla.
Palabras clave: Geoparque Mundial UNESCO Mixteca Alta; diques; Falla Tamazulapan; Falla Cieneguilla
INTRODUCTION
A dike is defined as a tabular intrusive body of 1 m to 10 m thick that cuts unconformably across the stratification or foliation of subsurface rocks (Acocella and Neri, 2003), usually t a high angle (≥ 45°) and with an extension or lateral spread spanning from a few meters to hundreds of kilometers (Hoek, 1991). Dikes are the result of a flow of pressurized magma that moves mainly through existing fractures or new cracks that originate as magma makes its way through the adjacent rock to finally solidify (Daniels et al., 2012). Dikes usually develop in geological contexts related to a) shield volcanoes on volcanic islands linked to hotspot activity and away from plate boundaries that impose a regional stress field; b) stratovolcanoes with local and regional tectonic stress controls; and c) shield volcanoes located along the oceanic ridges that impose strong regional control over the sites of the dikes (Acocella and Neri, 2009). They also occur as fillings of pure extension fractures (Delaney et al., 1986) and can form unique geometric arrangements, either in groups or swarms of multiple individual dikes with parallel, linear, or radial orientations between them. Normally, dikes are associated with a single geological event, and those belonging to the same swarm usually have a similar chemical composition (Janosy, 1994). The propagation of dikes requires that magma exerts enough pressure on the displacement tip to overcome the resistance of rock fractures, allowing the dike to grow as magma flows toward the tip. Therefore, the lateral propagation of dikes will depend on the source, pressure, and volume of magma (Gonnermann and Taisne, 2015).
The propagation of dikes is determined by the main stress planes in the host rock at local and regional levels, as they tend to form perpendicularly to the minimum stress axis (σ3) and parallel to the maximum stress axis (σ1), as described by Geshi and Oikawa (2016). This means that the regional stress regime at the time of the dike emplacement can be reconstructed from the behavior of the dike itself (Delaney et al., 1986; Janosy, 1994; Nakamura, 1977). Furthermore, dike orientation and morphology also reflect the emplacement process where magma composition, temperature, and pressure as well as the stress distribution the physical characteristics and strength of the encasing rock, play a key role in the rise of magma (Daniels et al., 2012). Acocella and Neri (2009) also suggested that, in addition to being controlled by the orientation of the main stresses in the crust, dikes propagate according to the presence of any topographic relief, the shape of the volcanic edifice, and the regional tectonic control. The fluctuations of the factors mentioned above gives rise to three different types or configurations of dikes: regional, circumferential, and radial (Hoek, 1991; Acocella and Neri, 2009). For instance, regional dikes usually occur as elongated swarms, most often parallel to each other, and where a distant (i.e., regional) stress field controls their emplacements (Llambias and Sato, 1990). In general, regional dikes occur as pure extension or traction fractures (i.e., Griffith cracks), although they can also be formed in existing faults or fractures. Also, regional dikes propagate in the oceanic crust, where their emplacement mechanism is related to the divergence of plates, although they are also common in continental areas, where they often extend from a few meters to hundreds of kilometers (Acocella and Neri, 2009; Gudmundsson and Marinoni, 1999). At the boundaries of the divergent plates, in continental or oceanic areas, extensional stress concentrates the strain on the rift axis where volcanoes are usually found (Acocella and Neri, 2009). In short, the spread of regional dikes is controlled by regional tectonism, where they propagate as magma-filled fractures in a principal stress plane (parallel to σ1 and perpendicular to σ3) (Gudmundsson and Marinoni, 1999). In the absence of a natural terrain elevation (e.g., mountains and hills), dikes propagate parallel to the orientation of the main stress field.
On the other hand, swarms of circumferential dikes occur as agglomerations with a primary geometry almost -- but not entirely -- circular or elliptical arrangement (Buchan and Ernst, 2018, 2019) or with concentric arched patterns (Acocella and Neri, 2009; Buchan and Ernst, 2018, 2019). They can be associated with various tectonic environments, like surrounding the edges of volcanic calderas or in the centers of igneous complexes in large igneous provinces (e.g., Chadwick and Dieterich, 1995; Mäkitie et al., 2014). It is worth mentioning that circumferential dikes, like regional dikes, are generally related to the absence of a significant topographic relief that affects their stress field. However, unlike regional dikes, circumferential dikes are not controlled by regional stress or tectonics. In the absence of such a regional stress field, the pattern, location, and propagation of a dike can be controlled by topographic irregularities, as is the case of shallow calderas or reservoirs (Acocella and Neri, 2009).
Radial dikes are commonly observed around stratovolcanoes, because the pressure of the magma chamber produces stress in the surrounding regions, whereas the load imposed by the edifice controls the main stresses, orienting them radially (σ1) and tangentially (σ3) (Hyndman and Alt, 1987) around the volcano (e.g., Chadwick and Howard, 1991; Acocella and Neri, 2003; Acocella et al., 2006; Muller and Pollard, 1977; Poland et al., 2008).
In this study, we analyze the distribution and characteristics (shape and emplacement orientation) of the dikes that emerge within the area of the Mixteca Alta UNESCO Global Geopark (GMUMA, for its acronym in Spanish), located in the western portion of the State of Oaxaca, Mexico (Figure 1b). The GMUMA covers an area of approximately 415 km2, limited by coordinates 17° 38' 14"-17° 28' North and 97° 27' 40"-97° 28' 10" West, so it covers part of the topographic charts of Tamazulapam E14- D25, Coixtlahuaca E14-D26 E14, Santiago Yolomecatl E14 D36-D35, and Asuncion Nochixtlan (Figure 1c). The formation of dikes and other igneous bodies present in the GMUMA has been broadly discussed (e.g., Ferrusquia-Villafranca, 1976; Martiny et al., 2000; Palacio et al. 2016; Santa Maria, 2009a), but the cartographic record of the distribution of dikes is still incomplete. It is also unknown how the orientation and morphology of these intrusive magmatic structures are related to the tectonic evolution of the region. Therefore, the present work aims to establish such relationship, using data from a geospatial analysis and field observations. This approach allowed us to define the type of configuration of dikes in the study area, their primary geometric pattern, their relationship with the nearby main tectonic structures and their implication on magmatic evolution. We expect that the result will contribute to improve the knowledge of the tectonic and magmatic evolution of the southern region of Mexico, increasing the geological interest in the region and the GMUMA. To this end, using data from a geospatial analysis and field observations, we defined the type of configuration of dikes in the study area, their primary geometric pattern, and their relationship with the main tectonic structures in the area and their implication on magmatic evolution.
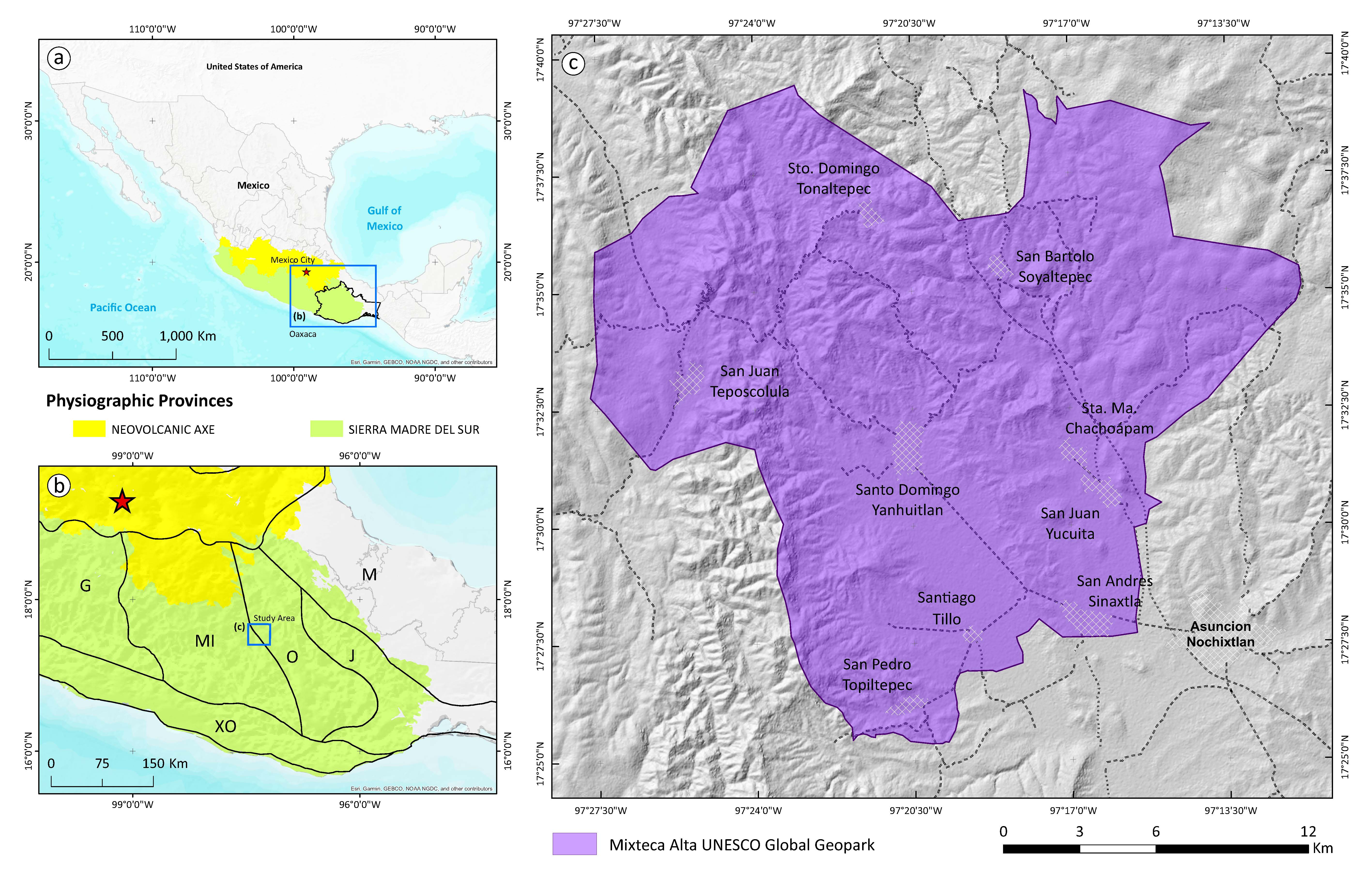
Figure 1 Location of the study area: a) Distribution of the Trans-Mexican Volcanic Belt (FVTM, for its acronym in Spanish) and the Sierra Madre del Sur Physiographic Province; b) distributions of the tectonostratigraphic terrains of Sierra Madre del Sur (modified from Morán-Zenteno et al., 2018). MI: Mixteco; O: Oaxaca; J: Juárez; M: Maya; XO: Xolapa; G: Guerrero; PM: Morelos Platform (proposed by Ortega-Gutiérrez et al., 2012); c) polygon of the Mixteca Alta UNESCO Global GMUMA.
GEOLOGICAL CONTEXT
The GMUMA is located in the Sierra Madre del Sur (SMS) physiographic province, which spans from the western portion of the States of Jalisco and Colima, in the north, to the eastern region of the State of Oaxaca, in the south, through the States of Michoacan, Guerrero, and Mexico (Figure 1a). It is limited by the Trans-Mexican Volcanic Belt (TMVB) to the north. The geology and stratigraphy of the study area, summarized in Figures 2 and 3, respectively, indicate that the oldest rocks correspond to two Mesozoic rock units (Montes de Oca, 2021). The first is the light gray Teposcolula Formation (Ortega-González, 1991), whose textures range from mudstone to packstone with flintstone nodules, and also containing benthic foraminifera, rudist bivalves, miliolids, and ostracods. The second is the Yucunama Formation, which has an approximate thickness of 300 m to 450 m (Ferrusquia-Villafranca, 1976) and is composed of nodular marls interspersed with sandstones, shales, and a conglomeratic horizon with subrounded calcareous clasts at the top (Castillo-Reynoso, 2018). Both Mesozoic rock units are successively overlaid by i) the Tamazulapam Formation (conglomerate with subrounded clasts ranging from 5 mm to 25 cm in size, of calcareous composition -- mainly limestone, marl, flintstone, and sandstone -- presenting a reddish sandy matrix and with thicknesses ranging from 20 m to 200 m) (Santamaría-Díaz, 2009a); ii) the Yanhuitlán Formation (a succession of 160 m to 600 m of thin semi-consolidated clay tosilty layers with red to yellow shades) (Castillo-Reynoso, 2018); iii) The Teotongo Deposits (volcano-sedimentary units formed by sandy epiclastic deposits sedimented in a lagoon environment and pyroclastic deposits, mainly lithic tuffs of intermediate composition) (Santamaría-Díaz, 2009b; Castillo-Reynoso, 2018); and (iv) the Llano de Lobos Tuff pyroepiclastic sequence, with a thickness of 300 m to 350 m (Ferrusquía-Villafranca, 1976). Several parts of these sequences of rocks are covered by a series of andesitic lava of the Oligocene with a thickness of 500 m to 1000 m which is classified as Yucudaac Andesite (Ferrusquía-Villafranca 1970, 1976) or Undifferentiated Andesite (Castillo-Reynoso, 2018). Additionally, there are other intrusive bodies, known as Suchixtlahuaca Hypabyssal Intrusions (Figure 2) formed by laccoliths, stocks, sills, and dykes of andesitic composition, whose ages vary between 45.5 ± 1.7 Ma and 20.0 ± 0.9 Ma (Ferrusquía-Villafranca, 1976; Martiny et al., 2000; Santamaría-Díaz, 2009b).
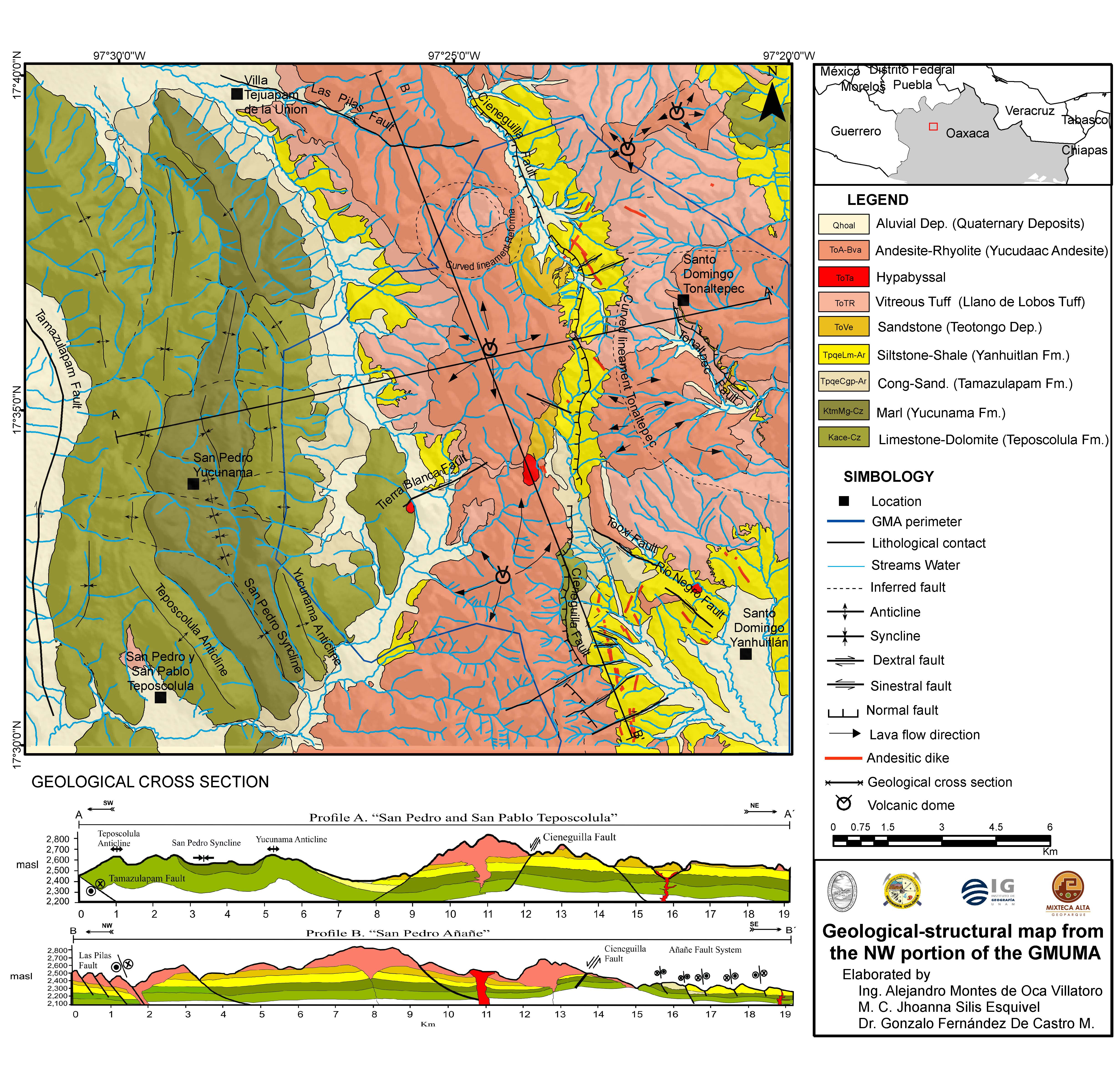
Updated from Montes de Oca (2021).
Figure 2 Geological-structural map of the NW portion of the GMUMA.
Tectonically, the Sierra Madre del Sur is considered one of the territories with the greatest structural-geological complexity in the American-Pacific continental border (Hernández-Santana et al., 1995). In fact, in the Oaxaca region, intense geological events that occurred since the Precambrian to the Present have been recorded among the most important that have affected southern Mexico. Several authors have proposed various models based on field observations, data from key areas in the continental crust, and inferences about the kinematics of the adjacent oceanic plates to describe the geological evolution of this territory (Keppie and Morán-Zenteno, 2005; Morán-Zenteno et al., 2007; Rogers et al., 2007; Pindell and Kennan, 2009; Ferrari et al., 2014; Morán-Zenteno et al., 2018). However, no model is currently available that fully explains the tectonic evolution of the region without raising questions related to its approach. Morán-Zenteno et al. (1999, 2000) indicated that the distribution of magmatic rocks in the SMS and the variations in the composition, stratigraphy, and apparent relationships with the tectonic environment of rocks correspond to active geodynamics characterized by the interactions of the North American, Caribbean, and Farallon Plates and the subsequent displacement of the Chortis Block. Martiny et al. (2000) revealed that the plutonic and volcanic rocks located in southern Mexico correspond to Paleocene-Miocene magmatism, which occurred at a time characterized by several tectonic events related to interactions of tectonic plates as a result of detachment and lateral displacement to the southwest of the Chortis Block, and with the emergence and evolution of the Caribbean Plate (Martiny et al., 2000; Morán-Zenteno et al., 1999). Other tectonic events that have affected the region include the amalgamation and rupture of Pangaea, the opening of the Gulf of Mexico, the Laramide Orogeny, the subduction of the Farallon Plate, and, currently, the subduction of the Cocos Plate below the North American Plate (Keppie and Morán-Zenteno, 2005; Morán-Zenteno et al., 2007; Rogers et al., 2007; Santamaría-Díaz, 2008; Pindell and Kennan, 2009; Ferrari et al., 2014; Morán-Zenteno et al., 2018). However, the role played by this redistribution of tectonic plates in determining the magmatism patterns in the region is still unknown (Morán-Zenteno et al., 1999).
At local scale, the tectonics of the study area can be summarized with the presence of several structures such as the Teposcolula Anticlinal, the Caltepec, Las Pilas, and Oaxaca Faults, and, particularly, the Tamazulapam and Cieneguilla Faults that are located closer to the GMUMA (Figure 2) (e.g., Lopez-Ticha, 1985; Centeno-García, 1988; Elías-Herrera et al., 2005; Nieto-Samaniego et al., 2006; Santamaría-Díaz et al., 2008). The Caltepec Fault has been described as an Early Permian dextral transpressional fault with N-S and NNW direction with a record of tectonic-magmatic-metamorphic events during the Permian, and multiple Mesozoic, Cenozoic, and probably even recent reactivation periods (Elías-Herrera et al., 2005). Elías-Herrera and Ortega-Gutiérrez (2002) interpreted the Caltepec Fault Zone as an important boundary that marks the juxtaposition of the Acatlán and Oaxacan Blocks that relates the final assembly of the western central Pangaea (clash of Gondwana against Laurentia in an oblique convergent environment in the Early Permian era). Santamaría-Díaz (2008) interpreted the continuation of the Caltepec Fault south of Tejupan as the Cieneguilla Fault and, instead, he relocated Caltepec between the Tamazulapam and Cieneguilla Faults as a normal and right lateral fault zone about 10 km wide, represented by a broad heterogeneous deformation zone and not by a direct trace. The Tamazulapam Fault, on the other hand, has two displacement orientations: a normal fault and a right lateral fault, with an important vertical component located to the west of the Tamazulapam and Teposcolula Anticlines. This fault brings into contact Cretaceous rocks with Paleocene-Eocene rocks from the south of the town of Tamazulapam to Santa Maria Yolotepec (Santamaría-Díaz et al., 2008; Ferrusquía-Villafranca et al., 2016). It was proposed for the first time by López-Ticha (1985) to describe a larger structure related to an ancient consolidation of land with its own basements and geological history (Elías-Herrera et al., 2005). It has an approximate N-S orientation with an average dip between 60º to 80º to the SW and NE and a trace variation of 20° following the Tamazulapam Anticline. It presents two curves in which the strike-slip displacement formed a transpressional deformation zone to the north and a transtensional deformation zone to the south, related to two regional tectonic events that affected the Tamazulapam Fault: (i) a transpressional event to the NE-SW which was active between the Late Eocene and the Early Oligocene, affecting the Chilapa, Yanhuitlan, Tamazulapam, Teposcolula, and Tecocoyunca formations, and (ii) a transtensional event, at the end of the Oligocene, with a maximum NE-SW extension orientation (Santamaría-Díaz et al., 2008; Ferrusquía-Villafranca et al., 2016). Traces of the Cieneguilla Fault are located about 15 km east of the Tamazulapam Fault; Cieneguilla is described by Santamaría-Díaz et al. (2008) as a normal fault with a dip to the west and a continuous trace of 22 km, a width of 8 m, and spreading to the north for another 32 km as small fault segments. These authors proposed that the Cieneguilla Fault is situated south of Tejupan, where the continuation of the Caltepec Fault had previously been located (Elías-Herrera et al., 2005). It cuts across the Llano de Lobos Tuff and the Teotongo Volcaniclastics to the north and the Yanhuitlán Formation to the south, binding it with the Yucudaac Andesite. Both, the Cieneguilla and the Tamazulapam Faults were activated during an extensional tectonic event between the Late Eocene and the Oligocene (Santamaría-Díaz et al. 2008).
METHODOLOGY
Dike Identification
In this study, we conducted the structural geological mapping of the Mixteca Alta UNESCO Global Geopark at a 1:50 000 scale, covering the northwest of the Asunción Nochixtlán municipality (Figure 1c). The identification and mapping of the GMUMA dikes were mainly based on the analysis of satellite images through Google Earth supplemented with information collected during fieldwork. It should be noted that the geological characteristics of the study area helped and facilitated the identification from Google Earth. For instance, since most dikes in the study cut through the Yanhuitlán Formation, there is a marked lithological contrast between both rock types (sedimentary for the Yanhuitlán Formation and igneous for the dikes). Besides, the highly advanced differential erosion process in the region (Palacio-Prieto et al., 2016; 2019) allowed that the dikes emerge with a slight slope over the Yanhuitlán Formation. Thus, as observed in Figure 4, the natural color contrast between both lithologies (reddish Yanhuitlán Formation versus dark dikes), as well as the morphology (longitudinal trace and relatively reduced thickness) of dikes make the latter easily recognizable on Google Earth images.

Figure 4 Google Earth and field image that shows the contrasts of color and competence between the dikes and the Yanuitlan Formation. Such contrast facilitated the detection of dikes in Google Earth.
Then, the preliminary map was verified during two fieldworks to confirm that the structures identified by means of satellite images corresponded to dikes. These fieldworks also served to compile information of lithology, degree of alteration, dike dimensions and geometry, as well as faults and diaclases when they were found. All the information were useful for interpreting and understanding the tectonic and magmatic evolution in the region.
Distribution, Configuration, and Structural Analysis of the Dikes
To determine the relationship and type of configuration of dikes in the GMUMA, we considered the presence of any topographic relief that could impose a local gravitational stress field or condition the geometric pattern resulting from the dikes, as well as the tectonic configuration of the region. Then, a digital elevation model (DEM) was created, derived from InSAR data (obtained from the Alaska database), to visualize with detail the topography. . Furthermore, the vector data of the dikes identified in the GMUMA were included to analyze their spatial distribution. In the same way, outstanding structural features were recognized, such as regional faults, folds, or diaclases that could serve as a reference to determine the orientation of past and current tectonic stresses or any potential relationship between the alignment of major tectonic structures and those of dikes.
The orientation of the mapped dikes was measured using a transporter in Google Earth (Table 1). Subsequently, these orientation data were imported into the software Stereo32 1.0.1 to obtain the rose diagrams of fractures and define their preferential courses and configuration with better detail. Following this sequence, 66 structural data of the dikes were analyzed and their orientations compared to determine how they interrelate.
Table 1 Courses and azimuths of magmatic dikes
No. | Gruop 1 | Gruop 2 | Gruop 3 |
1 | 54° | 27° | 325° |
2 | 22° | 22° | 319° |
3 | 326° | 332° | 326° |
4 | 25° | 322° | 316° |
5 | 32° | 302° | 323° |
6 | 25° | 8° | 327° |
7 | 315° | 328° | 325° |
8 | 321° | 350° | |
9 | 329° | 8° | |
10 | 333° | 347° | |
11 | 335° | 331° | |
12 | 332° | 338° | |
13 | 335° | 351° | |
14 | 330° | 353° | |
15 | 337° | 356° | |
16 | 330° | 353° | |
17 | 329° | 350° | |
18 | 316° | 350° | |
19 | 327° | 350° | |
20 | 325° | 347° | |
21 | 296° | 331° | |
22 | 341° | 334° | |
23 | 10° | ||
24 | 18° | ||
25 | 15° | ||
26 | 357° | ||
27 | 353° | ||
28 | 336° | ||
29 | 338° | ||
30 | 355° | ||
31 | 15° | ||
32 | 3° | ||
33 | 0° | ||
34 | 7° | ||
35 | 356° | ||
36 | 358° | ||
37 | 343° |
In addition, we reviewed the topographic maps edited by the INEGI of Tamazulapam E14-D25, Coixtlahuaca E14-D26, Santiago Yolomécatl E14-D35, and Asunción Nochixtlán E14-D36, all at a 1:50 000 scale, to generate a base map at a 1:50 000 scale showing the topographic, hydrographic, and regional hypsometric context of the GMUMA area. In this way, a preliminary cartographic base was obtained on which the distribution of dikes was highlighted for subsequent analysis and interpretation, aiming to determine which tectonic regime controlled the geometric pattern of the swarm and whether this regime was local or regional. We also considered the geometric characteristics of each dike, such as size, shape, and potential systematic interactions with adjacent dikes, to evaluate the evolution of fractures in the geological context.
RESULTS
Spatial Distribution of Dikes
The analysis of Google Earth images for the entire GMUMA revealed that dikes emerge only along an N-S corridor in the western zone of the park. The dikes tend to form three main groups according to the relative distance between them (Figure 5a).

Figure 5 a) Map of the study area showing the main geological structures in the region (Tamazulapan and Cieneguilla Faults) and the distribution of dikes in the GMUMA according to three groups (see Tables 1, 2, and 3). The location of the andesitic laccolith analyzed by Martiny et al. (2000) is shown. b) Rose diagrams representing the main orientation of each group of dikes in the region.
The first group is located in the northern portion of the study area northwest of Santo Domingo Tonaltepec municipality. It comprises 22 dikes showing a preferential orientation to the NNW, although some are oriented to the NE (Figure 5a).
The second group is located in the south-center, near the San Pedro Anane and Santo Domingo Yanhuitlán municipalities (Figure 5a), where 32 dikes of different lengths and thicknesses are distinguished. Ninety-five percent of these dikes are located west of the San Pedro Añañe municipality and are distributed along a strip approximately 4 km long by 2 km wide with an N-S orientation. They also have an NNW direction, with 3 m to 7 m thicknesses, although some deviate from this trend toward the NNE (Figure 5a).
A third group of 5 dikes is located further south, distributed in parallel with a trend toward the NW (Figure 5a).
The representative rose diagram of all faults (Figure 5a) indicates that preferential orientations of 66 dikes have a dominant population between N10°W and N40°W, although this is not always the case. For example, there are places within the swarm where dikes have an oblique direction not exceeding N35°W. The group of dikes located in the northern sector of the study area (group 1 or quadrant 1 in Figure 5a), as well as the dikes of group 3, are characterized by preferential strikes between N20°W and N40°W and another minor variation between N20°E and N30°E (Figure 5b). By contrast, the second group located in the south-center shows strikes between 0° and N30°W, with small deviations between 0° and N20°E (Figure 5b).
A distinctive characteristic of all dikes is that the distribution between the arrangement of fractures is propagated across the plane without arching or changing direction, following a systematic trend where compensations between dikes are common or they maintain a parallel and semi-parallel trend with a more or less constant separation between them (Figure 5a). On the other hand, we observed scarce geometric links between segments - areas of physical intersection between two or more fracture surfaces, for example, between adjoining or crossed fractures, or bifurcations in anastomosed fractures (Peacock et al., 2016). It is noteworthy that the distribution of the dikes shows similar directions to the drainage network of the study area (Figure 6). Some dikes, mainly those of groups 1 and 3, show an orientation similar to that of intermittent currents, while the dikes near San Pedro Añañe have an alignment similar to the limit of the subbasin. The analysis of the hypsometric map (Figure 6) indicates that the dikes coincide with the mountainous relief of the study area. For example, the second group of dikes is distributed at the foothills of the Yanhuitlán Valley, a gently sloping valley with multiple irregularities located in the southeast portion of the study area, where the Sacagua and Negro rivers flow across the bottom (Figure 6). Its main direction is NNW, relatively parallel to the plains, valleys, and foothills that are predominantly aligned toward the NW, in parallel with the higher zones that, in turn, are aligned with the mid-altitude mountains located at the center of the study area. These mid-altitude mountains show an NNW-SSE alignment corresponding to the highest-slope line (watershed or borderline).
General Characteristics of Dikes
The main characteristics of the dikes of the GMUMA were identified based on satellite images and field observations. The dikes generally have longitudinal traces ranging from a few meters to 1 km and measuring between 4 m and 5 m in thickness. They show evidence of fragile deformation (fractures and faults) and different degrees of weathering and erosion. For example, Figure 7 illustrates prototypes of dikes affected by erosion at the top (Figure 7a-b).Another feature observed in some dikes is a metamorphic aureole of variable thickness, generally not beyond 50 cm, which spreads toward the encasing rock (Figure 7b and c). Besides, the dike walls display a wide variety of textures and geometries. Some dikes show an arrangement of curviplanar diaclases that has led to the development of an “onion skin” alteration or spheroidal weathering, a process in which rounded rock cores (corestone) are surrounded by concentric layers called “shells” (Ollier, 1971). In these cases, the node edges are rounded, adopting a spheroidal shape, and, in turn, fragments are surrounded by approximately concentric layers of rock of relatively uniform thickness, which exhibit nodules measuring 30 cm to 40 cm inside the dike’s main body (Figure 7D).

Figure 7 Particularities of some dikes located in the Mixteca Alta-UNESCO Global GMUMA. The top of the dikes in a), b), and c) has been severely affected by weathering and erosion. Some dikes, such as b) and c), show a metamorphism aureole (delimited by red lines), while d) shows a dike with spheroidal weathering, and e) shows the surfacing of some GMUMA’s relatively “healthy” dikes.
Spheroidal weathering is common across the study area, not only within the dikes but also in other magmatic bodies, such as andesitic outcrops corresponding to the tourist geosites #5 and #33 of the GMUMA, located northeast of San Juan Teposcolula and south of Santo Domingo Tonaltepec, respectively.
However, there are several less altered dikes with massive textures, dense bodies, and scarce vesicles (Figure 7e). These dikes show diaclases in a grid arrangement (Figure 7e). Elsewhere, when the dike is slightly more altered, diaclases are better defined, and the dike appears as lava blocks (Figures 7b and c).
The morpho-structural analysis reveals that the dikes of the study area generally emerge with a planar morphology, that is, with simple, parallel, and tabular walls (Figure 8a, b, d), although they may be non-planar in some localities. In fact, according to Hoek (1991), the pattern of non-planar dikes that prevails in the Geopark has sinuous walls (Figure 8c), while others show stepped (Figure 8a, b) and braided (Figure 8e) segments. The dikes with sinuous walls (Figure 8c) are located mainly in dike groups 2 and 3, near San Pedro Añañe, characterized by a curvilinear appearance on their walls and equidistant from one another. On the other hand, the dikes with stepped geometry (Figure 8a,b) have jumps (distance between the steps perpendicular to their walls) of about 2 m. Some segments are connected by bridges (Figure 8a) of the same magmatic material. This type of geometry occurs in the three groups of dikes, although it is more frequent in the northern part of the study area. In fact, the longest dike identified within the GMUMA has several stepped segments., By last, braided dikes (Figure 8e) are characterized by the bifurcation and branching of 3 to 4 sinuous dikes. The most representative dike of this type identified in the study area is located south of San Pedro Añañe.

Figure 8 Morphology of the dikes of the Mixteca Alta-UNESCO Global GMUMA cutting across the Yanhuitlán Formation: a) stepped dike with a bridge linking two straight segments; b) stepped dikes with segments separated by jumps; c) sinuous dike; d) planar or straight dike; e) system of anastomosed or braided dikes. G1 and G2 refer to Group 1 and Group 2 dikes.
DISCUSSION
Most of the dikes studied in the GMUMA are distributed along a strip approximately 4 km long with an N-S direction and with a semi-parallel-to-parallel arrangement with each other. Although these dikes are discontinuous between them, they are similar in terms of orientation, thickness, and geometry within each of the three groups identified in this study (Figure 5). The general distribution is typical of regional dikes, and, according to the parameters described by Acocella and Neri (2009), the dikes of the GMUMA share similar geometric characteristics to dikes developed under an extensional regime. Such type of tectonic regime produces a divergence between blocks that usually give rise to dikes or generate the reactivation and development of faults and fractures along with fissures parallel to the regional stress field (Hjartardottir et al., 2012). In fact, as outlined previously in the section addressing the geological context, earlier studies have shown that plutonic and volcanic rocks of southern Mexico are related to several tectonic events connected with interactions of Tectonic Plates as a result of the detachment and lateral southwest displacement of the Chortis Block, and the emergence and evolution of the Caribbean Plate (Martiny et al., 2000; Morán-Zenteno et al., 1999). In the northwest of the State of Oaxaca, where our study area is located, the Cenozoic is characterized by transtensional tectonics that produced several faults with a general N-S direction (Morán-Zenteno et al., 2000), such as the Tamazulapam and Cieneguilla Faults, with which the traces of the studied dikes share a similar direction (Figure 5). According to Santamaría et al. (2008), both faults were reactivated repeatedly during an extensional tectonic event between the Late Eocene and the Early Oligocene (35.9 Ma and 29 Ma), with transpressional and transtensional movements (Ferrari et al., 2014; Morán-Zenteno et al., 2018) partly controlled by the tectonic boundaries of the terrains and the contrasting mechanical competences between them (Morán-Zenteno et al., 2000). However, given that the Cieneguilla Fault is the regional structure closest to the dikes and since it shows a primary N-S direction along most of its range, the genesis of the fault is likely related to contemporary magmatism during which the studied dikes were formed by magma that filled the fractures created, mostly with a similar orientation.
On the other hand, the dendritic-to-parallel drainage in the region and the fact that the distribution of dikes displays similar directions to such drainage constitute a consistent evidence of extensional tectonic control in the region. Additionally, it was observed that most dikes in the GMUMA have stepped segments with jumps of ~2 m or joined by bridges of the same magmatic material (Figure 8a and b), while other segments are braided (Figure 8e). This type of lateral displacement of dike portions indicates a system of post- or syn magmatic tectonic stresses. Dikes with marked jumps confirm the transpressional or transtensional movements in the study area previously mentioned by Santamaría-Díaz et al. (2008). The appearance of stepped dikes also demonstrates that the tectonic movements occurred shortly after the dike emplacement (before they cooled down completely), suggesting a temporary change in the orientation of the main strain (Pollard et al., 1982). Besides, the presence of dikes with bridges filled with the same magmatic material suggest the contemporaneity of the magmatic process and the tectonic activity.
Despite the lack of data on the ages or geochemical composition of the different groups of dikes found in the study area, it is known that the age of volcanic rocks outcropping in the region of Huajuapan de Leon and Tlaxiaco is between 34.8 ± 1.4 Ma and 31.4 ± 0.8 Ma (Martiny et al., 2000). In contrast, the magmatic intrusions took place approximately between 40.5 ± 1.7 Ma (Martiny et al., 2000) and 43.0±1.2 (Cerca et al., 2007), after applying the K-Ar method to an andesitic laccolith located at the center of both groups of dikes (star in Figure 5a). As the laccolith, the studied dikes intruded the Yanhuitlán Formation which has an age of 49 ± 8 Ma (Ferrusquía-Villafranca, 1976), consistent with the principle of cross-cutting relationships. In addition, these ages correspond to the diachronic pattern of magmatism in the Sierra Madre del Sur, associated with the displacement of the Chortis Block described by Ferrari et al. (2014). Therefore, the similar orientation, geometry and age of the dikes - fractures compare to other structures suggest that the dikes may have been emplaced concurrently. Furthermore, the variation in the direction of some dikes could also confirm the influence of different magmatic events related to the different reactivation phases of the Tamazulapan and Cieneguilla faults. The latter hypothesis is plausible and may explain the various degrees and types of alterations observed in the dikes (Figure 7) as it is expected that rocks of the same composition and age in the same geological and climatic context, as is the case of the dikes studied, are altered in the same way.
CONCLUSIONS
The analysis of Google Earth satellite images and fieldwork reveals three major groups of dikes in the GMUMA, with longitudinal traces oriented mainly to the NNW, although some deviate to the NNE. The dikes are distributed along a strip approximately 4 km long with an N-S direction and display a semi-parallel-to-parallel arrangement with each other, typical of regional dike swarms. This distribution indicates that GMUMA dikes emerged during an extensional tectonic regime. The similarity in the direction of dike traces with those of the Cieneguilla and Tamazulapan faults, combined with the information published of tectonic reactivations of both faults, suggests that magmatism occurred contemporaneously with reactivations, during which the magma penetrated through fissures.
FINANCING
This work was funded by the UNAM-DGAPA-PAPITT PE113820 project called “Geological and Geomorphological Mapping at 1:50.000 Scale of the Mixteca Alta GMUMA, Oaxaca, as a Means for Teaching and Disseminating Geological and Geographical Sciences”.
ACKNOWLEDGMENTS
This work summarizes the research conducted by the first author to obtain her bachelor’s degree. All the authors appreciate theassistance and support during fieldwork provided by the Mixteca Alta authorities. . We also acknowledge the observations and suggestions of two anonymous reviewers that improved the quality of the final manuscript.
CONFLICTS OF INTEREST
All authors declare that there is no interest of any sort that could influence or bias the contributions of this work.
REFERENCES
Acocella, V., y Neri, M. (2009). Dike propagation in volcanic edifices: overview and possible developments. Tectonophysics,471(1-2), 67-77. [ Links ]
Acocella, V., Porreca, M., Neri, M., Mattei, M., y Funiciello, R. (2006). Fissure eruptions at Mount Vesuvius (Italy): insights on the shallow propagation of dikes at volcanoes. Geology,34(8), 673-676. [ Links ]
Acocella, V, Neri, M (2003) What makes flank eruptions? The 2001 Mount Etna eruption and its possible triggering mechanisms. Bulletin of Volcanology, 65, 517-529. https://doi.org/10.1007/s00445-003-0280-3. [ Links ]
Buchan, K. L., y Ernst, R. E. (2019). Giant circumferential dyke swarms: catalogue and characteristics. Dyke swarms of the world: A modern perspective, 1-44. [ Links ]
Buchan, K. L., y Ernst, R. E. (2018). A giant circumferential dyke swarm associated with the High Arctic Large Igneous Province (HALIP). Gondwana Research,58, 39-57. [ Links ]
Campa, M. F., y Coney, P. J. (1983). Tectono-stratigraphic terranes and mineral resource distributions in Mexico. Canadian Journal of Earth Sciences,20(6), 1040-1051. [ Links ]
Castillo-Reynoso, Ramírez, V. E., Rivera Olguin, E (2018). Tamazulapam E14-D25, Escala 1:50,000 Estado de Oaxaca. Servicio Geológico Mexicano. [ Links ]
Centeno-García, E. (1988). Evolución estructural de la falla de Oaxaca durante el Cenozoico: México, DF, Universidad Nacional Autónoma de México, Facultad de Ciencias. Tesis de maestría. Facultad de Ciencias, UNAM. [ Links ]
Cerca, M., Ferrari, L., López‐Martínez, M., Martiny, B., y Iriondo, A. (2007). Late Cretaceous shortening and early Tertiary shearing in the central Sierra Madre del Sur, southern Mexico: Insights into the evolution of the Caribbean-North American plate interaction. Tectonics,26(3). [ Links ]
Chadwick Jr, W. W., y Dieterich, J. H. (1995). Mechanical modeling of circumferential and radial dike intrusion on Galapagos volcanoes. Journal of Volcanology and Geothermal Research,66(1-4), 37-52. [ Links ]
Chadwick, W. W., y Howard, K. A. (1991). The pattern of circumferential and radial eruptive fissures on the volcanoes of Fernandina and Isabela islands, Galapagos. Bulletin of Volcanology,53, 259-275. [ Links ]
Daniels, K. A., Kavanagh, J. L., Menand, T., y Stephen, J. S. R. (2012). The shapes of dikes: Evidence for the influence of cooling and inelastic deformation. Bulletin, 124(7-8), 1102-1112. https://doi.org/10.1130/b30537.1 [ Links ]
Delaney, P. T., Pollard, D. D., Ziony, J. I., y McKee, E. H. (1986). Field relations between dikes and joints: Emplacement processes and paleostress analysis. Journal of Geophysical Research, 91(B5), 4920-4938. [ Links ]
Elías-Herrera, M., Ortega-Gutiérrez, F., Sánchez-Zavala, J. L., Macías-Romo, C., Ortega-Rivera, A., y Iriondo, A. (2005). La falla de Caltepec: raíces expuestas de una frontera tectónica de larga vida entre dos terrenos continentales del sur de México. Boletín de la Sociedad Geológica Mexicana,57(1), 83-109. [ Links ]
Elías-Herrera, M., y Ortega-Gutiérrez, F. (2002). Caltepec fault zone: An Early Permian dextral transpressional boundary between the Proterozoic Oaxacan and Paleozoic Acatlán implications: Tectonics, 21(3), 4-1-4-18. [ Links ]
Ferrari, L., Bergomi, M., Martini, M., Tunesi, A., Orozco‐Esquivel, T., y López‐Martínez, M. (2014). Late Cretaceous‐Oligocene magmatic record in southern Mexico: The case for a temporal slab window along the evolving Caribbean‐North America‐Farallon triple boundary. Tectonics,33(9), 1738-1765. [ Links ]
Ferrusquía-Villafranca, I. (1976). Estudios geológico-paleontológicos en la región Mixteca PT 1 Geología del área Tamazulapan-Teposcolula-Yanhuitlán, Mixteca Alta, Estado de Oaxaca México. Boletín del Instituto de Geología, 97-155. [ Links ]
Ferrusquía, I., Segura, L. R., y Rodrigues-Torres, T. (1970). Geología del área Tamazulapan-Teposcolula-Yanhuitlán, Mixteca Alta, Estado de Oaxaca. Excursión geológica México-Oaxaca: Sociedad Geológica Mexicana, 97-119. [ Links ]
Geshi, N., y Oikawa, T. (2016). Orientation of the eruption fissures controlled by a shallow magma chamber in Miyakejima. Frontiers in Earth Science, 4, 99. https://doi.org/10.3389/feart.2016.00099 [ Links ]
Gonnermann, H., y Taisne, B. (2015). Magma transport in dikes. EnThe encyclopedia of volcanoes(pp. 215-224). Academic Press. [ Links ]
Gudmundsson, A., y Marinoni, L. B. (1999). Geometry, emplacement, and arrest of dykes. EnAnnales Tectonicae,13(1/2), 71-92. [ Links ]
Hernández Santana, J. R., Ortiz Pérez, M. A., y Zamorano Orozco, J. J. (1995). Regionalización morfoestructural de la Sierra Madre del Sur, México. Investigaciones geográficas, 31, 45-67. [ Links ]
Hjartardóttir, Á. R., Einarsson, P., Bramham, E., y Wright, T. J. (2012). The Krafla fissure swarm, Iceland, and its formation by rifting events. Bulletin of Volcanology,74, 2139-2153. [ Links ]
Hoek, J. D. (1991). A classification of dyke-fracture geometry with examples from Precambrian dyke swarms in the Vestfold Hills, Antarctica. Geologische Rundschau,80, 233-248. [ Links ]
Hyndman, D. W., y Alt, D. (1987). Radial dikes, laccoliths, and gelatin models. The Journal of Geology,95(6), 763-774. [ Links ]
Janosy, R. J. (1994). Structural investigations of the early Paleozoic Victoria Land dike swarm in the Ferrar-Koettlitz Glacier region, southern Victoria Land, Antarctica. Tesis de doctorado. Ohio State University. [ Links ]
Keppie, J. D., y Morán-Zenteno, D. J. (2005). Tectonic implications of alternative Cenozoic reconstructions for southern Mexico and the Chortis Block. International Geology Review,47(5), 473-491. https://doi.org/10.2747/0020-6814.47.5.473 [ Links ]
Llambias, E. J., y Sato, A. M. (1990). El Batolito de Colangüil (29-31° S) cordillera frontal de Argentina: estructura y marco tectonico. Andean Geology,17(1), 89-108. [ Links ]
López-Ticha, D. (1985). Revisión de la estratigrafía y potencial petrolero de la Cuenca de Tlaxiaco. Boletín de la Asociación Mexicana de Geólogos Petroleros,37(1). [ Links ]
Mäkitie, H., Data, G., Isabirye, E., Mänttäri, I., Huhma, H., Klausen, M. B., ... y Virransalo, P. (2014). Petrology, geochronology and emplacement model of the giant 1.37 Ga arcuate Lake Victoria Dyke Swarm on the margin of a large igneous province in eastern Africa. Journal of African Earth Sciences,97, 273-296. https://doi.org/10.1016/j.jafrearsci.2014.04.034 [ Links ]
Martiny, B., Martı́nez-Serrano, R. G., Morán-Zenteno, D. J., Macı́as-Romo, C., y Ayuso, R. A. (2000). Stratigraphy, geochemistry and tectonic significance of the Oligocene magmatic rocks of western Oaxaca, southern Mexico. Tectonophysics,318(1-4), 71-98. https://doi.org/10.1016/S0040-1951(99)00307-8 [ Links ]
Montes de Oca-Villatoro, A. (2021) Actualización cartográfica geológico- estructural de la porción Noroeste del Geoparque Mundial UNESCO Mixteca Alta. Tesis de licenciatura. Universidad Autónoma del Carmen, Campeche. [ Links ]
Morán-Zenteno, D. J., Martiny, B. M., Solari, L., Mori, L., Luna-González, L., y González-Torres, E. A. (2018). Cenozoic magmatism of the Sierra Madre del Sur and tectonic truncation of the Pacific margin of southern Mexico. Earth-Science Reviews,183, 85-114. https://doi.org/10.1016/j.earscirev.2017.01.010 [ Links ]
Morán-Zenteno, D. J., Cerca, M., y Keppie, J. D. (2007). The Cenozoic tectonic and magmatic evolution of southwestern México: Advances and problems of interpretation. https://doi.org/10.1130/2007.2422(03) [ Links ]
Morán-Zenteno, D. J., Martiny, B., Tolson, G., Solís Pichardo, G., Alba Aldave, L., Hernández-Bernal, M. D. S., y Silva Romo, G. (2000) Geocronología y características geoquímicas de las rocas magmáticas terciarias de la Sierra Madre del Sur. Boletín de la Sociedad Geológica Mexicana , 53, 27-58. [ Links ]
Morán-Zenteno, D. J., Tolson, G., Martınez-Serrano, R. G., Martiny, B., Schaaf, P., Silva-Romo, G., ... y Solıs-Pichardo, G. N. (1999). Tertiary arc-magmatism of the Sierra Madre del Sur, Mexico, and its transition to the volcanic activity of the Trans-Mexican Volcanic Belt. Journal of South American Earth Sciences,12(6), 513-535. https://doi.org/10.1016/S0895-9811(99)00036-X [ Links ]
Muller, O. H., y Pollard, D. D. (1977). The stress state near Spanish Peaks, Colorado determined from a dike pattern. Pure and Applied Geophysics,115, 69-86. https://doi.org/10.1007/BF01637098 [ Links ]
Nakamura, K. (1977). Volcanoes as possible indicators of tectonic stress orientation-principle and proposal. Journal of Volcanology and Geothermal Research, 2(1), 1-16. https://doi.org/10.1007/BF01637099 [ Links ]
Nieto-Samaniego, A. F., Alaniz-Álvarez, S. A., Silva-Romo, G., Eguiza-Castro, M. H., & Mendoza-Rosales, C. C. (2006). Latest Cretaceous to Miocene deformation events in the eastern Sierra Madre del Sur, Mexico, inferred from the geometry and age of major structures. Geological Society of America Bulletin,118(1-2), 238-252. [ Links ]
Ollier, C. D. (1971). Causes of spheroidal weathering. Earth-Science Reviews, 7(3), 127-141. https://doi.org/10.1016/0012-8252(71)90005-55 [ Links ]
Ortega-González, J. V., y Lambarria-Silva, C. (1991). Informe geológico del prospecto Hoja Oaxaca, compilación geológica IGRS 1129: Petróleos Mexicanos (PEMEX). Coordinación Divisional de Exploración, Gerencia de Exploración Región Sur, Subgerencia de Geología superficial y Geoquímica, Reporte inédito. [ Links ]
Palacio-Prieto, J. L. P., De Castro-Martínez, G. F., y González, E. M. R. (2019). Geosenderos en el Geoparque Mundial Unesco Mixteca Alta, Oaxaca, México. Cuadernos Geográficos,58(2), 111-125. [ Links ]
Palacio-Prieto, J. L., Rosado-González, E., Ramírez-Miguel, X., Oropeza-Orozco, O., Cram-Heydrich, S., Ortiz-Pérez, M. A., ... y de Castro-Martínez, G. F. (2016). Erosion, culture and geoheritage; the case of Santo Domingo Yanhuitlán, Oaxaca, México. Geoheritage, 8, 359-369. https://doi.org/10.1007/s12371-016-0175-2 [ Links ]
Peacock, D. C. P., Nixon, C. W., Rotevatn, A., Sanderson, D. J., & Zuluaga, L. F. (2016). Glossary of fault and other fracture networks. Journal of Structural Geology,92, 12-29. https://doi.org/10.1016/j.jsg.2016.09.008 [ Links ]
Pindell, J. L., & Kennan, L. (2009). Tectonic evolution of the Gulf of Mexico, Caribbean and northern South America in the mantle reference frame: an update. Geological Society, London, Special Publications,328(1), 1-55. https://doi.org/10.1144/SP328. [ Links ]
Poland, M. P., Moats, W. P., y Fink, J. H. (2008). A model for radial dike emplacement in composite cones based on observations from Summer Coon volcano, Colorado, USA. Bulletin of Volcanology,70, 861-875. [ Links ]
Pollard, D. D., Segall, P., y Delaney, P. T. (1982). Formation and interpretation of dilatant echelon cracks. Geological Society of America Bulletin ,93(12), 1291-1303. [ Links ]
Rogers, R. D., Mann, P., y Emmet, P. A. (2007). Tectonic terranes of the Chortis block based on integration of regional aeromagnetic and geologic data. [ Links ]
Santamaría-Diaz. A. (2009a). Cartografía geológica de la región de Tamazulapam, Nochixtlán, Chalcatongo, Yosundua, en el sur de México. Mexico: Universidad Tecnológica de la Mixteca. [ Links ]
Santamaría-Diaz, A. (2009b). Influencia de la falla de basamento no expuesta en la deformación Cenozoica: la falla de Caltepec en la región de Tamazulapam, en el sur de México. México: Universidad Tecnológica de la Mixteca. [ Links ]
Santamaría-Díaz, A., Alaniz-Álvarez, S. A., y Nieto-Samaniego, Á. F. (2008). Deformaciones cenozoicas en la cobertura de la falla Caltepec en la región de Tamazulapam, sur de México. Revista mexicana de ciencias geológicas,25(3), 494-516. [ Links ]
Sedlock, R. L., Ortega-Gutiérrez, F., y Speed, R. C. (1993). Tectonostratigraphic terranes and tectonic evolution of Mexico. GSA Spewcial Papers. https://doi.org/10.1130/SPE278-p1 [ Links ]
Received: February 23, 2023; Accepted: April 12, 2023; Published: May 26, 2023