Introduction
In Mexico, tomato cultivation is one of the most important agricultural activities, with a record production of 3,441,639.37 t in 2019 (SIAP, 2021). Various abiotic and biotic stresses affect tomato production and fruit quality. Among the biotic stresses, diseases are one of the most important challenging components, leading to serious effects on plant growth and productivity (Sharma and Bhattarai, 2019). The bacterial spot, caused by Xanthomonas vesicatoria, X. euvesicatoria, X. perforans and X. gardneri, is one of the most important diseases in tomato (Jones et al., 2004; Strayer et al., 2016). The plants with bacterial spot disease present small yellow-green lesions on leaves, progressing into light dark, and as the infection progresses the leaves become deformed and develop dark necrotic lesions (Vallad et al., 2004). In addition, this infection leads to defoliation and fruits with lesion, ultimately causing severe reduction in tomato productivity (Potnis et al., 2015). The control of bacterial spot in field is challenging, especially during warm and humid conditions, which characterize the main tomato producing areas in Mexico.
The chemical control has been a primary focus in past management strategies for bacterial spot tomato. The application in plants of copper-based bactericides, alone or combined with fungicides, such as mancozeb (ethylene-bis-dithiocarbamates), and antibiotics, such as streptomycin or kasugamycin, are the main strategies for the control of bacterial spot (Vallad et al., 2010; Šević et al., 2019). However, races of Xanthomonas resistant to copper and streptomycin are commonly present in field since 1960s (Thayer and Stall, 1961). In addition, the genes that encode resistance to copper and streptomycin can be transmitted through plasmids, allowing the rapid spread of resistance to other non-resistant bacteria (Ritchie, 2000). On the other hand, breeding programs to confer resistance to tomato plants against bacterial spot by insertion of resistance genes (R-genes) have been unsuccessful, due to apparition of new strains of the pathogen that overcome resistance (Adhikari et al., 2020).
Due to the high resistance of Xanthomonas to bactericides and the limited success of breeding programs to provided resistance to tomato plants against the bacterial spot, the identification and exploration for alternative control strategies become mandatory. The application of inducers in plants to confer a physiological state of resistance against phytopathogens has shown be alternative strategy in the control of diseases (Dewen et al., 2017). In addition, the resistance state in tomato induced against the bacterial spot has shown levels of efficiency, similar or superior to the standard copper bactericide control methods (Louws et al., 2001).
The plants have developed a unique ability to identify the pathogen microbes that cause infection through conserved microbial molecules known as pathogen-associated molecular pattern (PAMP) and these conserved molecules are elicitors of immunity system (Boller and Felix, 2009; Zipfel, 2014). On the other hand, extracellular immune receptor in the plant cell known as pattern recognition receptors (PRR) identify PAMP (Bhattarai et al., 2016). The perception of PAMP by PRR triggers the signaling of a state of immunity in plants known as immunity triggered by PAMP (ITP). This state of immunity confers resistance at local sites of infection of pathogens and contributes to the signaling for the systemic activation of resistance against future pathogenic attacks (Wu et al., 2014).
The use of PAMP inductors, e.g. proteins, peptides, oligosaccharides, lipids, nucleic acids, or any other cellular component, have shown relevance as an effective strategy for the control of phytopathogens (Dewen et al., 2017). Particularly, proteins obtained from Bacillus amyloliquefaciens and B. pumilus induce resistance against bacterial spot of X. vesicatoria in tomato (Lanna-Filho et al., 2013); also, polysaccharides obtained from X. gardneri induce resistance to bacterial spot in tomato (Luiz et al., 2015). In contrast, there are few reports about the ability of biological molecules belonging to X. vesicatoria to protect the plants against bacterial diseases (Gottig et al., 2018). The aim of this study was to determinate the effect of X. vesicatoria protein solutions as inducers of resistance against bacterial spot in tomato plants under in vitro conditions.
Materials and methods
Phytopathogenic bacteria and plant material
The BV801 strain of X. vesicatoria (BV801) was provided by the Phytopathology Laboratory of CIATEJ and was cultivated in NYG liquid medium (0.5 % peptone (N), 0.3 % yeast extract (Y), 2 % glycerol (G)) at 28 °C for 36 h on a rotary shaker at 200 rpm. The plant material was obtained from tomato seeds cv. Maya (Seminis®), which were sterilized with 3 % sodium hypochlorite for 5 min and washed with sterile distilled water. The seeds were germinated in vitro on MS medium (Murashige and Skoog, 1962) at 26 °C for 5 d. The seedlings were transferred to Half-closed Arbuscular Mycorrhizal - Plant (HAM-P) system described by Voets et al. (2005), with the following modification: a 12 mm diameter perforation was made on the lid of the Petri dish. Through this hole, the MS medium was renewal each 7 days and a sterile rubber plug was used to cover the perforation (Figure 1).
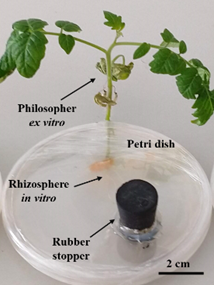
Figure 1 Modified Half-closed Arbuscular Mycorrhizal-Plant (HAM-P) system (Voets et al., 2005) with modifications (rubber stopper).
Protein extraction and quantification
The BV801 strain cultivated in NYG liquid medium was collected by centrifugation at 4 °C for 10 min at 8,000 rpm, and was washed three times with 1X PBS (Phosphate buffered Saline). The cells were lysed in SDS-Tris buffer (0.1 % Sodium Dodecyl Sulfate, Tris-base 100 mM and adjusted to pH 9.5), and the cell disruption was performed in a ultrasonicator at 20 kHz at 4 °C for 2 min with intervals of 30 sec. The sample was centrifuged at 4 °C for 30 min and 12,000 rpm and the supernatants were used for determination of soluble proteins. Protein quantification was performed using the Bradford assay and the BSA protein standard (Bradford, 1976). To determine the protein profile, the protein solutions was adjusted to a concentration of 31.25, 62.5, 125, 250, 500 and 1000 µg mL−1. For the tests of antibacterial activity induction of plant resistance proteins, solutions were adjusted in the concentrations of 0.001, 0.01, 0.1, 1.0, 10 and 100 μg mL−1. Protein solutions were stored at -20 °C until use.
Protein profile of X. vesicatoria strain BV801 by SDS-PAGE
The SDS-PAGE protocol (sodium dodecyl sulfate polyacrylamide gel electrophoresis) was used for protein profiling as described by Laemmli (1970). The stacking gel contained 4 % acrylamide (0.5 M Tris-HCl, adjusted to pH 6.8 10 % SDS w/v) and resolution of gel of acrylamide 12 % (1.5 M Tris-HCl, pH 8.8, 10 % SDS w/v) was made. Samples of 5 μL of protein solutions (31.25, 62.5, 125, 250, 500 and 1000 µg mL-1) were loaded with 4.75 μL of Laemmli sample buffer and 0.25 μL of β-mercaptoethanol and denatured at 95 °C for 5 min. Electrophoresis was performed in 1X SDS-PAGE buffer (250 mM tris, 1.92 M glycine, 1 % SDS, pH 8.3). The electrophoresis conditions were 120 volts at 80 amps per 100 min. Gel staining with Coomassie R-250 (50 % methanol, 10 % acetic acid, 0.25 % coomassie blue R-250) was performed. The excessing dye solution was removed with fading solution. The visualization and capture of images of the gels were carried out in a photodocumentor (Bio-Rad®, Gel Doc XR System).
Antibacterial activity of protein solutions
Antibacterial activity of BV801 proteins solutions were determinate in vitro test of inhibition in double-layer plaque as described by Cavalcanti et al. (2006) with minor modifications. BV801 bacterial strain was cultivated in NYG liquid medium and concentration was adjusted at 1×108 CFU mL−1 using a spectrophotometer (Eppendorf®, BioPhotometer) at 600 nm and optical density (OD) of 1. The double-layer plaque was prepared by adding 100 uL of bacterial suspension OD = 1 to 4.9 mL of NYGA (0.5 % peptone, 0.3 % yeast extract, 2 % glycerol, 0.7 % agar) at 40 °C and allowed to gel at room temperature. The different proteins solutions (0.001, 0.01, 0.1, 1.0, 10 and 100 μg mL−1) and SDS-Tris solution (dissolution buffer of proteins without protein) were added in drops of 10 μL on the double-layer plaque and allowed to dry under hood flow. Neomycin (1 μg μL−1) and sterile distilled water were used as control positive and negative of inhibitors, respectively. The Petri plates were incubated at 28 °C for 48 h and the inhibition halo of X. vesicatoria by proteins solutions and controls were registered. The experimental design was completely randomized and six repetitions per treatment were used.
Induction of resistance test in tomato against bacterial spot
An experiment was conducted in a completely randomized design with eight treatments and seven repetitions. The treatments were plants inoculated with the strain BV801 of X. vesicatoria and the application of 7 concentrations of Xanthomonas proteins solutions: 0.0 (diseased plants), 0.001, 0.01, 0.1, 1.0, 10 and 100 μg mL-1, and a control without protein and without phytopathogenic bacteria (healthy plants). The protein solutions were sprayed on both sides (adaxial and abaxial) of leaves with the help of a nozzle sprayer (≈0.5 mL / plant) (ATO50 Truper®). The protein solutions were subjected to 95 °C for 5 min, to promote the deployment of secondary protein structure and promote epidermal exposure. Protein solutions are allowed to cool to room temperature before being applied to the plant. After 36 h, the bacterial (BV801 strain) suspension (1×108 CFU mL−1) was applied on leaves. The plants were kept at 28 °C, 55 % HR and 10/14 h light/dark in bioclimatic chamber (SEV-PRENDO-México, INCL-20) for 14 days.
Severity of disease in tomato plants and plant growth parameters
The severity of X. vesicatoria in tomato plants was determined with a scale of 0 to 5 (Figure 2). The scale was based on percentage of tissue damaged due to inoculation of X. vesicatoria (0 = healthy plant, 1 = 20 %, 2 = 40 %, 3 = 60 %, 4 = 80 % and 5 = dead plant). The plant height (cm), stem diameter (mm), root length (cm), fresh biomass (g), dry biomass (g), number of branches and number of leaves were quantified at the end of the experiment.
Statistical data analysis
The severity scale was analyzed using Kruskal-Wallis nonparametric technique and median confidence interval (P ≤ 0.05). One-way analysis of variance (ANOVA) was performed on growth variables, that were compared with Tukey (P ≤ 0.05). A Bonferroni statistical test was used for dry biomass. The data were analyzed using Statgraphics centurion XVII (http://www.statgraphics.com/).
Results
Protein solutions profile of X. vesicatoria and antibacterial activity
The proteins profile on one dimension were obtained from X. vesicatoria, and approximately 35 bands conform the protein profile (1000 µg mL−1). Particularly, 19 protein bands have a higher proportion compared to the 16 others protein bands, evidenced by the dilution of 125 and 250 µg mL−1 (Figure 3). In the image, the arrows on the right indicate the position of the 19 main protein bands, whose estimated molecular weights were 10, 12, 15, 17, 20, 23, 25, 30, 35, 40, 45, 55, 60, 65, 70, 80, 100, 110, and 200 kDA. The bacterial growth inhibition test shown that only the antibiotic neomycin (1 μg μL−1) had a direct inhibitory effect on the growth of X. vesicatoria (Figure 4). These results indicate the absence of compounds with antibacterial activity in the protein solutions from strain BV801 and in the protein dissolution buffer (SDS-Tris) solution.
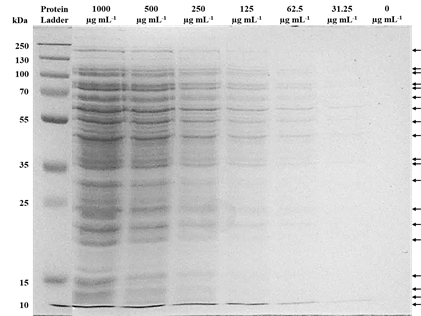
Figure 3 Protein profile obtained from X. vesicatoria visualized by SDS-PAGE method. The concentration of protein loaded in each lane is indicated at the top of the image. Protein ladder molecular weight of 10 to 250 kDa (PageRuler™ Plus Prestained Protein Ladder, Thermo Scientific™). The arrows on the right indicate the position of the 19 main protein bands.
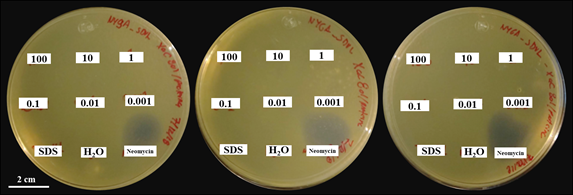
Figure 4 Bacterial growth inhibition test. The plates show experimental replicates. The numbers on the plates indicate the concentration of protein solutions applied in μg mL−1. SDS: protein dissolution buffer, H2O: sterile distilled water (negative inhibition control), antibiotic neomycin (positive inhibition control: 10 μL at 1 μg μL−1).
Effects of protein solutions on the disease severity of bacterial spot and growth plant
Significant effects were found in the reduction of the severity of the bacterial spot (95 % median confidence interval), due to the application of protein solutions from X. vesicatoria. Particularly, plants treated with protein solutions 0.1, 1.0 and 10 μg mL−1 showed a significant reduction on the severity scale by 55, 50 and 50 %, respectively, with respect to diseased plants (without protein solutions and inoculated with X. vesicatoria) (Figure 5). The protective effect of these protein solution treatments was demonstrated by significant reduction (test Kruskal-Wallis, P = 0.0035) in the severity of disease at 14 days after exposure to phytopathogenic bacteria (Figure 6). On the other hand, the biomass of plants (wet and dry) treated with protein solutions and healthy plants (without protein solutions and without X. vesicatoria) were statistically equal, but diseased plants (without protein solutions and inoculated with BV801) were significantly (Tukey and Bonferroni, P ≤ 0.05) lesser that healthy plants (Table 1). Likewise, the number of leaves and root length of healthy plants were significantly (Tukey, P ≤ 0.05) higher to diseased plants untreated with protein solutions. On the other hand, all growth variables of plants treated with 0.1 and 1.0 μg mL−1 protein solutions were statistically equal (Tukey, P ≤ 0.05) to healthy plants. No statistical differences were observed in plant height, stem diameter and number of branches.
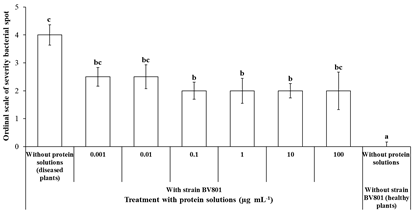
Figure 5 Effect of the application of bacterial protein solutions in tomato plants against bacterial spot caused by X. vesicatoria under in vitro conditions. Different letters indicate statistical differences according to the nonparametric test of Kruskal-Wallis (P = 0.0035) and 95 % confidence intervals of median. The bar represents the standard error.
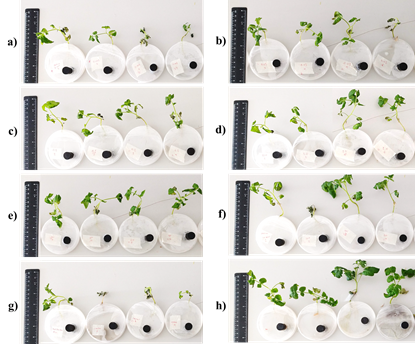
Figure 6 Plants treatments with X. vesicatoria strain BV8011 plus protein solutions (μg mL −1 ): a) 0.001, b) 0.01, c) 0.1, d) 1.0, e) 10, f) 100, g) Diseased plants (without protein solutions and inoculated with X. vesicatoria) and h) Healthy plants (without protein solutions and without X. vesicatoria). Left side: scale with length = 14 cm.
Table 1 Effect of application of bacterial protein solutions on plant growth of tomato plants under in vitro conditions.
Treatment | Plant height (cm) | Stem diameter (mm) | Number of branches | Number of leaf | Root length (cm) | Total biomass (g) | ||
Strain BV801 | Protein solutions (µg mL-1) | Wet | Dry* | |||||
With | 100 | 5.9±0.9 a | 1.7±0.2 a | 4.2±0.5 a | 11.8±1.6 ab | 12.7±2.8 ab | 0.9±0.3 ab | 0.05±0.1 b |
10 | 6.3±0.2 a | 1.8±0.1 a | 3.7±0.2 a | 10.2±1.0 b | 11.0±2.1 ab | 0.8±0.2 ab | 0.07±0.1 ab | |
1.0 | 6.4±0.7 a | 1.8±0.2 a | 4.3±0.3 a | 11.8±1.7 ab | 13.7±2.6 ab | 0.9±0.2 ab | 0.06±0.1 ab | |
0.1 | 6.4±0.3 a | 1.9±0.1 a | 4.3±0.2 a | 11.7±1.0 ab | 16.0±1.3 a | 0.9±0.1 ab | 0.07±0.1 ab | |
0.01 | 5.7±0.3 a | 1.9±0.1 a | 3.7±0.2 a | 10.5±0.6 ab | 11.3±0.9 ab | 0.7±0.1 b | 0.05±0.1 b | |
0.001 | 5.9±0.5 a | 1.8±0.1 a | 3.7±0.2 a | 9.30±0.7 b | 11.8±1.4 ab | 0.7±0.2 ab | 0.04±0.1 b | |
0† | 5.3±0.9 a | 1.5±0.2 a | 3.0±0.4 a | 7.00±0.9 b | 8.3±1.00 b | 0.6±0.1 b | 0.04±0.1 b | |
Without | 0‡ | 6.7±0.5 a | 2.1±0.1 a | 4.1±0.3 a | 15.7±1.5 a | 16.4±1.5 a | 1.4±0.2 a | 0.10±0.1 a |
Means with the same letter in column indicate no statistical differences according to Tukey and *Bonferroni statistical test (P ≤ 0.05). Standard error (±). †Diseased plants: without protein solutions and inoculated with BV801. ‡Healthy plants: without protein solutions and without BV801.
Discussion
The use of PAMP inductors in tomato to activate resistance state against phytopathogens has been an effective strategy in the control of bacterial spot infection (Blainski et al., 2017; Luiz et al., 2015; Lanna-filho et al., 2013; Cavalcanti et al., 2007). According to Cavalcanti et al. (2007), a state of resistance induced in plants is characterized by the reduction of the size and number of injuries that develop after the inoculation of a phytopathogen, which agrees with the reduction of scale of severity in plants observed when applying treatments of 0.1, 1.0 and 10 μg mL−1 of proteins solutions. Particularly, protein solutions from BV801 showed no capacity to inhibit in vitro growth of the X. vesicatoria, which suggests the absence of compounds with antibacterial activity in these protein solutions. Therefore, in this work the control mechanisms of the bacterial spot in tomato observed were related to the induction of resistance through PAMP inductors.
Particularly, the use of resistance inductors in tomato has shown the activation of resistance mechanisms that activate enzymes involved in resistance to oxidative stress such as catalase, superoxide dismutase and glutathione reductase (Blainski et al., 2018), and increased gene expression of pathogenesis-related proteins (PR-genes), PR1a and PR1b (Huang and Vallad, 2018). Systemic acquired resistance (SAR) and induced systemic resistance (ISR) are two forms of induced resistance, wherein plant defences are preconditioned by prior infection or treatment, that results in resistance against subsequent challenge by a pathogen (Reimer-Michalski and Conrath, 2016). The SAR is characterized by pathogen-related proteins (PR proteins) and can enhance cellular responses and defense against phytopathogens. In Arabidopsis, localized applications PAMP showed elevated levels of salicylic acid, the SAR regulatory metabolite and PR-genes expression (Mishina and Zeier, 2007). Together, this mechanisms limit progress and subsequent development of phytopathogens attack. Probably, these mechanisms could have been present in tomato plants treated with protein solutions of 0.1, 1.0 and 10 μg mL-1, treatments that showed less damage to infection by X. vesicatoria. However, further studies are necessary to corroborate these assumptions.
In regard to the use of PMAP inductors to activate resistance, in Xanthomonas genus has been reported that pathogenicity and hypersensitive response proteins (Hrp-proteins) of 20 to 25 kDA are PAMPs and that are involved in the induction of the defense response of plants (Gottig et al., 2018). In this research, the resolution of the BV801 protein profile showed the accumulation of protein bands 20, 23 and 25 kDA, probably related with Hrp-proteins. On the other hand, it has been indicated that flagellin peptides of 22 amino acids from Xanthomonas (flg22-Xac) are PAMPs that induce resistance to bacterial spot in tomato, and whose immune perception is mediated by PRR as FLS2 described in Arabidopsis (Bhattarai et al., 2016). Bacterial flagellin is related whit the motility of bacterial and is a PAMP preserved in Xanthomonas and it could be present in the BV801 protein solutions evaluated with inductors. However, more studies are necessary to confirm that flagellin peptides, as well as Hrp-proteins, are present in protein solutions of BV801.
Conclusion
In this research, we demonstrate that X. vesicatoria strain BV801 protein solutions contain PAMP that maintain eliciting activity of the plant immunity system and that they could be involved in the activation of the resistance state against pathogens. This protein solution did not show antibacterial activity. The presence of proteins that maintained PMAP activity allowed them to be recognized by the immune system of S. lycopersicum, leading to the activation of a state of resistance.