Introduction
The use of medicinal plants in Mexico has been documented since the pre-Hispanic age (Alonso-Castro et al., 2017). These resources are usually considered part of the traditional cultural knowledge of indigenous groups such as Mayan, Nahua, and Zapotec ethnicities (Heinrich, 2000), and nowadays, health professionals and practitioners use such medicinal plants (Alonso-Castro et al., 2017; Sharma et al., 2021). Furthermore, the wide range of metabolites present in medicinal plants could be useful for agriculture, nutrition, and the discovery of novel drugs (Dewick, 1997; Cseke et al., 2006; Singh et al., 2020). For this reason, plant bioprospecting has been frequently used as a strategy to identify and describe bioactive metabolites with medicinal properties (Anand et al. 2019; Landa-Cansigno et al., 2020; Monribot-Villanueva et al., 2020), as defense mechanisms by interfering with molecular targets in the cell of herbivores (Valencia-Mejía et al., 2022), microbes (Alvin et al., 2014; Ramírez-Reyes et al., 2019; Bonilla-Landa et al., 2022), or can affect cell signaling or protect against oxidative or UV stress (Kasote et al., 2015).
The Burseraceae family includes ca. 600 tropical and temperate species distributed into 20 genera (Tucker et al., 2009). Some of these species contain secondary metabolites such as triterpenes and steroids (Álvarez et al., 2015), phenolics (Maldini et al., 2009b), and lignans (Gigliarelli et al., 2015), which are mainly found in the bark resin. In addition, extracts of some species have exhibited antioxidant (Kitts et al., 1999), antibacterial (Borsato et al., 2000), and antitumoral (Jang et al., 2004) properties. Bursera Jacq. ex L. (Burseraceae) is a woody genus with 126 valid species (WFO, 2022), 92 of which are found in Mexico (Marcotullio et al., 2018). Some of them are economically important due to their resins and essential oils, which are used as incense in temples and religious ceremonies (i.e., “copal”), as well as cosmetics, such as varnish, and in traditional medicine for the treatment of several illnesses (Rzedowski and Calderón de Rzedowski, 1996).
Bursera simaruba Sarg. (Burseraceae), commonly named “mulato”, “palo mulato”, “chacá”, “cohite” or “cuajiote”, is widely distributed in the state of Veracruz, Mexico (Rzedowski and Calderón de Rzedowski, 1996), and is a representative element of tropical forests in North and Central America (Pennington and Sarukhán, 1998). This species is valuable for ecological restoration and reforestation (Orantes-García et al., 2013). Mature trees of B. simaruba are used as windbreak barriers (Avendaño Reyes and Acosta Rosado, 2016) and living fences (Castellanos-Castro and Bonfil, 2010). The wood of B. simaruba is used for the manufacture of handicrafts, firewood, and charcoal, while the fruits are utilized by the local population as food or forage material for livestock (Rico-Gray et al., 1991). In addition, there are reports of the leaves of B. simaruba being used for the treatment of fever symptoms (Ankli et al., 1999), dermatitis (DeCarlo et al., 2019), urinary tract diseases (Rzedowski and Calderón de Rzedowski, 1996), and diabetes (Castro-Juárez et al., 2014), as well as evidence of their antioxidant (Sánchez-Medina et al., 2001), anti-inflammatory (Noguera et al., 2004) and antibacterial activities (Yasunaka et al., 2005). It has also been recorded as a β-glucosidase inhibitor (Sánchez-Medina et al., 2001). However, the phytochemistry of B. simaruba leaves has been poorly addressed, and most of the metabolites responsible for such biological effects remain unknown.
Therefore, the objectives of this study were: 1) to determine the main chemical groups in B. simaruba leaves using qualitative tests; 2) to identify metabolites from the methanolic extract of B. simaruba leaves by using ultra high-resolution liquid chromatography coupled to high-resolution mass spectrometry; 3) to determine the antibacterial, antifungal, and antidiabetic in vitro activities of the methanolic extract of B. simaruba leaves and to correlate such activities with the traditional uses of this plant; and 4) to test the antidiabetic activities of pure compounds identified in the methanolic extract of B. simaruba leaves.
Materials and Methods
Plant collection and identification
Plant material (B. simaruba leaves) was collected in the municipality Puente Nacional, Veracruz, Mexico (19°19'43"N, 96°28'57"W) from three individuals. Taxonomic determination was performed using keys and descriptions previously published by Rzedowski and Calderón de Rzedowski (1996) and corroborated by Biol. Carlos Durán and Dr. Sergio Avendaño (curators of the Herbarium XAL of the Instituto de Ecología, A.C.). A duplicate specimen was deposited in the Herbarium XAL (D. A. Infante voucher number 01 (XAL0106195)).
Preparation of extract
Fresh leaves (~500 g) of B. simaruba were pooled and then washed with distilled water, dried, and stored at (80 °C before lyophilization in a freeze dryer (FreeZone 1, Labconco, Kansas, MO, USA) for six days. Afterwards, dried leaves were randomly divided into three groups, pulverized, and stored at 4 °C. Methanolic extracts (MeOH-Ex) were obtained with an accelerated solvent extraction system (ASE 350, Dionex Corporation, Sunnyvale, CA, USA) following the protocol previously described by Juárez-Trujillo et al. (2018). For this purpose, 3 g of plant material were mixed with 1 g of diatomaceous earth (Thermo Scientific, Waltham, MA, USA) and placed in a 34 ml cell. It was then subjected to a single static cycle of 15 minutes at 60 °C. Then, MeOH-Ex was concentrated by eliminating the solvent using rotary evaporation under reduced pressure at 40 °C (RII, Büchi, Flawil, Switzerland).
Phytochemical screening for detecting secondary metabolites
Qualitative phytochemical tests were carried out with 1 mg of MeOH-Ex of each sample following the methodologies described by Domínguez (1973) and Vásquez-Morales et al. (2022) for total determinations of terpenes, steroids, phenols, alkaloids, saponins, flavonoids, tannins, and coumarins, as briefly described in Table 1.
Table 1: Methodology used to identify secondary metabolites groups by qualitative tests according to Domínguez (1973) and Vásquez-Morales et al. (2022).
Chemical group | Methodology | Positive test |
---|---|---|
Terpenes and steroids | Standard procedure with acetic anhydride and sulfuric acid | Blue, green, red, or violet coloration |
Phenols | Standard procedure with iron chloride | Black, blue, or green coloration |
Alkaloids | Dragendorff´s reagent | Turbidity or precipitate formation |
Saponins | Standard procedure with distilled water | Abundant foam was formed and remained stable at least for 5 min |
Flavonoids | Shinoda’s test | Orange, pink, red, or violet coloration |
Tannins | Gelatin-salt reagent | Turbidity or precipitate formation |
Coumarins | Standard procedure with sodium hydroxide | Green, red, or yellow fluorescence |
Chemical profiling by UPLC-ESI+-MS-QTOF
Chemical profiling of MeOH-Ex was performed as was previously described by Monribot-Villanueva et al. (2020). The analysis was carried out using an ultra-high-resolution chromatographic system (UPLC, Class I, Waters, Milford, MA, USA) coupled with a quadrupole time of flight (QTOF) high-resolution mass spectrometer (Synapt G2-Si, Waters, Milford, MA, USA) with an electrospray ionization source in positive mode. Data were acquired and processed with the software MassLynx v. 4.1 and MarkerLynx v. 4.1 from Waters (Milford, MA, USA). The mass spectra of the major (relatively most abundant) chromatographic peaks were compared with public databases such as METLIN (2022), ChemSpider (2022), PubChem (2022), and Food Database (2022) using a maximum mass error of ±5 ppm as an accuracy criterium of chemical identity.
Biological activity assays
Antibacterial assay
The antibacterial potential of the MeOH-Ex of B. simaruba leaves was determined against the phytopathogenic Gram-negative Pseudomonas cichorii (Swingle 1925) Stapp 1928 and Pectobacterium carotovorum (Jones 1901) Waldee 1945, which are responsible for damaging various plant crops. Both pathogens belong to the collection of the “Laboratorio de Alta Tecnología de Xalapa (LATEX), Universidad Veracruzana (UV)”, Xalapa, Veracruz, Mexico, and strains of each were supplied by Dr. Mauricio Luna-Rodríguez (Facultad de Ciencias Agrícolas, Universidad Veracruzana, Xalapa, Mexico). These strains have been previously utilized and reported on by our research team (Ramírez-Reyes et al., 2018, 2019; Bonilla-Landa et al., 2022).
Bacteria strains were grown on King B agar (Sigma Aldrich, St. Louis, MO, USA), and the bacterial cultures were incubated under darkness at 26±2 °C for 24 h in a convection heat oven (Binder GmbH, Tuttlingen, Germany). The antibacterial activity of the MeOH-Ex of B. simaruba leaves was evaluated employing the agar-diffusion method according to Ramírez-Reyes et al. (2019). For this test, Petri dishes (90 mm diameter) were served with a mixture of King B agar (20 ml) and bacterial suspension (100 µl) in sterile water adjusted to a turbidity value of 0.5 according to the McFarland scale (approximately 1-2 × 108 colony-forming units per ml (UFC/ml)). In each Petri dish, wells of 1 cm in diameter (n=6) were drilled to receive each treatment. The MeOH-Ex (1 mg/ml) was dissolved into a H2O-AcOEt-DMSO mixture (2:1:1). The solvent mixture was used as a negative control, and oxytetracycline (Sigma Aldrich, St. Louis, MO, USA) at 2.1 mM dissolved in sterile water was used as a positive control. The plates were incubated for 24 h at 27 ºC in triplicate. Antibacterial activity was evaluated by measuring the diameter (mm) of the observed inhibition halo.
Antifungal assay
The antifungal potential of the MeOH-Ex of B. simaruba leaves was evaluated against Fusarium solani (Mart.) Sacc. (LAT-059), according to the protocol of Guevara-Avendaño et al. (2018) with minor modifications. This strain of F. solani belongs to the collection of LATEX (UV), and it was provided by Dr. Mauricio Luna-Rodríguez (UV), originally isolated from chili pepper (Capsicum annuum L.). For this assay, the fungal pathogen was grown on Potato Dextrose Agar (PDA, Difco, Detroit, MI, USA) at 28 °C for seven days before the antifungal assay. The surface of the fungus was scraped using an inoculation loop to obtain the spores and resuspended in 10 ml of sterile distilled water. Serial dilutions were made to obtain a suspension containing about 1 × 106 UFC/ml. The MeOH-Ex of B. simaruba leaves was dissolved in a solution of H₂O-MeOH (85:15) at a concentration of 2 mg/ml, mixed homogeneously with PDA medium, and poured into 12-well polystyrene microplates with a final volume of 4 ml. Thiabendazole (Sigma Aldrich, St. Louis, MO, USA) dissolved in H₂O (2 mg/ml) was used as a positive control, and 2 ml of H₂O-MeOH (85:15) mixed with PDA was used as a negative control. Wells of 1 cm in diameter were made and 2 µl of the spore suspension (1 × 106 UFC/ml) was added in the center. Microplates were incubated at 27±2 °C and the mycelial growth diameters (mm) were recorded at 48 h. Inhibition of fungal growth was calculated using the equation GI DC−DT /DC ×100 as was previously reported by Valencia-Mejía et al. (2022), where GI represents the percentage of growth inhibition (%), DC the average mycelial diameter of the positive control treatment (mm), and DT the mycelial diameter growth (mm).
Antidiabetic activity based on α-glucosidase and α-amylase enzymes inhibition
The antidiabetic activity of the MeOH-Ex of B. simaruba leaves was determined by in vitro enzymatic inhibitory assays on α-amylase and α-glucosidase, according to Landa-Cansigno et al. (2020). The α-glucosidase enzyme (≥100 U/mg protein) from the yeast Saccharomyces cerevisiae (Desm.) Meyen (Sigma Aldrich St. Louis, MO, USA) was diluted to 0.005 mg/ml in phosphate buffer (100 mM, pH 7.2, Sigma Aldrich, St. Louis, MO, USA). Then, 20 μl of extracts dissolved at 1 mg/ml in PB was mixed with 100 μl of 4-nitrophenyl-α-D-glucopyranoside (Sigma Aldrich, St. Louis, MO, USA) at 1 mM in PB. Acarbose (≥95%, Sigma Aldrich, St. Louis, MO, USA) was used as a positive control (30 mM). In addition, based on the analytical results, a commercially available standard of chlorogenic acid (0.35 mM, Sigma Aldrich, St. Louis, MO, USA) was also tested. The reaction mixture was incubated for 5 minutes at 30 °C. After the incubation time, the enzyme was added to each well, and the microplate was incubated for 30 min at 30 °C. Absorbance was measured at 405 nm at the beginning (Absblank) and after 30 min (Absextract) in a microwell spectrophotometer (Multiskan FC, Thermo Scientific, Waltham, MA, USA). Also, the absorbance of the enzymatic reaction without an inhibitor was measured (Abscontrol). The inhibition percentages (PI) were calculated with the equation
Testing the inhibition of the α-amylase enzyme (4 U/ml, Sigma Aldrich, St. Louis, MO, USA) was carried out by measuring the 2-chloro-4-nitrophenyl-α-D-maltotrioside (35 mM, Sigma Aldrich, St. Louis, MO, USA) hydrolysis. This assay was carried out in triplicate testing the MeOH-Ex of B. simaruba leaves (1 mg/ml) and chlorogenic acid (0.35 mM) dissolved in PB (40 mM, pH 6.8). Acarbose solution (3 mM) was used as a positive control at the beginning (Absblank) and the end (10 min) of the assay (Absextract). The absorbance of the enzymatic reaction without an inhibitor was also measured (Abscontrol). The PI was calculated in the same way as for the α-glucosidase assay.
Statistical analyses
Antibacterial, antifungal, α-glucosidase, and α-amylase assays were carried out in triplicate. Results are expressed as the average ± standard deviation of inhibition percentages. For each trial, a Shapiro-Wilks normality test was performed, and the data were analyzed with the nonparametric Kruskal-Wallis test followed by Dunn's Post-hoc test for group comparisons. All statistical analyses were performed with the Agricolae library (De Mendiburu, 2010) in R software v. 4.1.2 (R Core Team, 2020).
Results
Phytochemical profiling
The preliminary chemical screening of the MeOH-Ex of B. simaruba leaves exhibited a broad spectrum of metabolites (Table 2) where terpenes and phenolics exhibited the highest content, while alkaloids are moderated. In addition, a low content of saponins, flavonoids, tannins, and coumarins was detected (Table 2). On the other hand, the chemical profiling using ultra-high resolution liquid chromatography coupled with high-resolution mass spectrometry allowed us to tentatively identify ten phenolic compounds from different subcategories. Figure 1 shows a representative chromatogram of the MeOH-Ex of B. simaruba leaves and each identified compound is pointed out and marked with numbers (1-10). Table 3 shows the chromatographic and spectrometric data of each compound tentatively identified and marked in Figure 1. All tentative identifications were performed with a maximum mass error of ±5 ppm. Caffeic acid, chlorogenic acid, and the dihydrochalcone derivative phlorizin were tentatively identified. In addition, five different flavonoids were also tentatively identified, being the most representative group. Of these, three correspond to glycosylated derivatives (quercitrin, quercetin-glucoside, and apigenin-glucoside), and two aglycones (apigenin and kaempferol). Finally, the lignans burseran and yatein were also tentatively identified in the MeOH-Ex of B. simaruba leaves.
Table 2: Qualitative phytochemical analysis of secondary metabolites in total methanolic extracts of Bursera simaruba Sarg. Symbols (-), (+), (++), (+++) indicate the absence, a low, moderate, or high presence, respectively of each type of metabolite group in samples.
Terpenes and steroids | Phenols | Alkaloids | Saponins | Flavonoids | Tannins | Coumarins |
---|---|---|---|---|---|---|
+++ | +++ | ++ | + | + | + | + |
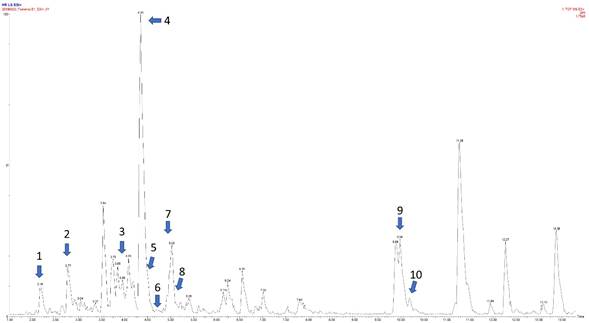
Figure 1: Representative image of the chromatogram of the MeOH-Ex of B. simaruba Sarg. leaves obtained by UHPLC-MS-ESI+. The y-axis shows the intensity expressed in % and x-axis the retention time in minutes in positive ionization. The identity of each compound marked (1-10) is shown in Table 3.
Table 3: Tentative identification of phenolic compounds in the ethanolic extract of leaves of B. simaruba Sarg. RT: Retention time in minutes. M/Z: mass/charge ratio. Mass error in ppm.
# | RT | M/Z | Compound | Formula | Adduct | Mass Error | Fragments | Chemical subgroup | |
---|---|---|---|---|---|---|---|---|---|
1 | 2.16 | 163.0394 | Caffeic acid | C9H8O4 | [M-H2O+H]+ | 1 | 145.0274 | 135.0434 | Phenolic acid |
2 | 2.77 | 377.0841 | Chlorogenic acid | C16H18O9 | [M+Na]+ | 1 | 163.0394 | 145.0275 | Phenolic acid ester |
3 | 3.98 | 449.1074 | Quercitrin | C21H20O11 | [M+H]+ | 2 | 431.0986 | 413.0863 | Flavonoid |
4 | 4.35 | 433.1129 | Apigenin-glucoside | C21H20O10 | [M+H]+ | 0 | 415.1028 | 397.0925 | Flavonoid |
5 | 4.45 | 487.0867 | Quercetin-glucoside | C21H20O12 | [M+Na]+ | 4 | 303.0511 | 271.0618 | Flavonoid |
6 | 4.71 | 437.1444 | Phlorizin | C21H24O10 | [M+H]+ | 0 | 341.0719 | 317.0878 | Dihydrochalcone |
7 | 4.93 | 287.0564 | Kaempferol | C15H10O6 | [M+H]+ | 3 | 241.0481 | 213.0557 | Flavonoid |
8 | 5.15 | 271.0608 | Apigenin | C15H10O5 | [M+H]+ | 4 | 163.0369 | 153.0176 | Flavonoid |
9 | 9.98 | 409.1622 | Burseran | C22H26O6 | [M+Na]+ | 1 | 359.1839 | 106.0839 | Lignan |
10 | 10.18 | 423.1418 | Yatein | C22H24O7 | [M+Na]+ | 3 | 383.1449 | 151.0750 | Lignan |
Antimicrobial assays
The MeOH-Ex of B. simaruba leaves did not exhibit any antibacterial activity against the tested bacterial strains of Pseudomonas cichorii and Pectobacterium carotovorum when compared with the zone of inhibition produced by the antibiotic oxytetracycline, which produced an inhibition halo of 20.3±0.2 mm to Pectobacterium carotovorum, and 20.6±0.7 mm to Pseudomonas cichorii, respectively (Fig. 2). On the other hand, the MeOH-Ex of B. simaruba leaves exhibited low inhibition of the mycelial growth in Fusarium solani (16.3%±1.88) (Fig. 3). The PDA treatment and vehicle (PDA+H2O-MeOH) did not exhibit inhibition of the mycelial growth of Fusarium solani (Fig. 3). However, thiabendazole exhibited the most significant antifungal activity (GI=100%±0) (H=10.368, DF=3, P=0.01568) (Fig. 3).
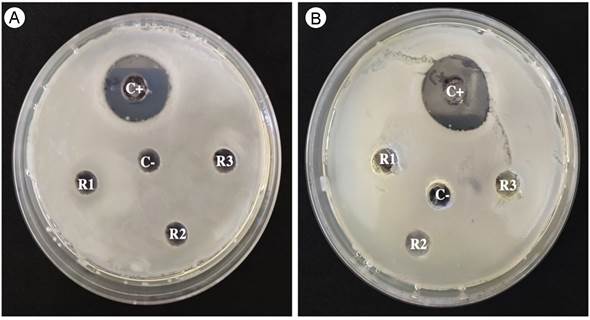
Figure 2: MeOH-Ex of B. simaruba Sarg. did not exhibit antibacterial activity against Pseudomonas cichorii (Swingle 1925) Stapp 1928 (A) and Pectobacterium carotovorum (Jones) (B). Symbology: positive control (C+), negative control (C-), extracts replicate of Bursera simaruba leaves (R1, R2, R3).

Figure 3: Mycelial growth rate (mm) of Fusarium solani (Mart.) Sacc. treated with MeOH-Ex of Bursera simaruba Sarg. leaves and controls. Bars represent mean (± SD) of mycelial growth rate of F. solani with potato dextrose agar (PDA), PDA + mix with H₂O:MeOH (85:15), MeOH-Ex of leaves of B. simaruba (2 mg/ml), and thiabendazole treatments (2 mg/ ml).
α-amylase and α-glucosidase inhibition
The MeOH-Ex of B. simaruba leaves showed significant in vitro inhibition on α-amylase (87.7±1.41% of inhibition) and α-glucosidase (75.9±17.08%) enzymes. The α-amylase inhibition by MeOH-Ex was significantly lower (H=10.532, DF=3, P<0.05) than that observed in the reference drug acarbose (99.6±0.49%), while for the α-glucosidase inhibition there were no significant differences (H=7.7234, DF=3, P=0.052) compared to acarbose (77.7±1.66%) (Fig. 4). In addition, chlorogenic acid exhibited significant inhibition on α-amylase (49.5±1.08%) and α-glucosidase (85.1±0.45%) enzymes, but only the inhibition of α-amylase was significantly different to those displayed by MeOH-Ex and acarbose (H=10.532, DF=3, P<0.05).
Discussion
Phytochemical profiling
Medicinal plants are well-known sources of metabolites that help in the treatment of symptoms of infectious illnesses as well as the prevention of non-communicable diseases (Alonso-Castro et al., 2017). The MeOH-Ex of B. simaruba leaves exhibited a complex diversity of secondary metabolites, including terpenes/steroids, phenolic acids, alkaloids, saponins, flavonoids, coumarins, and tannins. The presence of carbohydrates, triterpenes, flavonoids, long-chain fatty acids, methyl esters, phenolic acids (Bah et al., 2014), lignans (Maldini et al., 2009a), and tannins (Borges-Argáez et al., 2000) have already been reported in B. simaruba bark, which is the most studied vegetative part of Bursera spp., and few preliminary studies about the chemical composition of leaves have been conducted (Borges-Argáez et al., 2000; Carretero et al., 2008; Noge et al., 2011; Sánchez-Monroy et al., 2020). The chemical profiling on MeOH-Ex of B. simaruba leaves by high-resolution mass spectrometry allows us to tentatively identify ten phenolic compounds including caffeic acid, chlorogenic acid, phlorizin, quercitrin, quercetin-glucoside, apigenin-glucoside, apigenin, kaempferol, burseran and yatein. Previously, the presence of luteolin, protocatechuic acid, gallic acid, (-)-epicatechin, apigenin (Bah et al., 2014), proanthocyanidins (Maldini et al., 2009a), yatein, β-peltatin-O-β-D-glucopyranoside, hinokinin, bursehermin, 3,4-dimetoxyphenyl-1-O-β-D-(6-sulpho)-glucopyranoside, 3,4-dihydroxyphenylethanol-1-O-β-D-(6-sulpho)-glucopyranoside, 3,4,5-trimetoxyphenyl-1-O-β-D-(6-sulpho)-glucopyranoside, scopoletin, floroacetophenon-4-neohesperidoside, 4-acetyl-3,5-dihydroxyphenyl-β-D-glucopyranoside and picraquassioside (Maldini et al., 2009b) have been reported in B. simaruba bark. Our tentative identifications are in accordance with Sánchez-Monroy et al. (2020), who reported rutin, phlorizin, myricetin, naringin, quercetin, phloretin, naringenin, and galangin in leaves and stems of several Bursera species, although B. simaruba was not included in that investigation.
Leaves of B. simaruba have been used in Mexican traditional medicine for the treatment of fever, stomachache, spider bites, and headache (Borges-Argáez et al., 2000), as well as illnesses related to the urinary tract and as an anti-inflammatory agent (Rzedowski and Calderón de Rzedowski, 1996). In addition, leaf volatile components have exhibited anticancer activity against human lung carcinoma and human colon adenocarcinoma cell lines (Sylvestre et al., 2007). The chemical complexity of their leaves is probably associated with the wide spectrum of therapeutic activities attributed to this species (Noguera et al., 2004; Álvarez et al., 2015).
Antimicrobial activity
Regarding antimicrobial activity, extracts of the bark and leaves, resins, infusions, and tinctures of B. simaruba have exhibited antibacterial activity against both Escherichia coli (Migula 1895) Castellani & Chalmers 1919 and Staphylococcus aureus Rosenbach 1884, using extracts at concentrations of 1 mg/ml and 8 mg/ml, respectively (Yasunaka et al., 2005). However, in our study, we did not find a significant inhibitory effect of the MeOH-Ex against P. cichorii and P. carotovorum. Similar results were reported by Sánchez-Monroy et al. (2020) with extracts of leaves and stems of five Bursera spp., where extracts from leaves of Bursera schlechtendalii Engl., Bursera galeottiana Engl. and Bursera morelensis Ramírez did not inhibit the growth of the Gram-positive (Bacillus subtilis (Ehrenberg 1835) Cohn 1872 and Staphylococcus epidermidis (Winslow & Winslow 1908) Evans 1916 and negative (E. coli and Salmonella typhimurium (Loeffler 1892) Castellani and Chalmers 1919 bacteria. In contrast, the leaf extract of Bursera aptera Ramírez exhibited minimal inhibitory concentrations (MIC) values of 3.84, 3.84 and 0.002 mg/ml against S. epidermidis, E. coli, and S. typhimurium, respectively (Sánchez-Monroy et al., 2020).
On the other hand, the MeOH-Ex of B. simaruba leaves exhibited low antifungal activity against F. solani (16.3%). This inhibition is lower than that observed in the essential oil of B. morelensis, which significantly inhibited the mycelial growth (60%) of this fungus at 0.5 μl/ml (Medina-Romero et al., 2021). In addition, it has been reported that leaf extracts of Bursera species such as B. aptera, B. fagaroides Engl., B. schlechtendalii, and B. morelensis inhibited the mycelial growth of Fusarium boothii O'Donnell, T. Aoki, Kistler & Geiser (Sánchez-Monroy et al., 2020). Fusarium spp. are pathogenic fungi that directly affect human health or indirectly impact crop production for human food through the biosynthesis of mycotoxins contaminating vegetables and cereals (Pani et al., 2014; Chtioui et al., 2022). Although phenolic acids and flavonoids are not always able to inhibit the mycelial growth of some Fusarium species, they can nonetheless inhibit mycotoxin synthesis (Chtioui et al., 2022). Caffeic acid, which was tentatively identified in the MeOH-Ex of B. simaruba leaves, inhibited the biosynthesis of the mycotoxin trichothecene without affecting the mycelial growth of Fusarium culmorum (Wm.G. Sm.) Sacc. (Atanasova-Pénichon et al., 2018). In addition, caffeic acid decreased the synthesis of trichothecenes type B produced by Fusarium graminearum Schwabe (Pani et al., 2014). This suggests that, although we observed a moderate antifungal inhibition, the presence of phenolic compounds, such as caffeic acid, may play a role in the inhibition of mycotoxins from F. solani. This hypothesis should be addressed in future studies. In addition, the presence of terpenes, phenolics, and alkaloids was identified in the MeOH-Ex of B. simaruba leaves. These phytochemical groups are the main ones reported as antifungals in medicinal plants (Saxena et al., 1995) and they could be involved in the moderated antifungal effect observed.
Antidiabetic activity
The most promising bioactivity determined in the MeOH-Ex of B. simaruba leaves was the antidiabetic one. A key treatment of diabetes mellitus type 2 is the inhibition of α-amylase and α-glucosidase enzymes, which delay carbohydrate digestion and cause the consequent reduction in glucose absorption (Bischoff, 1994; Tundis et al., 2010). The high prevalence and incidence of diabetes, as well as the gastrointestinal side effects related to current treatment drugs (Bischoff, 1994; WHO, 2013, 2018), encourages us to search for new hypoglycemic agents from natural sources not yet studied. Antidiabetic properties have previously been attributed to B. simaruba bark, but there are no details about the mechanisms and metabolites involved (Castro-Juárez et al., 2014). In contrast, aqueous extracts of B. simaruba leaves collected in Yucatán, Mexico, did not inhibit α-amylase and α-glucosidase enzymes (Rodríguez-García et al., 2019), but they did so on β-glucosidase (Sánchez-Medina et al., 2001). The MeOH-Ex of B. simaruba leaves inhibited α-amylase (87.7%) and α-glucosidase enzymes (75.9%). These differences among previous studies could be attributed to different factors such as the solvent used for extraction and testing of the enzymatic inhibition reaction, or the different chemical dynamics influenced by environmental conditions.
The α-amylase inhibition by the MeOH-Ex of B. simaruba was lower than those determined in medicinal plants such as Phyllanthus amarus Schumach. & Thonn., Smilax ferox Wall. ex Kunth, and Rhus chinensis Mill., among others, but higher than that exhibited by Commiphora myrrha (T. Nees) Engl. (Table 4). Regarding α-glucosidase inhibition, the MeOH-Ex of B. simaruba exhibited lower inhibition compared to Rhizophora mangle L., but higher than S. ferox and R. chinensis, among others (Table 4). Interestingly, chlorogenic acid inhibited α-amylase enzyme at lower levels compared to the MeOH-Ex of B. simaruba, suggesting that there is more than one compound responsible for this activity. In contrast, our results suggest that chlorogenic acid could be the main compound involved in α-glucosidase inhibition.
Table 4: Comparison of in vitro α-amylase and α-glucosidase inhibition of ME from Bursera simaruba Sarg. with extracts of some medicinal plants. Data from this study are expressed in mean ± standard deviation (SD) (n=3). Symbology: methanol (MeOH), ethanol (EtOH). NE=Not evaluated.
Species | Extract | α-amylase (%) | α-glucosidase inhibition (%) | Reference |
---|---|---|---|---|
Phyllanthus amarus Schumach. & Thonn. | EtOH | 94.7±1.37 | NE | Ali et al., 2006 |
Smilax ferox Wall. ex Kunth | MeOH | 99.2±1.48 | >40 | Deepak et al., 2021 |
Rhus chinensis Mill. | MeOH | >90 | 81.1±1.36 | Deepak et al., 2021 |
Commiphora myrrha (T. Nees) Engl. | MeOH | 71.3±0.58 | 58.3±1.5 | Abdel-Hady et al., 2019 |
Bergenia ciliata (Haw.) Sternb. | MeOH | 93.5±2.1 | 30-68 | Bhandari et al., 2008 |
Rhizophora mangle L. | Aqueous | 96.7 | 92.03 | Rodríguez-García et al., 2019 |
Bursera simaruba Sarg. | MeOH | 87.7±3.57 | 75.9±17.08 | This study |
Acarbose | Drug reference | 99.6±0.49 | 77.7±1.66 | This study |
Chlorogenic acid | Standard reference | 49.5±1.08 | 85.1±0.45 | This study |
Extracts or fractions from herbal medicines have been reported as inhibitors of α-amylase and α-glucosidase enzymes, and phenolic compounds, whereas terpenes are among the main groups of metabolites reported to inhibit these enzymes through competitive and non-competitive mechanisms (Ali et al., 2006; Andrade-Cetto et al., 2008; Tundis et al., 2010). Interestingly, these families of specialized metabolites were also identified in the current study, and we propose that some of them could be involved in the inhibition of both enzymes.
Phenolic compounds such as caffeic acid and chlorogenic acid have been reported as inhibitors of α-amylase and α-glucosidase enzymes (Tundis et al., 2010). Since these phenolics were putatively identified in the MeOH-Ex of B. simaruba, we suggest that both metabolites may be participating in the antidiabetic activity observed. These phenolic compounds are active on these enzymes due to their specific pattern of hydroxyl (-OH) groups, which form hydrogen bonds with specific amino acids at the enzyme active sites (Oboh et al., 2015; Proença et al., 2022). Oboh et al. (2015) suggest that chlorogenic acid has a lesser affinity for binding to α-amylase and α-glucosidase enzymes, because the substituted quinic acid may produce steric constraints, which are unfavorable for accommodation in the active sites of the enzymes. In addition, flavonoids putatively identified in MeOH-Ex, such as kaempferol and apigenin, have been reported as inhibitors of both enzymes (Proença et al., 2022). The chemical features in flavonoids involved in the inhibition of the α-amylase enzyme are the double bond between C-2 and C-3, and the OH groups at C-5 and C-3 in rings A and C, respectively, whereas the OH groups at C-3’ and C-3 in rings B and C, respectively, cause B-ring specific entry into the catalytic active site of the α-glucosidase enzyme (Proença et al., 2022). Altogether, we determine that leaves of B. simaruba are natural sources of bioactive phenolic compounds with important antimicrobial and antidiabetic activities that should be further studied.